Introduction
Magnetic seizure therapy (MST), as the name implies, is a treatment that involves the induction of therapeutic seizures generated through magnetic fields. The impetus for developing this treatment was the goal of achieving comparable therapeutic effects to ECT, while minimizing its cognitive side effect profile. ECT is known to be a highly effective treatment for treatment-resistant depression (TRD) ( ), and this is reviewed in detail in this book in Chapter 19 . As highlighted in this chapter, ECT is the most effective treatment for depression with a remission rate of up to 75% in TRD ( ). Although it has impressive treatment effects, a significant proportion of patients do not tolerate the treatment due to troublesome side effects that include postictal confusion ( ), anterograde and retrograde memory loss ( ), and other cognitive sequelae ( ). In fact, difficulties forming new memories can last up to several months after completion of a treatment course of ECT ( ; ; ), and although rare, the loss of past memories, formed prior to a treatment course, remains as a serious adverse effect ( ).
ECT has been optimized and fine-tuned to improve its tolerability. For example, the development of right unilateral ultrabrief pulse width ECT demonstrated similar efficacy in treating depression, with fewer cognitive side effects in comparison to standard ECT techniques ( ; ). Evidence suggests that higher electrical field charge can predict cognitive outcomes for ECT, and that RUL ECT tends to have far fewer adverse cognitive effects than bilateral ECT ( ). This advancement in ECT delivery was made possible with knowledge of the neurophysiology of neuronal depolarization ( ; ). This supports the notion that rational approaches to improvements in convulsive therapy are warranted and may lead to additional advances in the future. Even with these advances in ECT practice, cognitive side effects and negative stigma still greatly limit its use and accessibility, as less than 1% of patients with TRD end up receiving this treatment ( ). Therefore, the development of MST brings the promise of a fresh approach with the hope for little to no cognitive side effects, presumably as a result of more focal stimulation and an altogether different method of stimulus ( ). Based on the concept of electrical shunting from impedance of the skull and cerebrospinal fluid, electrical fields produced through electromagnetic induction could in theory greatly improve targeting of brain structures and eliminate cognitive side effects. The hope for MST is that it will achieve similar efficacy to ECT, but with a superior cognitive side effect profile to reduce the morbidity for patients with severe psychiatric and treatment-resistant illness.
In this chapter, we first review the origins of MST and then explore recent advances in the use of MST in TRD and related conditions. We then focus on research exploring potential mechanisms of action of MST. This chapter includes a review of the safety, efficacy, and adverse effects of MST.
History and development
The initial development of MST emerged out of work in the mid-1980s on the magnetic stimulation of nerve and muscle tissue in healthy individuals and patients with neurological conditions. Barker was the first to report on subconvulsive magnetic stimulation in the human brain, and described its relative ease of use, limited adverse effects, and the great clinical potential of this technology, in comparison to pure electric stimulation ( ). Barker found significant ease in dealing with the high resistance structures of the skull in order to produce muscle contractions in the contralateral limb, which was previously seen with electric impulses ( ). This early work was with magnetic stimulators capable of frequencies only as high as 0.33 Hz, and so development of magnetic stimulators capable of much higher frequencies of stimulation would later be essential for the advancement of this field in psychiatry, and medicine in general ( ).
Soon after Barker’s work researchers using magnetic stimulators capable of repetitive transcranial magnetic stimulation (rTMS) showed that, while overall safe, rTMS had the capability to induce generalized tonic-clonic seizures in healthy individuals without epilepsy with frequencies as low as 10 Hz stimulation ( ). While unwanted in this particular study, this finding would later be the basis for purposefully induced therapeutic seizures with magnetic stimulation. In 1994, Sackeim commented on the potential of this technology and described how MST could drastically increase the specificity of brain areas targeted in convulsive type treatment, which could theoretically reduce or eliminate side effects seen with ECT treatment; brought about by discharge of deeper brain structures ( ). This then spurred the first investigations of magnetic seizure therapy in mammals and primates, and later in human subjects.
Lisanby et al. performed the seminal translational research and early troubleshooting that brought MST from the bench to the bedside, with the development of optimal treatment parameters and targeting of specific brain regions ( ). The first MST device could produce frequencies of 25 Hz, and would not induce seizures reliably in macaques ( ). Yet, they were able to demonstrate consistent seizures with a device capable of a treatment frequency of 40 Hz with a 6.3 s train duration and machine output of 90% intensity. With this device though the authors describe struggles with overheating coils, which would be a major limitation in later clinical trials ( ). A subsequent report demonstrated the first successful use of MST in a human, a case report of a young female patient diagnosed with TRD who received four MST treatments during a course of ECT ( ). In this case, 40 Hz stimulation was administered over the right prefrontal cortex for 4 s; the patient did experience modest clinical improvement ( ).
MST devices capable of 50 Hz frequency for 8 s train durations were developed next ( ), and in 2003, Kosel et al. published a case report on the first full MST treatment course for a patient with TRD with these treatment parameters ( ). This patient was in remission after 12 treatments, and importantly, there were minimal side effects, no memory issues, and a fast reorientation time after treatments, with overall improvement in verbal and nonverbal learning tasks ( ). Concurrent with the above human studies, a histological analysis in 12 macaques randomized to full courses of ECT, MST and sham demonstrated a clear absence of structural brain damage with MST treatment ( ). These early reports suggested both safety and efficacy of MST, and they set the stage for several case series and small pilot studies on the safety and feasibility of MST as a treatment for TRD.
In the first clinical trial to prospectively investigate the effects of MST in patients with TRD, Lisanby et al. studied the effect of MST in 10 inpatients in a within-subject comparison to ECT during an acute treatment course ( ). In this study, the authors treated these inpatients with two MST treatments, with a maximum output of 60 Hz treatment at 100% output for 6.6 s with successful tonic-clonic seizures in all 10 patients ( ). The authors experimented with three different coil arrangements and with different coil types as well in this study ( ). They found an overall more favorable cognitive side effect profile posttreatment with MST treatments when compared to ECT treatments on the same patients during the same treatment course ( ). This study, however, was not designed to compare the efficacy of MST to that of ECT. It is also important to note that the authors reasoned that the maximum treatment output was limited with the MST technology of that time, and they suggested that a direct comparison of efficacy and tolerability for equivalently dosed MST and ECT would be needed in the future. We review studies that address these specific issues below.
By this point, the High Dose MST device was developed to answer the issue of equivalent dosing for ECT and MST, as it was able to provide stimulation at 6 × the seizure threshold, identical to that used in right unilateral ECT. This MST device also provides 100 Hz frequency for a duration of 10 s ( ) and is currently used in major clinical trials of MST. Kirov et al. were the first to test this machine in a pilot, feasibility study in 11 patients with MDD or schizoaffective disorder ( ). They tested both frontal and vertex stimulation targets with more consistent seizures found with the vertex stimulation approach. No adverse effects were seen, and similar to that seen in the above studies, reorientation time after stimulation was much quicker with MST compared to ECT in these patients. Further technical advances in the delivery of MST would allow for more reliable seizure induction in the frontal cortex target, the main target in recent, large clinical trials described below.
Clinical studies
Recent MST studies generally incorporate larger patient samples and use more rigorous clinical trial designs than those above, which is now culminating in the large, prospective RCTs currently underway in the field. Below we explore these recent clinical studies being completed by a variety of research groups in several countries.
White et al., initially reported on the essential components of anesthesia for patients undergoing MST versus ECT. This was a prospective, nonrandomized controlled trial in which 10 patients underwent MST with 10 matched controls. One key finding from this study confirmed previous reports that MST produced a marked reduction in reorientation times compared with ECT. Another finding was that patients required less succinylcholine in MST treatment, and that this dose reduction is needed to reduce the risk of waking paralysis ( ). Authors also found that the patients who underwent MST required less antihypertensive medication, had less postictal suppression after seizures, and they also found, while still significant, there was less improvement in depression scores as indexed by the Hamilton Rating Scale for Depression. This may have been due to underdosing of patients receiving MST (1.3 × seizure threshold with MST versus 2.5 × with ECT), which was a common problem in early MST trials, as described above. There was an equivalent hemodynamic response from the two treatments. Of note, the more rapid reorientation time seen with MST here is a consistent finding evident in larger clinical trials that will be elaborated on in sections below.
In one of the earlier studies aiming to directly compare the efficacy and safety profile of MST with ECT in patients with unipolar and bipolar depression, Kayser et al. did not find any difference in clinical improvement between the groups ( N = 20, total) ( ). MST treatment was delivered with 100 Hz frequency over the vertex for up to 6 s duration, compared to RUL ECT with a pulse width of 0.5 ms. There were less cognitive side effects seen with MST versus ECT on a test of neglect, and orientation time was significantly better with MST. In this study, there were no issues with eliciting reliable seizures with MST, which had been raised as a concern in the past. Nonetheless, this was an open label study of small sample size. This group has also reported on a mixed sample of 26 patients with MDD and BD treated with 100 Hz MST up to 10 s of stimulation with 100% machine output, with the ability to treat at 6 × seizure threshold. In this study, treatment was for up to 12 sessions ( ). Authors found a 69% response rate and 46.2% remission rate in this study, with half of responders relapsing several months after treatment. They did not find differences in seizure characteristics between responders and nonresponders. Cognitive outcomes were mostly positive, with improvements in visuospatial learning and memory, visual perception, executive functioning, and worsening in verbal fluency. Kayser et al. followed this study with an analysis of predictors of response to MST with 12 additional patients added to the above group ( N = 38) ( ). They found a significant higher frequency of family history of mood disorders, fewer past MDEs, lower baseline HRSD scores, and potentially few symptoms of anhedonia and somatic anxiety in MST responders. Remitters did tend to have less anxiety symptoms as well ( ).
In their most recent report, Kayser et al. focused on the neuropsychological outcomes of MST in a group of 10 patients diagnosed with MDD or BDII ( ). Patients again were treated with 100 Hz stimulation over the vertex. Six patients responded and four remitted, and importantly, other than decreases in the German test RWT formal-lexical-verbal fluency, there were no significant decreases in other cognitive domains, including retrograde amnesia on the Autobiographical Memory Interview-Short Form (AMI-SF). There have been difficulties in properly assessing autobiographical memory in MST studies, because of inherent issues in the AMI-SF; this issue and a further exploration of the cognitive sequelae of MST in TRD is expanded on below.
Fitzgerald et al. published several studies important for the development of MST. In 2013, they published the first study in which MST was delivered three times per week, similar to common practices for acute ECT courses; MST was delivered at 100 Hz frequency over 10 s with the vertex as the brain target ( ). Thirteen patients with TRD were given up to 18 MST treatments, and while response and remission rates were lower than most other studies reported here (38.5% and 15%, respectively), improvements in depression were significant, and there were no significant adverse cognitive effects, including with memory and orientation time. As described previously by , authors here encountered the issue of early wakening paralysis due to early reorientation with MST. They concluded that alternative brain targets, including frontal and unilateral approaches should be explored, which would become the focus of later studies . In a follow-up study, Fitzgerald et al. completed the largest study to date comparing MST to ECT in TRD, with RUL ECT of 1.0 ms pulse width at three times seizure threshold, in a double-blind RCT. While underpowered ( N = 37), they did not find a significant difference in the primary depression outcomes between the two groups. They did find relatively low efficacy in both groups actually ( ), possibly due to the chronicity of illness of this group and use of propofol as the anesthetic agent, which is known to decrease the quality and duration of seizures ( ). For MST treatment, the vertex was targeted at 100 Hz frequency at 3 times per week up to 15 treatments. The authors did find that the cognitive measure of digit symbol coding improved more so with MST over ECT.
In a recent rater-blinded nonrandomized comparator trial, Zhang et al. compared treatments of 100 Hz MST over the vertex to bifrontal ECT in patients with major depressive episodes, although not clearly with TRD, for up to six treatments ( ). While the duration of this treatment trial appears to be short in comparison to trials above, authors found no significant differences in depression outcomes between groups, and did replicate findings of faster recovery of consciousness, orientation and breathing after treatment with MST compared to ECT; this extended to improvements in formal cognitive measures of memory and attention ( ).
Recently, Daskalakis et al. published the largest open-label trial of MST in TRD to date ( N = 86). Patients in this study were treated, importantly, with prefrontal MST two-to-three times per week in patient blocks divided by treatment frequency (25, 50–60, and 100 Hz treatment) up to a maximum of 24 treatments, or until remission of their depression ( ). Authors here reported a finding of potentially higher clinical efficacy on overall depression for patients receiving high frequency (100 Hz) versus low frequency (25 Hz) MST, with a response and remission rate of 41.7% and 33.5%, respectively, in this 100 Hz treatment group. High frequency MST was associated with shorter reorientation time after treatment as well. As alluded to above, while there was decline in the specific cognitive test AMI-SF in this study, there are methodological issues with this scale, as declining scores equivalent to those in this MST treatment group have been found with pharmacotherapy alone ( ; ). Authors also found improvements in visuospatial memory and delayed recall in this study. Adverse events included induction of mania in one patient, one fall and shoulder dislocation, and one case of superficial burn from MST coil. The much anticipated follow-up study, comparing MST to ECT directly in a randomized controlled trial, is now currently underway ( ClinicalTrials.gov Identifier: NCT03191058 ).
Researchers are now starting to capitalize on these early, promising results to explore novel treatment delivery schedules for MST in TRD patients. Wang et al. posited that since MST has fewer cognitive side effects than ECT, it could potentially be tolerable for patients to receive MST treatments every day for 6 continuous working days. In their small case series using this treatment schedule, these authors provided 100 Hz MST over the vertex and demonstrated early efficacy in two of three patients with no cognitive sequelae, and some actual improvement in cognition during this interval. They did not have any extended follow-up period to assess for relapse though ( ). In a similar, larger follow-up study, 15 patients were treated with 100 Hz vertex stimulation for 10 s on 6 consecutive weekdays. There was a 60% response and 47% remission rate, with some improvement in measures of memory and other neurocognitive profiles. There were no serious adverse events and no dropouts. However, there was heterogeneity in the severity of the patients’ depressive illness, some experiencing apparent mild-to-moderate episodes with limited treatment refractoriness, and many patients were started on new antidepressants the day before MST treatment began ( ). Nonetheless, the main takeaway from these recent studies is that with the milder adverse effect profile and novel mechanism of action, there is room for flexible experimentation with the MST delivery schedule that ECT cannot allow for given its cognitive side effect profile.
Adverse effects and interactions
Study of the adverse effects of MST has expectedly focused on cognitive sequelae, as described above. In general, MST has proven to be cognitively safe, and potentially pro-cognitive, in the treatment of TRD. Further evidence for this is described below. We also explore in this section other adverse effects of MST. It is important to note that the number of patients who have received MST to date is still relatively small, so there is a possibility of unforeseen, rare effects emerging in future studies.
One of the main motivating factors for the development of MST, as described above, is to create a treatment without the debilitating cognitive side effects of ECT and with similar efficacy. McClintock et al. performed a systematic review of the neurocognitive effects of MST in computer, animal and human models. They highlight the shorter reorientation time, limited retrograde amnesia, and complete absence of anterograde amnesia with MST compared to ECT, and deem MST cognitively safe ( ). Polster et al. performed an open-label study comparing specifically the effects of 100 Hz MST over the vertex and RUL ECT on memory retrieval, including cued memory, in patients diagnosed with TRD ( ). They used a within subject open-label design that lacked blinding, and treated patients with 10–12 treatments. Patients receiving MST in this study performed equally well to healthy controls on tests of delayed recall, while those receiving ECT performed worse ( ). There were no differences between groups on cued recall ( ). As described above, the evidence to date suggests that the cognitive sequelae of MST in patients with TRD is minimal, and drastically less than for those receiving ECT. The definitive head-to-head studies investigating these effects and treatment mechanisms are underway now.
In their early work, Lisanby et al. described that at the neuropathological level there have been no neuropathological changes found with MST treatment, and limited hippocampal remodeling, when compared to ECT, in animal models ( ). In terms of physiological response, in comparison to ECT in human patients, MST appears to deliver less of a sympathetic surge postseizure, and less parasympathetic mediated bradycardia ( ); this is in addition to less physical and cognitive side effects, corroborated in later studies and discussed thoroughly above. Also as described above, similar to ECT, in MST treatment there is the risk of wakening paralysis, especially during dose titrations in the initial treatment phase. While not associated with any lethality or long-term physical effects, this potential adverse effect is unpleasant for patients.
There are other potential adverse effects not related to cognition in MST treatment. As described above, due to the risk of magnetic coils overheating, there is a risk of superficial burns with this treatment ( ). also describe a potential adverse effect of MST in bipolar depression, which is also seen with ECT treatment: they describe two patients that achieved remission of their depressive episodes with subsequent emergence of mania or hypomania requiring pharmacotherapy to resolve this mood switch ( ). It was not entirely clear in these cases though that MST caused the switch in mood, as it may have been due to lithium taper in one patient, and due to natural evolution of disease in the other case. Nonetheless, manic switch from MST treatment appears to be a potential adverse effect shared in common with ECT.
Mechanism of action
There is limited understanding of the mechanisms of action of MST for relieving depression in patients diagnosed with TRD. It is assumed that the convulsion induced by MST is a necessary component, but this has not been confirmed in sham-controlled trials, as previously done with ECT ( ). Nonetheless, as MST is a relatively novel treatment, our understanding of its mechanism benefits from concurrent advances in neuroscience occurring over the past few decades. Many of the above described clinical studies were completed with parallel, add-on investigations into the neurobiological mechanisms involved. Below we summarize this intriguing work into the mechanism of action of MST in TRD, with a focus on biomarkers and predictors of response with electroencephalogram (EEG), transcranial magnetic stimulation-EEG (TMS-EEG) and neuroimaging technologies.
One consistent finding in studies of MST compared to ECT is less postictal suppression after MST. Researchers have also described shorter seizure durations, lower ictal EEG amplitude, as well as a significant effect of head diameter on the variability of MST effects ( ). The significance of these differences are explored below. However, before further exploring results from human-based studies, it is important to review the evidence from computational models of MST. Computational models are needed for estimations of electric fields produced by MST in humans, so that we can create better hypotheses of the mechanisms of MST in the brain. In vivo estimations of electric fields in live humans are not possible, and animal models are limited in themselves both by measurement technique and marked differences in neurophysiology compared to humans. To address this issue, Deng et al. completed a thorough and revealing study, which was the first computational model to compare electric field strength between MST and ECT ( ). Authors used a simplified spherical head model and compared the treatment parameters of multiple MST and ECT configurations, including double-cone MST and bilateral and right unilateral ECT. They found that MST consists of a higher activation threshold, yet similar electric field strength to ECT, and importantly, that MST electrical fields attenuate markedly more rapidly as a function of brain depth, with a field strength approaching zero at the center of the brain when compared to ECT. ECT tends to produce a strong field throughout the entire brain, especially in the case of bilateral ECT. In fact, when compared to ECT with standard parameters of 800 mA current and 0.3 ms pulse width, MST has 10–60 times more focal stimulation ( ). This finding is essential to understand the current, major hypothesis as to why MST does not produce the cognitive side effects that ECT does: the area of focus with MST does not include deeper brain structures involved in memory like the hippocampus. This notion is elaborated on above in the clinical studies section of this chapter.
Neuroimaging studies investigating the effects of MST with pre-MST and post-MST imaging have been helpful in elucidating the mechanism of action of MST. In the first study published in this area, Kosel et al. reported the first case of a full MST treatment course in a patient with TRD ( ). Along with clinical improvement, the authors observed increased frontoparietal and basal ganglia perfusion posttreatment on single photon emission computed tomography (SPECT) imaging ( ). In a study involving 10 patients diagnosed with TRD, Hoy et al. used fluorodeoxyglucose-positron emission tomography (FDG-PET) pre- and post- at least 6 completed MST treatments, and they found increased metabolism in several brain areas posttreatment, especially left-sided brain increases; specifically increases were observed in the basal ganglia (globus pallidus, substantia nigra, and putamen), as well as the orbitofrontal cortex (OFC), medial-prefrontal cortex (MPFC), and dorsolateral prefrontal cortex (DLPFC). The authors suggest that they found a “rebalancing” of signals in limbic-cortical regions ( ). This was one of the first studies to clearly demonstrate potential differences in mechanism between ECT and MST in a human subject, as ECT treatment is associated with decreases in brain activity, but here authors found clear, consistent and robust increases in brain activity, more in line with the mechanism of action of rTMS ( ). Lastly, in one study completed in parallel with a clinical trial described above, Kayser et al. treated 26 patients with MDD and BD with 100 Hz MST up to 12 sessions, and used FDG-PET imaging as an investigational biomarker ( ). They found increased brain metabolism in the frontal cortex and decreased activity in the left striatum in the 12 patients included in this analysis.
Several researchers have used simple analyses of EEG data to investigate the mechanism of action of MST. In conjunction with one of their clinical studies, Kayser et al. analyzed bifrontal EEG output to characterize the seizure quality in MST versus ECT ( ). They measured seizures in 7 patients diagnosed with TRD, and 1 with BD, whom received 12 MST treatments over the vertex at 100 Hz for up to 10 s. This was compared in open-label fashion and intraindividually to an ECT course of brief pulse width. They found no visible differences in both motor and EEG seizures between ECT and MST, although seizures were nonsignificantly longer with ECT, especially the polyspike phase. Similar to other studies, they did also find a significantly quicker reorientation time with MST versus ECT, approximately 2 versus 8 min ( ). Also, it is important to note that there was no significant clinical improvement in either group, suggesting a possible failed study ( ). Soehle et al. completed a similar analysis on separate groups of patients diagnosed with TRD receiving either MST or ECT, and added an analysis of the bispectral index (BIS), a measure of depth of anesthesia ( ). They found significantly longer seizures with ECT compared to MST, and also found faster recovery time and eye opening with MST. Interestingly, BIS was significantly lower in the ECT group at all time points compared to MST, demonstrating a lower level of wakefulness associated with this treatment, even when anesthesia doses were controlled for ( ). Last, Backhouse et al. analyzed seizure quality in patients receiving MST at low, moderate and high frequencies (25, 50–60, and 100 Hz) with fronto-mastoid EEG, and found better quality seizures in moderate and low frequency MST compared to high frequency based on a scoring system derived from the ECT literature ( ). Seizure quality correlated with improvement in suicidality ( ), but not with overall depression. Authors here hypothesized that shorter seizures, and presumably lower seizure quality in high frequency MST, which actually correlated with better overall depression improvement in MST, may be due to early transition in seizures to the slow wave phase, which may be reflective of better pyramidal cell entrainment. Overall, it is still unclear what constitutes a good quality seizure in MST treatment, and whether this is an essential component of its mechanism.
Building on this early work in EEG, several groups have attempted to further explore the complexities and richness of EEG data in order to better understand MST mechanism of action. Using resting state EEG (RS-EEG) in 21 patients with MDD, Hill et al. found that MST led to upregulation of synchronization in the theta band subnetworks of the bilateral frontal and parieto-occipital areas, possibly suggesting an overlapping mechanism of action with rTMS ( ). These authors also found decreased beta spectrum connectivity, and clinically, they demonstrated that change in theta connectivity predicted HDRS-24 improvement with a sensitivity of 89% and specificity of 67%. In a similar analysis of 34 patients with TRD treated with MST and ECT, Farzan et al. analyzed RS-EEG data and showed greater reductions in gamma frequency and in the complexity of fine timescale signal in the fronto-central and parieto-occipital regions; these changes were associated with improvement in depression for both ECT and MST. Also, greater increases in course time scales was linked to worsening cognition in ECT, which was not seen in MST ( ). One theory authors discussed here is that MST may mirror the positive changes in brain oscillations with ECT, by decoupling aberrant frontoparietal and default mode network (DMN) circuit connections in the brain, without the negative changes of increasing slow oscillations, which are associated with cognitive impairment. Atluri et al. also used RS-EEG in a comparison of TRD patients receiving ECT ( N = 22) and MST ( N = 24), as well as healthy controls. They analyzed brain microstates, which correspond to networks in the brain, and found that an increase in State A duration and a decrease in State B, C and D frequency associated with depression improvement with ECT. They also found that for MST, change in suicidality correlated with shorter duration of states A, B, C and a higher frequency of state D, possibly through alterations in the DMN, and that a decrease in the frequency of state B correlated with improved cognition for MST ( ). Similar to above, this group found that increases in slow cortical oscillations correlated with ECT and not with MST ( ). Overall, while complex and exploratory, these studies suggest a different mechanism of action on brain circuitry between MST and ECT, with evidence suggesting that the lack of cognitive adverse effects with MST may be correlated with a lack of increase in slow wave oscillations that occurs consistently with ECT. It is still unclear how these brain dynamics equate to improvement of depression in MST treatment, and how varying MST treatment frequencies affect this. Further work in these areas is needed.
A relatively novel neurobiological technology that can probe brain function combines transcranial magnetic stimulation and EEG (TMS-EEG). TMS-EEG has recently been applied as a potential biomarker for MST with the hopes of providing nuanced neurophysiologic information that can guide MST treatment selection for patients. Only one study to date has investigated this technique as a marker specifically for depression response in MST. In this study, Hadas et al. looked at TMS-EEG as an index of connectivity between the subgenual cingulate cortex (SGC) and the DLPFC. They assessed this in 31 patients receiving MST, with TMS-EEG measures pretreatment and posttreatment ( ). Reduced connectivity between the SGC and DLPFC correlated with depression response on the HRSD-24, and reductions in hippocampal activation were associated with a decline in cognition, as indexed by the MoCA scale, generally agreeing with results from the above studies in other technological modalities ( ). Sun et al. published two studies investigating TMS-EEG as a biomarker to predict response of suicidality in patients with TRD treated with MST. They found that improvement in suicidal ideation (indexed by the Beck Scale for Suicidal Ideation) was correlated with changes in the EEG measures N100 and long-interval cortical inhibition (LICI) over the DLPFC, and they suggest that these measures are possible correlates of GABA interneuron function, potentially recruited by MST through transsynaptic depolarization ( ). In their follow-up study, Sun et al. found significant correlation between change in LICI over frontal and central electrodes and change in suicidal ideation in responders; the reduction in LICI identified those achieving resolution of suicidal ideation with 90% sensitivity and 88% specificity ( ). It is hypothesized that the decreases in GABA interneuron function account for increased neuroplasticity, indexed by changes in LICI, correlating with improvements in suicidality.
Researchers have explored alternative biomarkers in recent MST studies. One study explored heart rate variability (HRV) as a biomarker for treatment response in MST. HRV is known to be decreased in patients diagnosed with MDD ( ). Noda et al. measured HRV pre- and post-MST treatment ( ). Authors here found an association between baseline HRV (i.e., root mean square of successive r-r interval [RMSSD]) and improvement in executive function, but not depressive symptomatology. This improvement in cognitive functioning was hypothesized to be mediated through normalization of DMPFC and dorsal anterior cingulate functioning, known to be important in the regulation of cardiovascular physiology.
In sum, exciting research happening now is beginning to explore and explain the neurobiological underpinnings of the mechanisms of action of MST in TRD. There is evidence suggesting dual, parallel antidepressive and antisuicidal effects in TRD patients, with cognitive sparing effects.
Other indications
The majority of studies on MST in humans have focused on MDD, and specifically TRD. Yet, there have been a number of more recent, exploratory studies on additional potential psychiatric indications for MST. There are several studies focused solely on bipolar depression. Kayser et al. was first to publish on this in a case report of a patient with BD treated with 100 Hz MST for nine treatments with good effect, and a partial relapse after 6 months ( ). Noda et al. demonstrated the safe and efficacious use of frontal MST in an adolescent who was diagnosed with bipolar disorder II depression, , treated with 18 MST sessions ( ). Tang et al. most recently published the largest trial of MST for patients diagnosed with BD in 26 patients completing an adequate trial of MST (vertex 100 Hz, or frontal 25, 50, 60, or 100 Hz) ( ). There was a 38.5% response rate and 23% remission rate with limited cognitive sequelae in this study; one patient in the study did have emergence of hypomania that was responsive to medication treatment. In parallel with the prospective, randomized trial underway in unipolar depression, a large randomized trial of ECT versus MST is currently underway for bipolar depression ( ClinicalTrials.gov Identifier: NCT03641300 ).
Several secondary analyses have explored other indications for MST. Two studies report on suicidality itself as a treatment target for MST in both unipolar ( ) and bipolar depression ( ), and authors suggest that this may be an independent treatment target separate from core unipolar and bipolar depressive illness. There has been one negative trial on the use of MST in obsessive compulsive disorder ( ), and one small trial in schizophrenia suggesting feasibility and potential efficacy for MST ( ). Other exploratory investigations are underway, including for borderline personality disorder ( ClinicalTrials.gov Identifier: NCT03361826 ).
Conclusion
MST is an emerging interventional psychiatric treatment with growing evidence to support its efficacy and safety in the treatment of TRD. Research is demonstrating that the mechanisms of action of MST on the brain differ from those of ECT, with apparently far fewer cognitive side effects. This is indeed an exciting time for research in MST, as years of translational work are now being followed by large, impactful clinical trials. MST is poised to become an essential, first line treatment for patients struggling with TRD.
References
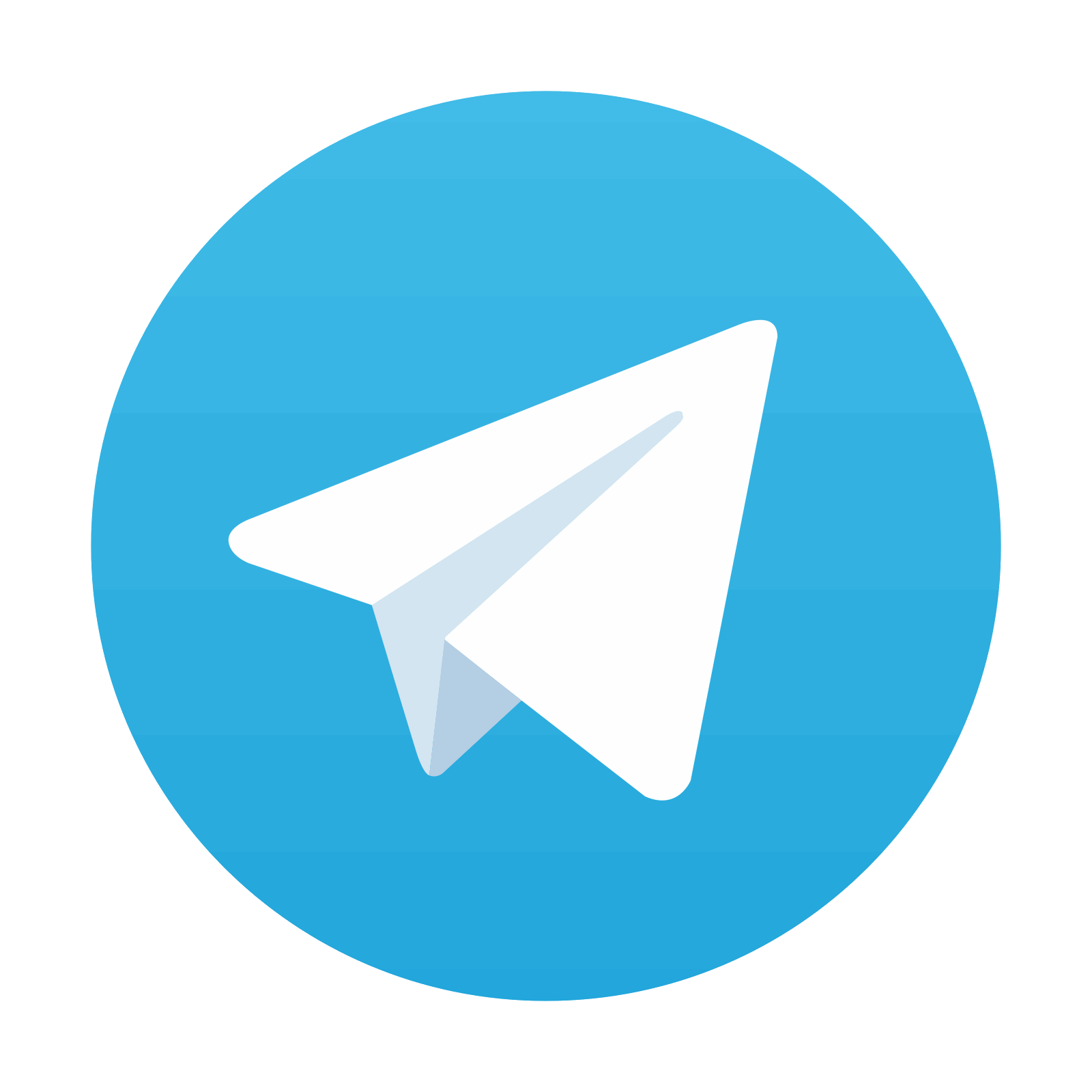
Stay updated, free articles. Join our Telegram channel

Full access? Get Clinical Tree
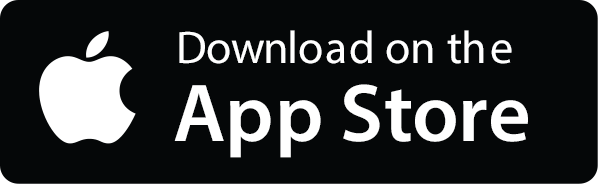
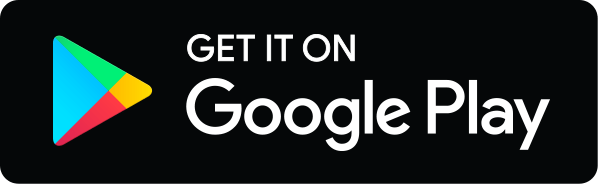
