Chapter 6
Movement and Strength Training
Chapter objectives
- Provide an overview of contemporary approaches and techniques used in neurorehabilitation for facilitating or retraining voluntary movement and for improving strength.
- Distil key messages from research regarding a selection of these movement and strength training techniques.
- Delineate the upper limb characteristics of children and adults who may benefit from application of each technique.
Abbreviations
APBT | Active passive bilateral therapy |
ARAT | Action Research Arm Test |
BATRAC | Bilateral training with rhythmic auditory cueing |
Bobath/NDT | Bobath concept/neurodevelopmental therapy |
CIMT | Constraint-induced movement therapy |
CNS | Central nervous system |
COPM | Canadian Occupational Performance Measure |
CRPS | Complex regional pain syndrome |
EMG | Electromyography |
ES | Electrical stimulation |
EXCITE Trial | Extremity Constraint-Induced Therapy Evaluation trial |
FES | Functional electrical stimulation |
FMA | Fugl–Meyer Motor Function Assessment |
fMRI | Functional magnetic resonance imaging |
GAS | Goal attainment scaling |
HABIT | Hand-arm bimanual intensive training |
HSP | Hemiplegic shoulder pain |
HI CIMT | High intensity constraint-induced movement therapy |
HIPM | Hypertonicity Intervention Planning Model |
IP | Interphalangeal (joint) |
LO CIMT | Low intensity constraint-induced movement therapy |
MACS | Manual Ability Classification System |
MAL | Motor activity log |
mCIMT | Modified constraint-induced movement therapy |
MASMS | Modified Ashworth Scale of Muscle Spasticity |
MCP | Metacarpophalangeal (joint) |
NMES | Neuromuscular electrical stimulation |
PEDro | Physiotherapy Evidence Database Scale of Methodological Quality |
PNF | Proprioceptive neuromuscular facilitation |
RCTs | Randomised controlled trials |
TES | Threshold electrical stimulation |
TENS | Transcutaneous electrical nerve stimulation |
VECTORS | Very early constraint-induced movement during stroke rehabilitation study |
WMFT | Wolf Motor Function Test |
WFMT-FAS | Wolf Motor Function Test—functional activity scale |
Z&Z | Zancolli and Zancolli Hand Classification |
6.1 Changes in rehabilitation theory and practice
The strategies used in rehabilitation practice to promote movement control after brain injury are described as being “based on a cumulative history of clinical experience and scientific understanding” [1, p. 11]. That is, as changes in scientific theory and knowledge related to central nervous system (CNS) function and dysfunction take place over time, so too do ideas about ‘best practice’ rehabilitation strategies and techniques. Rehabilitation ‘pioneers’ such as Berta and Karel Bobath (Bobath concept/neurodevelopmental therapy), András Pető (conductive education), Signe Brunnstrom (Brunnstrom movement therapy), Margaret Rood (Rood’s sensorimotor therapy) and Herman Kabat, Dorothy Voss and Margaret Knott (proprioceptive neuromuscular facilitation) developed their various intervention techniques on the basis of their clinical practice and interpretation of the scientific knowledge available to them at the time. However, as knowledge has changed over time in relation to the reasons for movement dysfunction after brain injury, many of the techniques used in these early rehabilitation approaches have been questioned and researchers have sought to determine whether one approach is superior to another. Pollock et al. [2] addressed this question in a Cochrane Review of therapy approaches for improving lower limb function following stroke that included studies related to neurophysiological, motor learning and mixed approaches. In conclusion, the Review authors noted that
There is evidence that [a rehabilitation] intervention, using a mix of components from different approaches, is significantly more effective than no treatment or placebo control in the recovery of functional independence following stroke. There is insufficient evidence to conclude that any one … approach is more effective in promoting recovery of lower limb function … following stroke than any other approach. We recommend that future research should concentrate on investigating the effectiveness of clearly described individual techniques and task-specific treatments, regardless of their historical or philosophical origin [2, p. 2].
Thus, in preference to continued focus on ‘approaches’, these authors and others [3–6] have recommended research that aims to determine the efficacy of clearly described rehabilitation techniques used to support individual goal achievement. In addition to suggesting a research focus on such techniques, it is presumed that the authors would similarly recommend the use of such techniques in neurological rehabilitation practice. With this in mind, the remainder of this chapter provides an overview of the three main approaches to neurological rehabilitation (the biomechanical, neurophysiological and restorative neurorehabilitation approaches) as a background to the presentation of a selection of contemporary intervention techniques.
6.2 Common rehabilitation approaches: Assumptions, principles and strategies
6.2.1 Biomechanical approach
The biomechanical approach to rehabilitation focuses on impairment-level (or capacity-level) factors that limit participation in valued activities [4]. It is described as applying “the principles of physics to human movement and posture with respect to the forces of gravity” [7, p. 165]. In this approach, movement is conceptualised in terms of physical forces (gravity, pressure), levers (bones and joints) and torques (muscles), and is concerned with how each of these factors affects the person’s equilibrium (balance) and movement. Intervention is focused on improving muscle strength, physical endurance and the range of motion, stability and mobility of joints [8]. In rehabilitation for people with physical disability, the prevention of deformity, or reduction of the effects of deformity, is achieved through positioning, stretching, the application of physical modalities (such as splints or adaptive seating) and the use of rehabilitation equipment and technologies (such as functional electrical stimulation, robotic devices or computers) [9].
In therapy practice the ‘rehabilitative’ or ‘adaptation’ model can be considered to fit within a biomechanical approach [10]. This model aims to promote independence despite residual impairment [11]. It has been described as using biomechanical principles, environmental adaptation and provision of alternate strategies for task completion to compensate for limitations in, or absence of, voluntary movement control [11, 12]. Strengths of the biomechanical approach include its usefulness for identifying problems with motor and sensory capacity, its promotion and measurment of improvements in those capacities, and its focus on compensation and remediation. However, it is proposed that this approach is more effective when integrated with approaches that incorporate broader, functionally-focused models [4, 13].
Examples of intervention aims and principles, assessment tools, and intervention strategies typically used within the biomechanical approach to the management of upper limb hypertonicity are presented in Table 6.1.
Table 6.1 Biomechanical approach: intervention principles and strategies [4, 17–20].
Intervention Aims |
|
Principles | Improve impairment-level factors by:
|
Assessment Focus | Impairment-level factors such as:
|
Assessment Tools (examples) |
|
Intervention Strategies and Techniques (examples) |
|
6.2.2 Neurophysiological approaches
Neurophysiological rehabilitation approaches [21] to intervention for movement problems after brain injury have been described as including sensorimotor therapy [22], proprioceptive neuromuscular facilitation (PNF) [23], movement therapy [24], and Bobath/neurodevelopmental therapy (Bobath/NDT) [25, 26]. Early neurophysiological approaches were based on reflex-hierarchical theories of motor development and control, where movement organisation was thought to be reliant on the integration of the CNS and appropriate sensory input. Thus, treatment principles focused on influencing the CNS itself through stimulation of sensory receptors [21]. The aim of such treatment was to normalise increased muscle tone and facilitate the integration of lower-level reflexes as the basis for voluntary movement. Treatment techniques were designed to facilitate and inhibit abnormal movement patterns [21]. Rood [22] summarised this approach as follows:
It seemed to me that if it were possible to apply the proper sensory stimuli to the appropriate receptor as it is utilized in normal sequential development, it might be possible to elicit the motor responses reflexly, and by following neurophysiological principles, establish proper engrams. The movement which results in response to a summation of reflexes is “boosted” up to the higher centres for final reception at the sensory cortical level [22, p. 445].
Of the various neurophysiological approaches, Bobath/NDT is the most widely used intervention approach for children and adults with brain injury in Australia [27, 28], Sweden [29] and the United Kingdom [30–32]. It is also, therefore, the approach that has most often been compared with more recently developed practice approaches. For example, in a systematic review [33] of 16 ‘high quality’ randomised controlled trials (RCTs), of which 9 were concerned with the upper limb, three studies compared Bobath/NDT with the Motor Relearning Programme/Movement Science, and six compared impairment-focused therapy, robotic therapy, independent practice of motor tasks, forced use/constraint-induced movement therapy and repetitive bilateral arm training with Bobath/NDT. Kollen et al. [33] concluded that
no evidence is available for the superiority of any approach … on sensorimotor control of the upper and lower limb, dexterity, mobility, ADL [Activities of Daily Living], HRQOL [Health-related Quality of Life], and cost-effectiveness [33, p. 93].
Reflecting changes in theory and practice, Bobath/NDT is now described as having integrated contemporary neurosciences knowledge into its approach, including a systems theory of motor control, and current concepts relevant to motor learning and neuromuscular plasticity, specifically the adaptive changes that occur in neural and non-neural tissues secondary to brain injury [6, 33, 34]. Contemporary Bobath/NDT practice is identified as a
conceptual, problem-solving framework that [clinicians] can use to visualize and problem-solve [their] way through the complex, multistage process of recovery from stroke. NDT/Bobath provides a holistic, humanistic framework that allows clinicians to see when and how to incorporate treatment techniques such as FES [Functional Electrical Stimulation], CIMT [Constraint-Induced Movement Therapy], and so on [6, p. 388].
Examples of intervention aims and principles, assessment tools, and intervention strategies typically used within the Bobath/NDT approach to the management of the upper limb after brain injury are presented in Table 6.2. Bobath/NDT associations exist in many countries (for example, Australia, the United Kingdom, the United States of America, Canada, Europe, India and the Philippines), and are easily accessible via the worldwide web should further information be required.
Table 6.2 Bobath/neurodevelopmental therapy: intervention principles and strategies [34–38].
Intervention Aims |
|
Principles | Improvement of motor control by:
|
Assessment Focus |
|
Assessment Tools (examples) |
|
Intervention Strategies and Techniques (examples) |
|
The other neurophysiological approaches mentioned earlier in this section (Rood’s sensorimotor therapy, Kabat and Knott’s PNF and Brunnstrom’s movement therapy) are not addressed in detail here since they are less frequently used in contemporary upper limb rehabilitation after brain injury. Instead, a brief description of the intervention strategies and modalities used in each approach is presented in Table 6.3. In general, the aim of these approaches is to control muscle activation and movement patterns through sensory stimulation which is theorised to influence higher cortical areas of the CNS [1].
Table 6.3 Sensorimotor therapy, proprioceptive neuromuscular facilitation and movement therapy: intervention principles and strategies.
Neurophysiological Intervention Approach | Intervention Strategies and Modalities |
Rood’s Sensorimotor Therapy [22, 39] | Facilitatory proprioceptive techniques:
Inhibitory proprioceptive techniques:
Facilitatory exteroceptive techniques:
Inhibitory exteroceptive techniques:
|
Proprioceptive Neuromuscular Facilitation [23, 40, 41] | Diagonal/spiral patterns of movement are used to strengthen muscles, and improve sensory awareness and motor function Techniques used to facilitate diagonal patterns include:
|
Brunnstrom’s Movement Therapy [24, 42, 43] | Movement recovery is promoted by using synergy patterns to elicit movement in the sequence it usually appears post-stroke Seven stages of recoverya are described as:
Movement recovery is facilitated through these stages by:
|
a The seven stages of recovery are reflected in the Fugl–Meyer Motor Function Assessment (FMA) [44], which is sometimes called the Brunnstrom–Fugl–Meyer Motor Function Assessment since it is based on Brunnstrom’s stages of recovery [42]. The FMA is reported to be more discriminative than the Motor Assessment Scale [45], and to correlate strongly with the Arm Function Test [46].
6.2.3 Restorative neurorehabilitation approaches
The restorative neurorehabilitation approaches aim to:
take the insights about neural plasticity derived from animal models and [people without brain injury] and apply them therapeutically in … patients after acute or chronic injury to the CNS. The long-term goal is to restore, where possible, the functions previously performed by permanently damaged tissue [and, if that is not possible, to promote] behavioral adaptation to loss of function [through] contextual changes and psychological adjustment [47, p. 2].
Advances in neurosciences research have indicated that the brain and CNS have the ability to reorganise after injury due to the property of plasticity [48, 49]. ‘Plasticity’, as used in this context, refers to the capacity of the CNS to adapt to the functional demands placed upon it through both anatomical and physiological changes [50, 51]. Research indicates that, for children and adults with brain injury, restorative rehabilitation strategies, such as environmental enrichment, repetitive use, task-specific intensive practice, and invasive (implantable electrodes) and non-invasive (electromagnetic) cortical stimulation, are effective for improving upper limb function through cortical reorganisation [52]. However, while neural plasticity is now recognised as possible, the underlying mechanisms remain unclear, and further research is required to translate animal studies to clinical practice [47, 53]. Tables 6.4 and 6.5 provide a summary of intervention principles and techniques and define strategies for optimising motor learning within this approach.
Table 6.4 Restorative neurorehabilitation: intervention principles and strategies [48, 50, 74–76].
Intervention Aims |
|
Principles |
|
Assessment Focus |
|
Assessment Tools (examples) |
|
Intervention Strategies and Techniques (examples) |
|
Table 6.5 Motor learning strategies within restorative neurorehabilitation [21, 50, 74, 77, 78].
Strategy | Description |
Repetition |
|
Practice Conditions |
|
Task Orientation |
|
Ecological Validity |
|
Shaping |
|
Feedback and Knowledge of Results |
|
Motivation |
|
6.3 A selection of contemporary techniques: Indications and considerations
The use of specific research evidence-based techniques when treating upper limb hypertonicity is supported in the rehabilitation literature in preference to the use of general ‘treatment approaches’ [12, 79, 80]. A systematic review of the best available evidence for treatment techniques for children with cerebral palsy found support for 15 discrete interventions that reflected current neuroscience knowledge [79]. Effective interventions relevant to the management of upper limb spasticity/hypertonicity included Botulinum toxin-A (BoNT-A) to reduce spasticity, serial casting to reduce contracture, and CIMT, bimanual training, goal-directed/functional training, (occupational) therapy following BoNT-A, and home programmes for improving motor activity performance and/or self-care.
In relation to adults with stroke, Pomeroy et al. [12] have made recommendations regarding ‘best evidence’ upper limb rehabilitation techniques on the basis of available RCTs and consensus guidelines. In making their recommendations, the authors highlighted the importance of providing intervention at sufficient dose (number of repetitions) to make an impact within a therapeutic environment that enhances motor learning. Recommended interventions include mental practice, visual imagery, mirror therapy, cortical stimulation, robotic devices, EMG biofeedback, functional electrical stimulation (FES), CIMT and bilateral training. However, the authors also noted that there is more evidence for improvement in arm function than hand function in adults post-stroke and that, in particular, there is little evidence for improvement in hand coordination and dexterity.
It is beyond the scope of this book to provide extensive information about the many interventions that are available for use in upper limb rehabilitation. Instead, a selection of contemporary, evidence-based techniques is described in more detail in the remainder of the chapter. These include CIMT (Section 6.3.1), bimanual training (Section 6.3.2), motor imagery and mental practice (Section 6.3.3), mirror therapy (Section 6.3.4) and electrical stimulation (Section 6.3.5). Casting and Botulinum neurotoxin-A are addressed in Chapters 8 and 9, respectively.
6.3.1 Constraint-induced movement therapy
6.3.1.1 Description
Constraint-induced movement therapy was originally designed to address ‘learned non-use’ of an affected (hemiplegic) upper limb after stroke. Learned non-use is proposed to arise following injury to areas of the cortex involved in movement control, particularly the primary motor and the supplementary motor areas (see Section 2.4.1.4). The functions of the supplementary motor area are not fully understood, but include the planning of movements that are internally-generated (in comparison to movements that occur in response to external circumstances or input), sequence planning, and coordination of both sides of the body (for example, during bimanual tasks). Impairments in somatosensory awareness and motor control are thought to set up a cycle of decreased movement capacity, unsuccessful attempts to move, reduced motivation to move, limb immobilisation and, finally, a reduction in representation of the limb in the cortex [81, 82].
CIMT (also known as constraint-induced therapy or “Taub therapy”) is described as a behavioural, problem-solving approach to rehabilitation of the upper limb following stroke [83]. It consists of three main elements: (i) constraint of the person’s non-affected limb, (ii) repetitive, task practice and (iii) adaptive task grading or ‘shaping’ (see Box 6.1) [82, 84]. ‘Forced use’ is differentiated from CIMT by including only restraint of the less-affected limb during home-based activities; intensive training and coaching are not included [85]. Modified-constraint-induced movement therapy (mCIMT) includes restraint and supervised training, but training times/intensities, restraint time and supervision ratios are modified in comparison to CIMT [86].
6.3.1.2 Mechanisms of effect
Reduced motor cortex excitability and a decrease in the cortical area representing the affected upper limb have been found after stroke [52]. However, the results of CMIT studies have shown increased metabolic and synaptic activity, and cortical reorganisation of motor maps in the primary motor cortex, premotor cortex, supplementary motor area and cerebellum, as well as other areas linked to movement control in both the ipsilesional (same hemisphere as the injury) and contralesional (hemisphere opposite to the injury) brain areas [51, 82]. In relation to movement, research has indicated that movement which promotes selective motor control, increased joint isolation and reduced use of synergies (see Section 2.4.1.2) is most likely to lead to adaptive plastic changes [82].
Hemiplegic cerebral palsy is related to three typical lesions: cerebral malformation, periventricular lesions and cortical/subcortical (including grey matter) lesions [69, 89]. Variations in the degree of motor impairment and hand function have been linked to these different types of lesions. Children with cerebral malformations have demonstrated mild limitations in hand function compared to those with periventricular lesions, while children with cortical/subcortical lesions have shown the most limited hand function, especially if lesions are apparent in the thalamus and/or basal ganglia [69, 89–91]. Hand function in children with cortical/subcortical lesions also appears to be more affected when the lesion is extensive, and when the hemiplegic hand is controlled by (uncrossed) ipsilateral corticospinal projections alone [92, 93]. To date, studies of CIMT indicate improvements in hand function regardless of type of lesion. However, weak trends are evident favouring greater improvement for children with cortical/subcortical lesions [69], and for those in whom both hemispheres contribute to control of the affected limb due to preservation of corticospinal projections from the contralateral hemisphere, a feature which typically resolves in older children [93].
Animal studies have also shown that cortical changes were only apparent after successful completion of complex motor tasks, rather than in response to the performance of easy, unskilled movements [94]. This finding has implications for the grading of tasks (or shaping) during CIMT training, ensuring that they are sufficiently challenging whilst allowing successful completion. Further, cortical changes in animals have also been found to correspond to the consolidation of motor skill that occurs during the late stages of training rather than in response to the initial acquisition of motor skills [95]. Similarly, in children with hemiplegic cerebral palsy, Aarts et al. [96] have suggested that improved quality of hand function and increased use of the affected upper limb after mCIMT are based on better utilisation of existing motor capacity rather than on improvements in underlying muscle strength or motor selectivity. On the basis of these findings, the authors proposed that the aspect of learned non-use that may be most responsive to mCIMT could be movement refinement (or quality of movement) rather than increased quantity (frequency or automaticity of use).
6.3.1.3 Research evidence
CIMT for adults post-stroke was introduced as the key element of the Extremity Constraint-Induced Therapy Evaluation (EXCITE) trial, a prospective, randomised, seven-site, single-blind clinical trial conducted between January 2001 and January 2003 [88]. Participants were stratified according to their initial level of motor ability and then randomised into immediate or delayed intervention groups. The trial methodology is described in Box 6.2 and outcomes are presented in Table 6.6. The EXCITE methodology and outcomes are presented in some detail since the protocols and inclusion/exclusion criteria of other CIMT/mCIMT studies are largely based on, or compared to, those in the original trial.
Table 6.6 EXCITE trial outcomes.
Study | Outcomes |
Wolf, Winstein, Miller et al. (2006) |
|
Wolf, Winstein, Miller et al. (2008) |
|
Park, Wolf, Blanton et al. (2008) |
|
Schweighofer, Han, Wolf et al. (2009) |
|
Lang, Thompson & Wolf (2013) |
|
In addition to many clinical trials, the use of both CIMT and mCIMT for adults post-stroke has been the subject of numerous RCTs and systematic reviews, with or without meta-analysis. Two recent systematic reviews (with meta-analyses) are described here. Nijland et al. [102] considered the effects of mCIMT for people in the acute or sub-acute phase after stroke. The review hypothesis was that mCIMT would have a positive effect on upper limb function, and that a lower dose of mCIMT would be more beneficial than the complete CIMT format during acute or sub-acute post-stroke recovery (defined as the first 10 weeks after stroke onset). This hypothesis was based on the findings of a number of animal studies [103–105] and those of the ‘very early constraint-induced movement during stroke rehabilitation (VECTORS) study [106]. The animal studies found that exclusive use of the affected forelimb within the first 7–15 days post-stroke led to an increase in the extent of the original lesion, while the VECTORS study indicated that CIMT was as effective, but not better than, an equal dose of ‘usual occupational therapy’. Further, higher intensity CIMT led to less motor improvement at 90 days, suggesting that higher doses of motor training early after stroke were not necessarily of more benefit. Importantly, though, no evidence of activity-dependent enlargement of brain lesions was found on magnetic resonance imaging for participants in the higher intensity group, as was found in the animal studies [106].
Five RCTs, described as being of ‘good’ methodological quality (PEDro scale), were included in the systematic review of mCIMT [102]. Two studies compared high intensity (HI) CIMT with usual care (see Box 6.3 for definitions) [107, 108], two compared low intensity (LO) CIMT with usual care [109, 110], and one study compared HI CIMT with LO CIMT [106]. Recruitment among the five studies ranged from 2–19 days post-stroke. Results showed statistically significant differences in favour of CIMT when compared with the usual therapy for motor capacity and activity. However, when the different intensities of CIMT were compared, statistically significant differences in favour of LO CIMT were found for all measures. The authors noted that
the current review suggests that LO CIMT may be more beneficial during the acute or sub-acute
phase than HI CIMT. However, because of the relatively small number of heterogeneous studies, caution is required in the interpretation of the results [102, p. 432].
The second systematic review discussed here compared CIMT with other dose-matched interventions for post-stroke upper limb movement dysfunction [88]. Twenty-two studies, described as ‘good’ quality (PEDro scale) were included in the review. The majority of the included studies used a training dose of between 20 and 30 hours (compared to the ‘traditional’ CIMT dose of 60 training hours). Outcomes were compared in relation to motor capacity (using the FMA, 15 studies), upper limb activity outcomes (using the ARAT, 14 studies), and comprehensive function (on the Functional Independence Measure, 6 studies). Statistically significant effects in favour of CIMT were found across all these measures. In conclusion, the review authors stated that:
Although this finding largely replicates those of previous systematic reviews, the present review permits a stronger conclusion regarding the efficacy of CIMT for UL deficits in adult stroke survivors … Because we were able to identify sufficient trials that used dose-matched control groups, we can draw a more robust conclusion that the efficacy of CIMT does not seem to be a function of increased time spent in therapy [88, p. 408].
CIMT and mCIMT for children with cerebral palsy have been investigated in both clinical trials and systematic reviews, and studies comparing constraint with bimanual training have also been common (see Section 6.3.2.3). Two reviews are described here [111, 112]. Charles and Gordon [111] included 15 studies in their review of forced use and constraint-induced therapy (CIT), although these were described as providing evidence that was “tentative at best” [111, p. 249]. Four studies were classified as providing Level 2 evidence and the remainder were classified between Levels 3 and 5 (using the American Academy of Cerebral Palsy and Developmental Medicine classification). The authors noted that variability in the type and duration of restraint, length of intervention, practice intensity, and assessment measures made it difficult to draw conclusions regarding efficacy. Restraint included casting, slings and mitts, and intervention duration ranged between two and three weeks. Participants in all the studies had hemiplegia, however aetiology differed, and included cerebral palsy, acquired brain injury and stroke. Ages ranged from 12 months to 18 years. The authors concluded that, since all the studies reported positive outcomes, forced use and CIT showed potential for improving hand function in children with hemiplegia, but that further research was necessary.
Hoare et al. [112] undertook a Cochrane Review that evaluated the effectiveness of CIMT, mCIMT and forced use for children with cerebral palsy (hemiplegia). Three studies were included, a RCT of CIMT [113, 114], a controlled clinical trial of mCIMT [115], and another of forced use [116]. The study that investigated CIMT used a bivalved fiberglass cast to restrain the less-affected arm, worn during therapy for six hours/day over 21 consecutive days [113, 114]. The clinical trial of mCIMT used a fabric glove over a volar thermoplastic splint, worn on the unaffected hand for two hours/day, 7 days/week over two months in the child’s usual environments (home/preschool). The intervention was compared with traditional, weekly occupational therapy [115]. The forced use study provided restraint through a short arm cast from below the elbow to the fingertips of the unaffected upper limb. Occupational therapy was provided twice weekly in 30-minute sessions over six weeks for the treatment and control groups [116].
The systematic review analyses indicated a positive treatment effect favouring CIMT, a significant treatment effect for mCIMT at two and six months, and a significant treatment effect at six weeks for forced use [112]. Only one study included comprehensive information about individual upper limb characteristics [69]. Older children, and those with very poor function, were found to make larger improvements in response to training compared with younger children or those with mild functional impairments. The reasons for these outcomes were unclear. The authors recommended that the use of CIMT, mCIMT and forced use for children with cerebral palsy should remain within clinical trials until further evidence becomes available.
More recently, Dong et al. [117] published a systematic review of studies that compared CIMT and Bimanual Intensive Training in children with unilateral cerebral palsy, ranging between two and 16 years of age. Seven studies were identified, described as having ‘fair’ quality (PEDro scale). Four studies used an intervention regime of 6 hours/day over 10 days in an intensive day camp (total 60 hours); two studies included 6 hours/day over 15 days in a camp (total 90 hours); and the final study used 3 clinic-based hours/day for 3 days/week, combined with 3 home-based hours/day for 4 days/week over 10 weeks (total 210 hours). Some studies used a theme-based program such as circus training and some did not. All the studies reported significant improvement in function for the affected upper limb. One study noted that 26 weeks after 60 hours of CIMT, predictors of a ‘best response’ were CIMT and a larger initial change in percentage scores on the Melbourne Assessment of Unilateral Upper Limb Function between baseline and three weeks. In addition, at 26 weeks, the odds of achieving a favourable outcome for unimanual capacity were 21 times greater for CIMT than bimanual training [118]. The systematic review authors concluded that a combination of the two interventions may be most appropriate for promoting both unimanual capacity (through CIMT) and bilateral performance (through Bimanual Intensive Training) [117]. Current research is underway to determine the optimal dosage of such hybrid models of upper limb training [118].
6.3.1.4 Indications
The EXCITE CIMT trial included adults post-stroke with mild (20° active wrist extension, 10° active finger extension) and moderate (10° active wrist extension, 10° active extension in two fingers) upper limb impairment (see Box 6.2) [97]. Improvements were noted at impairment and activity levels in both of these participant groups, whether in the acute/sub-acute or chronic phase of recovery, although improvements were more limited for people in the moderate/chronic group. The trial outcomes also suggested that older adults who demonstrated reduced speed of upper limb movement, and/or reduced proprioception, and/or a WFMT-FAS score below the threshold of 3.44/6 were less likely to maintain functional improvements in the year following CIMT (see Table 6.6) [100]. The upper limb characteristics described in the EXCITE trial as indicative of ‘mild impairment’ appear to correspond with characteristics within the Hypertonicity Intervention Planning Model (HIPM) 1 group (see Table 3.2), while characteristics demonstrated by those with ‘moderate impairment’ correspond with the HIPM 2a group.
The 22 studies of CIMT included in the systematic review by Stevenson et al. [88] used a range of assessments to define participant characteristics for inclusion in CIMT. The majority applied at least two of the assessments described in Table 6.6. Thus, it appears that, similar to the EXCITE trial, the indications for inclusion in a range of CIMT studies were mild or moderate upper limb impairment after stroke. Again, these characteristics appear to reflect attributes within the HIPM groups 1 and 2a.
Indications for mCIMT for adults with stroke, as investigated in the five studies in the systematic review by Nijland et al. [102] are similar to those described in Table 6.7:
- At least 10° of active movement in the thumb and two or more fingers (n = 3, 60%)
- NIHSS, Motor Arm score 1–3 (n = 4, 80%)
- Motor Assessment Scale, Upper-Arm Function ≥ 3/6 (n = 2, 40%)
Table 6.7 CIMT research: characteristics of adults with stroke [88].
Assessment | Characteristics/ Assessment Score | Frequency of use in studiesa (N = 22, %) |
Active wrist/finger extension | 20° wrist extension 10° finger extension | n = 12, 54.5 |
Modified Ashworth Scale | Grade < 2/6 indicating mild or moderate hypertonicity (see Table 4.2) | n = 12, 54.5 |
Brunnstrom Arm Recovery Stage | Stage > 3/7 indicating reducing spasticity and increasing capacity for joint isolation (see Table 6.3) | n = 7, 31.8 |
Motor Assessment Scale [70] | Upper-Arm Function ≥ 3/6, indicating increasing strength and ability to control the shoulder and elbow against gravity | n = 2, |
National Institutes of Health Stroke Scale (NIHSS) [119] | Motor Arm score 1–3, indicating capacity to generate muscle contraction and increasing ability to control upper limb against gravity | n = 3, 13.6 |
a N is the number of studies and n is the number of times an assessment was used.
Indications for participation in CIMT or mCIMT are more difficult to ascertain for children and adults with cerebral palsy, with many studies simply stating that the inclusion requirement was ‘hemiplegic cerebral palsy’, and that ‘children with all degrees of impaired hand function were included’ [69, 121, 122]. In addition, the majority of studies with children have used constraint as a control group while investigating the effectiveness of bimanual training (see Section 6.3.2), making it difficult to identify clear criteria for the upper limb in relation to CIMT. Consideration of the studies included in the systematic review by Dong et al. [117], plus some more recent RCTs, provides some guidelines regarding upper limb characteristics suitable for participation in CIMT (see Table 6.8). One RCT reported that ‘best responders’ to intensive training were children who were older, had poorer hand function at baseline, and had left hemiplegia [119]. The authors noted that this finding confirmed previous reports [69] that children with poorer function may respond better to intensive upper limb training, possibly because they have greater scope to improve than those with milder impairment. Interestingly, these results for children with cerebral palsy are different to those found in the EXCITE trial for adults with stroke. Whereas outcomes for the adults were similar between low and high functioning groups during the first year after CIMT, most improvements over the second year were in the higher functioning group.
Table 6.8 CIMT research: characteristics of children with cerebral palsy.
Assessment | Characteristics/ Assessment Score | Frequency of use in studies (N = 6, %) |
Diagnosis/Age |
| n = 6, 100 |
Motor Impairment [121] |
| n = 1, 16.7 |
Active wrist/finger extension [123, 124] |
| n = 2, 33.3 |
Jebsen–Taylor Test of Hand Function [123, 124] |
| n = 2, 33.3 |
Modified Ashworth Scale of Muscle Spasticity (MASMS) [119–125] |
| n = 2, 33.3 |
Manual Ability Classification System (MACS) [119–125] | MACS Levels I–II, where:
| n = 2, 33.3 |
Manual Ability Classification System (MACS) [96] | MACS Levels I–III, where:
| n = 1, 16.7 |
In summary, the upper limb characteristics of children with cerebral palsy described as appropriate for exposure to CIMT or mCIMT correspond to the HIPM groups 1 and 2a. Children whose characteristics fit within HIPM 2b are also included in several studies [96, 121]. Children with more severe impairments (MACS Level III) [96] were described as ‘best responders’ in terms of reduction in developmental disregard (learned non-use).
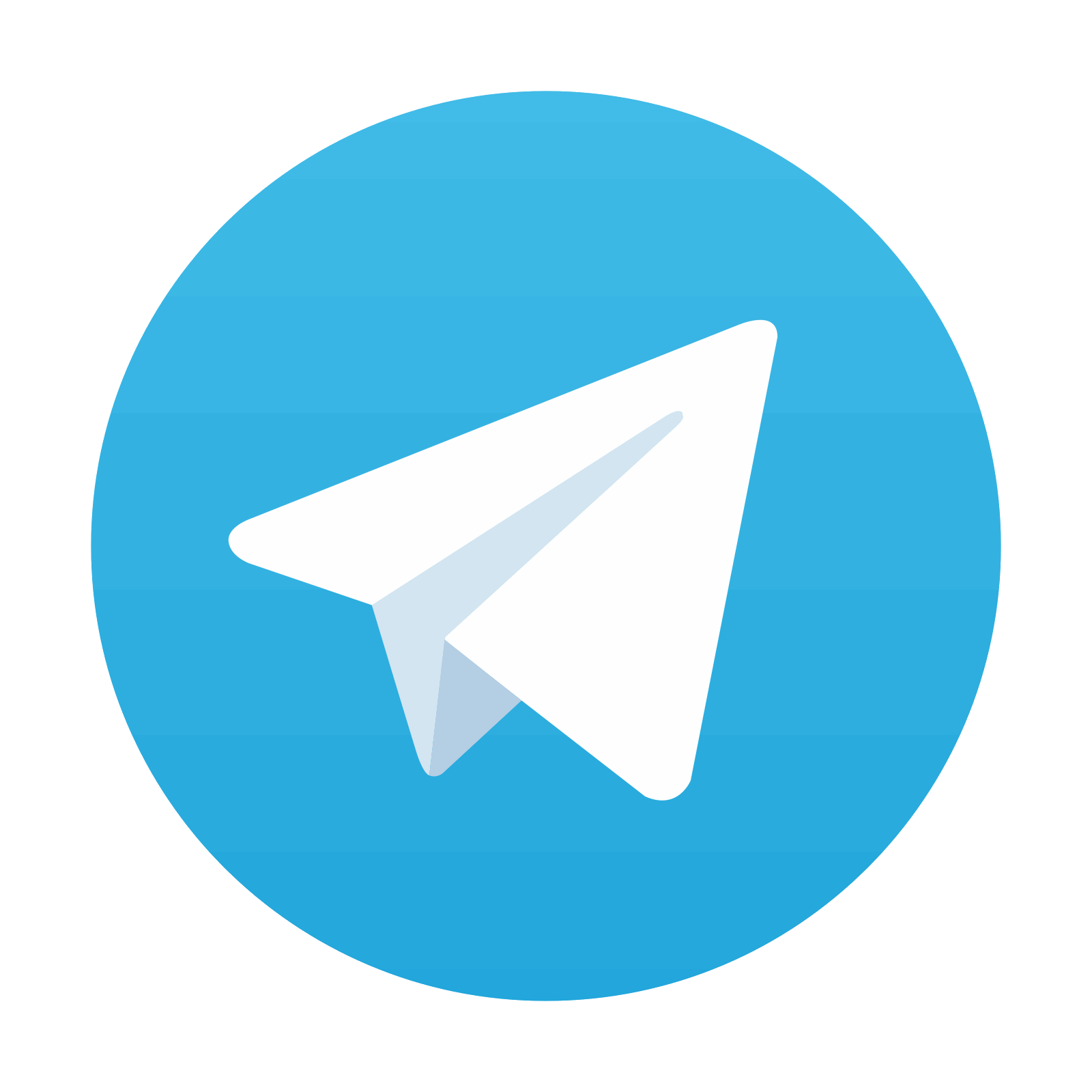