Motor cortex stimulation produces significant relief of symptoms in many forms of refractory chronic pain disorders.
Key points
- •
Motor cortex stimulation (MCS) provides relief of many chronic pain syndromes that have been refractory to multiple treatment modalities.
- •
MCS has been used successfully to treat pain in patients with central post-stroke pain, anesthesia dolorosa, post-herpetic neuralgia, multiple sclerosis, phantom limb pain, and spinal cord injury.
- •
Patients undergo a craniotomy for implantation, followed by a trial period of stimulation. Patients who are considered to be responders then undergo implantation of the pulse generator for chronic stimulation.
- •
Multiple techniques are available for localizing the precentral gyrus: Frameless stereotaxy with or without functional magnetic resonance imaging, somatosensory evoked potentials, and facial electromyography.
- •
Stimulation parameters vary widely, and patients often require multiple programming sessions. Some patients that experience return of their symptoms can be “recaptured” through programming changes.
Introduction
The relationship between the motor cortex and certain types of pain has been known for many years, but the nature of that relationship remains obscure. In 1954, Penfield and Japser reported relief of severe pain after resection of the primary motor cortex; previous resection of the sensory cortex had not been effective. The obvious morbidity of this approach prevented its widespread adoption. Subcortical neurostimulation for pain primarily focused on sensory pathways. However, stimulation of the primary sensory area was not found to be effective. In 1991, Tsubokawa made the serendipitous observation that chronic stimulation of the precentral gyrus below the threshold to produce a motor response was able to alleviate certain types of deafferentation pain. Subsequently, Meyerson and associates observed that the technique was particularly effective for trigeminal neuropathic pain. Since that time, a number of reports have confirmed efficacy for a number of deafferentation pain syndromes.
The mechanism of action for motor cortex stimulation (MCS) has been attributed to modulation of pathologic hyperactivity in thalamic relay nuclei that results from deafferentation, possibly because sensory neurons below a deafferenting lesion cannot exert physiologic inhibition of nociceptive neurons. Higher order neurons within the sensory pathway are known to exhibit enhanced sensitivity when afferent signals are lost. Thalamic hyperactivity has been identified as a correlate of deafferentation pain in a feline model, and MCS has been shown to normalize this to a greater degree than stimulation of the primary somatosensory cortex. However, the precise pathway involved remains elusive.
Introduction
The relationship between the motor cortex and certain types of pain has been known for many years, but the nature of that relationship remains obscure. In 1954, Penfield and Japser reported relief of severe pain after resection of the primary motor cortex; previous resection of the sensory cortex had not been effective. The obvious morbidity of this approach prevented its widespread adoption. Subcortical neurostimulation for pain primarily focused on sensory pathways. However, stimulation of the primary sensory area was not found to be effective. In 1991, Tsubokawa made the serendipitous observation that chronic stimulation of the precentral gyrus below the threshold to produce a motor response was able to alleviate certain types of deafferentation pain. Subsequently, Meyerson and associates observed that the technique was particularly effective for trigeminal neuropathic pain. Since that time, a number of reports have confirmed efficacy for a number of deafferentation pain syndromes.
The mechanism of action for motor cortex stimulation (MCS) has been attributed to modulation of pathologic hyperactivity in thalamic relay nuclei that results from deafferentation, possibly because sensory neurons below a deafferenting lesion cannot exert physiologic inhibition of nociceptive neurons. Higher order neurons within the sensory pathway are known to exhibit enhanced sensitivity when afferent signals are lost. Thalamic hyperactivity has been identified as a correlate of deafferentation pain in a feline model, and MCS has been shown to normalize this to a greater degree than stimulation of the primary somatosensory cortex. However, the precise pathway involved remains elusive.
Indications and patient selection
Most reports of successful pain control using MCS involve deafferentation owing to some form of neurologic injury. This includes post-stroke pain, trigeminal neuropathic pain/anesthesia dolorosa, post-herpetic neuralgia, multiple sclerosis, phantom limb pain, spinal cord injury, brachial plexus lesions, pelvic pain, and complex regional pain syndrome. As with any invasive treatment, patients should have failed maximal medical management before MCS is considered. Before surgery, patients should undergo a pain psychology screening.
MCS has proven to be an effective treatment option in many refractory pain syndromes. However, when counseling patients, it should be remembered that the percentage of patients who achieve good long-term pain control remains suboptimal with current techniques, and it is difficult to predict who will respond well. In addition to a standard discussion regarding the risks and benefits of the procedure, patients should also be counseled regarding the frequent need for reprogramming sessions.
Operative technique
- 1.
Positioning. Patients are placed under general anesthesia with intubation and positioned supine in a Mayfield head holder with the head turned contralateral ( Fig. 1 ). A shoulder roll is used if the patient’s habitus requires it.
Fig. 1
( A ) Surface rendering of preoperative magnetic resonance imaging showing central sulcus ( pink ), sylvian fissure ( blue ), and planned electrode ( green ) and contact ( yellow ) positions. ( B ) Postoperative lateral scout view of a computed tomography of the head. Metallic artifact from the implanted electrode shows good correlation with preoperative planning.
- 2.
Preoperative medications. Routine surgical antibiotic prophylaxis is administered before incision.
- 3.
Incision. Preoperative magnetic resonance imaging (MRI) is used with frameless stereotaxy for intraoperative navigation, allowing the surgeon to create a small incision overlying the relevant portion of the precentral gyrus.
- 4.
Craniotomy. Two burr holes are created and connected to create a small crantiotomy over the precentral gyrus.
- 5.
Localization and Implantation. The central sulcus is localized by the presence of N20-P20 phase reversal on median nerve somatosensory evoked potentials. A 2 × 4, 8-contact paddle electrode is placed in the epidural space overlying the facial or upper extremity region of the motor cortex. The electrode is then used for motor evoked potentials with electromyography to confirm motor activity. Iced saline is prepared for irrigation if a seizure is induced. The minimum thresholds for motor activity and any seizure activity are noted. After confirmation, a paddle electrode is sutured to the dura over the precentral gyrus over the motor area that corresponds to the patient’s pain distribution.
- 6.
Closure. Leads are tunneled and externalized using percutaneous extensions. The inner table of the bone flap is thinned to allow for the increased mass effect from the paddle electrode as well as an exiting site for the electrode leads. The incision is closed in layers, and a sterile dressing is applied to the externalized leads.
- 7.
Stimulation parameters and trial. Optimal pain relief is usually noted with fairly low frequency and high pulse width. Initial stimulation parameters are 50 Hz with 450 μsec pulse width. If any intraoperative after discharges are noted, maximal voltage is set at 70% of the after discharge threshold. Otherwise, maximal voltage is set at 70% of the voltage required for motor unit action potentials on electromyography. Our current practice is to perform electrode implantation at the start of the week and allow the patient to the end of the week to detect a significant difference in symptomatology. Given the high rate of programming changes and the wide variance of interpatient stimulation parameters, implantation of the IPG is performed at the end of the week if the patient has any improvement in his or her symptoms.
Localization of the primary motor cortex
Accurate localization of the primary motor cortex (Brodmann area 4) can be performed with a multitude of techniques. Anatomically, the central sulcus is easily identifiable because it does not connect to any other sulcus. The postcentral sulcus lies posteriorly and is identified by its connection with the intraparietal sulcus, which runs perpendicularly toward the occipital lobe. The precentral sulcus lies anteriorly, which is frequently bisected into the inferior precentral sulcus and superior precentral sulcus. The central sulcus can be visualized on MRI as the superficial sulcus just anterior to the prominent cingulate sulcus. The precentral sulcus can be visualized on MRI by observing its connection with the superior frontal sulcus on an high axial image and the inferior frontal sulcus on a lateral sagittal image. Landmarks can assist in estimating the somatotopic organization of the precentral gyrus. When viewed on axial images, the “omega sign” describes the appearance of a knoblike structure that maps to the hand region of the precentral gyrus. The upper extremity is usually represented posterior to the middle frontal gyrus, the face is represented in an area between the inferior frontal sulcus and the sylvian fissure, and the lower extremity is represented medially from the convexity onto the paracentral lobule. When these landmarks are combined with modern MRI techniques, frameless stereotaxy can allow the surgeon to plan the incision and craniotomy locations precisely.
There are multiple functional techniques to confirm the location of the central sulcus and precentral gyrus as well as the somatotopy of the precentral gyrus. Somatosensory evoked potentials, generated by stimulation of the median or ulnar nerves, are well known to neurosurgeons. Observation of the N20–P20 phase reversal confirms the location of the central sulcus. Motor evoked potentials allow for identification of the somatotopic organization of the precentral gyrus. The location with the lowest motor threshold for a given anatomic region is considered to be the somatotopic localization of that region. Identification of the motor threshold also assists with determination of programming parameters to maximize pain control while minimizing seizures from exceeding motor thresholds.
Functional MRI (fMRI) has been used to confirm somatotopy of the motor cortex, and it can be useful in cases of severe motor deficit or amputation where the often partially remapped motor cortex can be established by fMRI. Mogilner and Rezai described a multimodality technique that utilizes frameless MRI with fiducials, fMRI, somatosensory evoked potentials, motor evoked potentials, and magnetoencephalography. Pirotte reported a case series comparing preoperative fMRI localization with intraoperative functional localization and showed excellent concordance between the techniques. However, intraoperative functional localization with stimulation remains the gold standard. In modern neurosurgery, frameless stereotaxy is ubiquitous, easy to perform, and allows for accurate planning of a minimal incision and craniotomy.
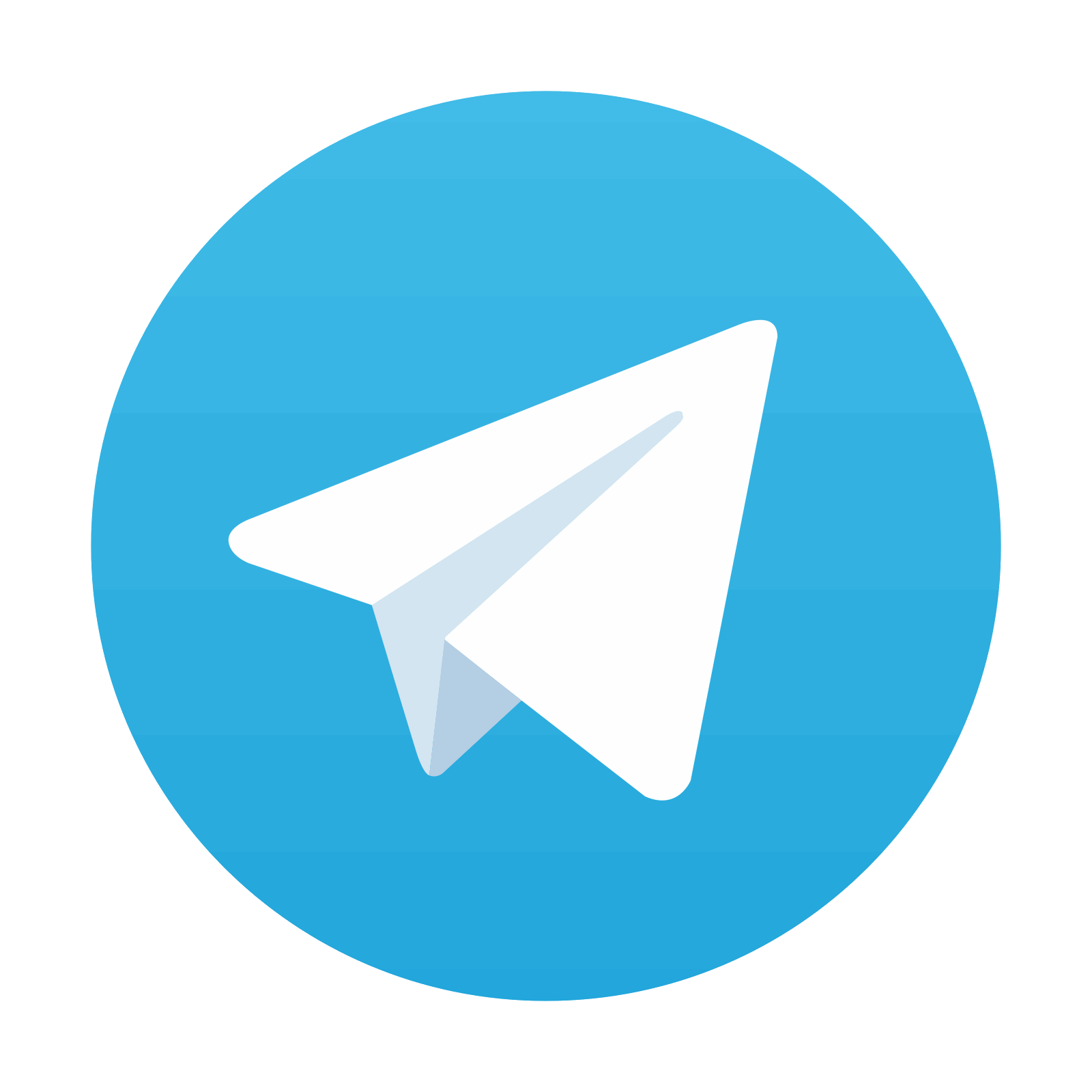
Stay updated, free articles. Join our Telegram channel

Full access? Get Clinical Tree
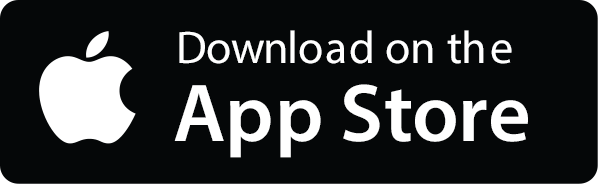
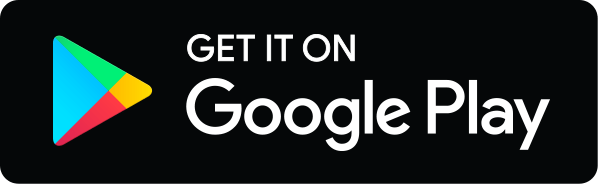