Image registration is the process of transforming images acquired at different time points, or with different imaging modalities, into the same coordinate system. It is an essential part of any neurosurgical planning and navigation system because it facilitates combining images with important complementary, structural, and functional information to improve the information based on which a surgeon makes critical decisions. Brigham and Women’s Hospital (BWH) has been one of the pioneers in developing intraoperative registration methods for aligning preoperative and intraoperative images of the brain. This article presents an overview of intraoperative registration and highlights some recent developments at BWH.
Image registration is the process of transforming images acquired at different time points, or with different imaging modalities, into the same coordinate system. It is an essential part of any neurosurgical planning and navigation system because it facilitates combining images with important complementary, structural, and functional information to improve the information based on which a surgeon makes critical decisions.
Magnetic resonance imaging (MRI) can be used to generate both structural and functional neurologic images and thus plays an important role in planning neurosurgical procedures. High-resolution anatomic magnetic resonance (MR) images can be used to discriminate between healthy and pathologic tissue, functional MRI (fMRI) can be used to identify the location, and extent, of important cognitive areas of the brain, whereas diffusion tensor imaging (DTI) can be used to identify the white matter connectivity of the brain ( Fig. 1 ). If important cognitive areas are injured or removed during surgery, it can have an adverse effect on the patient’s quality of life after surgery.

It is especially important to put these complementary images into the same coordinate system when functional areas are located adjacent to a tumor. A map of important anatomic structures and surrounding functional areas can then be built and used by the surgeon to plan the surgical procedure. Because the brain is enclosed by the skull, a rigid transformation that consists of a translation and rotation is adequate to align these images. The importance of fMRI in planning tumor resections is underlined by the findings of Petrella and colleagues who studied the effect of therapeutic decision making in patients with brain tumors. Treatment plans before and after fMRI differed in 19 of 49 patients, with a more aggressive approach recommended after imaging in 18 patients. The availability of fMRI resulted in reduced surgical time in 22 patients who underwent surgery, a more aggressive resection in 6 patients, and a smaller resection in 2 patients.
Other modalities have been and are also currently being used in neurosurgical navigation systems. Positron emission tomography (PET) allows the function and metabolism of the brain to be analyzed. It is mainly used to localize the most malignant parts of brain tumors but has also been used to locate eloquent areas of the cortex. However, it does not provide useful structural information and is therefore combined with high-resolution structural imaging, such as MRI and computed tomography (CT), to accurately locate the functional areas in relation to anatomic structures. For planning of neurosurgical procedures, PET images are usually combined with those of MRI because they provide the best tissue contrast. fMRI has now superseded PET as a functional imaging modality for locating eloquent cortex because it eliminates any radiation exposure.
Registration also plays an important role in other neurosurgical guidance applications besides tumor removal, for instance, in resection of suspected epileptogenic centers. The epileptogenic centers are located using information from subdural electrodes, and the location of these electrodes is extracted from CT. By registering CT with structural MRI, the centers can be precisely located within the brain anatomy and used to plan the resection.
Because of brain shift, tissue resection, and retraction, the preoperative image information does not necessarily reflect the intraoperative anatomy the surgeon sees during surgery, even after rigid registration. Brain shift, a deformation of the brain tissue that occurs after the craniotomy and opening of the dura mater, is caused by various combined factors: cerebrospinal fluid (CSF) leakage, gravity, edema, tumor mass effect, and administration of osmotic diuretics. A clinical example of brain shift is shown in Fig. 2 . Reported deformations of the brain tissue caused by brain shift measure up to 24 mm, and further deformations may be induced because of tissue manipulation during the resection.

For acquiring up-to-date anatomic information during surgery, intraoperative MRI has been introduced into the surgical workflow. Intraoperative imaging during neurosurgical procedures helps identify any residual tumor tissue and leads to a significant increase in the extent of tumor removal and survival times. However, intraoperative images are often of a degraded quality compared with the preoperative images. Many factors lead to the reduced quality of intraoperative MR images: fast acquisition protocols, which are used to reduce the surgical time, intraoperative MRI scanners that often operate on lower magnetic field strengths than diagnostic MRI scanners, use of surface coils instead of head coils, and disturbances to the main magnetic field caused by the surgery.
Ultrasonography (US) is another imaging modality used to acquire up-to-date images during neurosurgery. Compared with MRI, acquisition is faster with US and does not require the patient to move, but image quality and soft tissue discrimination is reduced. US can identify functional information such as blood flow but not areas of cognitive functions, such as motor, visual, and language cortices. Reliable and accurate acquisition of intraoperative fMR images is, in general, not feasible because of factors such as time constraint, the requirement that the patient be conscious during acquisition of fMR image, and disturbances of the magnetic field from the surgical procedure.
How then can we accurately and robustly provide the surgeon with the location of these important eloquent areas in relation to the intraoperative anatomy? Nonrigid transformations have a high degree of freedom and are capable of accommodating the local brain deformations that occur during surgery. Nonrigid intraoperative registration has therefore been introduced as a way of mapping the preoperative functional information into the intraoperative space. In practice, a transformation cascade is constructed to facilitate intraoperative navigation of the preoperative image data ( Fig. 3 ). First, the functional images, typically those of fMRI and DTI, are rigidly registered to a high-resolution preoperative anatomic image. Next, the preoperative anatomic image is nonrigidly registered with the intraoperative image. Finally, to facilitate navigation of these images, the intraoperative image is registered with the physical patient space by estimating a rigid transformation from corresponding (fiducial) landmarks identified in the image and on the patient. Most commercial neurosurgical navigation systems register the preoperative images to the intraoperative space using fiducial markers and rigid registration and do not take into account the nonrigid deformations caused by brain shift.

Brigham and Women’s Hospital (BWH), an affiliate of Harvard Medical School, has been one of the pioneers in developing intraoperative registration methods for aligning preoperative and intraoperative images of the brain. This article presents an overview of intraoperative registration and highlights some of the recent developments at BWH. The rest of the article is organized as follows. First, patient-to-image registration is introduced followed by a discussion of the error involved in such estimations. Next, an introduction of image-to-image registration and the specific challenges of intraoperative registration are provided. Finally, an overview of the research that has been performed to handle these challenges is presented.
Patient-to-image registration
The primary requirement of a neurosurgical navigation system is to relate both preoperative and intraoperative images to the physical patient space, that is, to ensure that when the surgeon points a tracked device to an easily identifiable structure on the patient, the navigation system shows the pointer at the correct location in the images. The common approach is to identify a set of homologous points in the 2 spaces the image space and the physical patient space. One example is to record the positions, f = { f 1 , …, f n }, of a set of n fiducial markers attached to the skull as located using a tracking device and the corresponding set of points, m = { m 1 , …, m n }, in the image. The transformation is established by finding a rigid body transformation R point , which minimizes the root-mean-square (RMS) distances between the homologous points.
arg min R p o int ∑ i = 1 n ( f i − R p o int ( m i ) ) 2 n
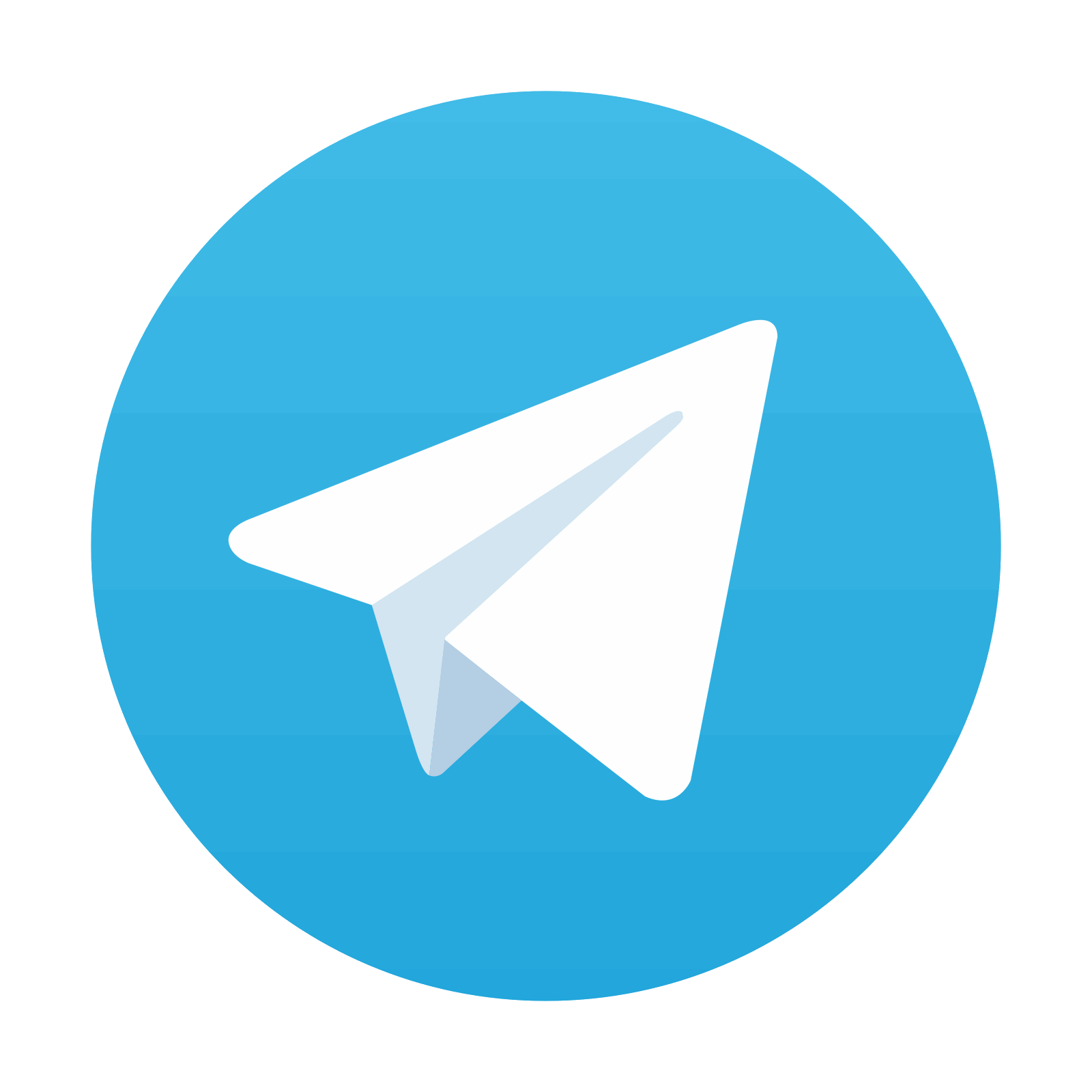
Stay updated, free articles. Join our Telegram channel

Full access? Get Clinical Tree
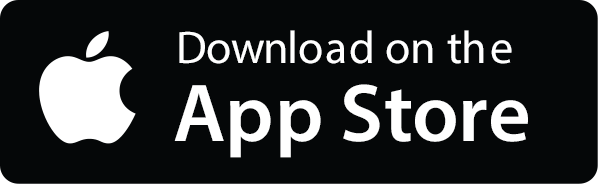
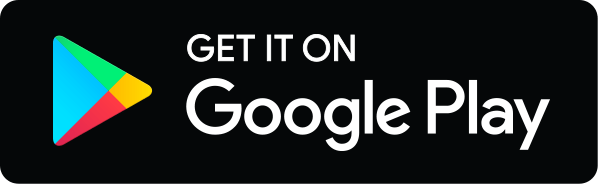