© Springer Science+Business Media New York 2017
Sudhansu Chokroverty (ed.)Sleep Disorders Medicinehttps://doi.org/10.1007/978-1-4939-6578-6_2222. Multiple Sleep Latency Test
(1)
Henry Ford Hospital Sleep Disorders and Research Center, Wayne State University Medical School, 2799 W. Grand Blvd, Detroit, MI 48202, USA
(2)
Internal Medicine, Henry Ford Health System, 2921 W. Grand Blvd, Detroit, MI 48202, USA
Keywords
Pathological sleepinessMSLT methodsMSLT normsMSLT reliability and validityCauses of sleepinessIntroduction
Daytime sleepiness as a consequence of inadequate sleep the previous night is a common experience for most adults. Because of the universality of the acute experience of daytime sleepiness, it is typically minimized as a health problem within the general population; in a 1997 Gallup Poll of Americans, only 6 % of those reporting impairing sleepiness considered it medically serious [1]. Although minimized by laypeople, increasingly chronic excessive daytime sleepiness is recognized as an important and significant symptom in medicine and public health. Furthermore, excessive daytime sleepiness can and should be distinguished from fatigue, tiredness, and lassitude, although many patients may not make such distinctions themselves unless they are carefully queried.
Representative surveys of the populations of industrialized countries have found that between 11 and 32 % of respondents report that sleepiness interferes with activities almost daily [1–5]. Sleepiness is associated with a number of medical, behavioral, and pharmacologic causes, and, regardless of its cause, has serious social and medical consequences. Nearly half of the patients with excessive sleepiness seen at sleep disorders centers report automobile accidents; more than half report occupational accidents, some life threatening; many have lost jobs because of their sleepiness; and the impact of sleepiness has a disruptive effect on family life [6]. Information on traffic and industrial accidents in the general population suggests a link between sleepiness and life-threatening events. For example, the highest rate of self-reported automobile accidents occurs between 2 and 6 am, which is remarkable because fewer automobiles are on the road during these hours [7]. A population-based study related Department of Motor Vehicle (DMV)-verified automobile accidents to excessive sleepiness as measured by the MSLT and a commonly used subjective sleepiness questionnaire, the Epworth Sleepiness Scale (ESS) [8]. Those with the lowest MSLT scores had the highest accident rates, while the ESS was not predictive (see discussion below). Shift workers, a particularly sleepy subpopulation, have the poorest job performance and the highest rate of industrial accidents among all workers [9].
Problems in assessing sleepiness became evident during early research on the daytime consequences of sleep loss before the clinical significance of sleepiness was recognized. Sleep loss compromises daytime functions. Virtually all individuals experience dysphoria and reduced performance when they do not sleep adequately. The majority of performance tasks are insensitive to the effects of sleep loss [10], but long and monotonous tasks are reliably sensitive to the changes in the quantity and quality of nocturnal sleep. Using various measures of mood, including factor analytic scales, the most consistent and systematic response to sleep loss is increased sleepiness. Among the various subjective measures of sleepiness, the Stanford Sleepiness Scale (SSS) is the best validated [11]. Yet clinicians have found that patients may rate themselves alert on the SSS even as they are falling asleep [12]. The likely reason for such discrepancies is that subjective daytime sleepiness has multiple dimensions [13].
Normal and pathologic variations in daytime sleepiness and alertness can now be directly assessed and quantified by the multiple sleep latency test (MSLT), a test of the rapidity with which a subject falls asleep in a standardized, sleep-conducive setting, repeated at 2-h intervals throughout the day. The MSLT uses standard sleep recording methods to document both the rate of sleep onset and the appearance of rapid eye movement (REM) episodes at sleep onset. Other procedures that have been used to quantify sleepiness and alertness (but because of a variety of shortcomings are not widely used), including pupillometry, subjective rating scales, and tests of vigilance or reaction time, are all correlated to some extent with the MSLT. The MSLT has become the standard method in clinical sleep disorders medicine for documenting the complaints of excessive daytime sleepiness and to document treatment success. It is also used to document sleep-onset REM periods, a diagnostic sign of narcolepsy. The American Academy of Sleep Medicine has indicated that the MSLT should be used as part of an evaluation of suspected narcolepsy and may be helpful in the evaluation of suspected idiopathic hypersomnia [14]. Since the development of the MSLT by Carskadon and Dement [15] in the late 1970s, enormous progress has been made in the scientific investigation and understanding of both normal and pathologic variations in sleepiness and alertness. The original R–K method of recording and scoring has recently been slightly modified (see Chap. 6).
Multiple Sleep Latency Test Methods
Recording Montage
General and specific technical guidelines for the administration of the MSLT have been published [16]. Briefly, the guidelines require that the standard Rechtschaffen and Kales (R-K) recording montage be used in performing the MSLT [16]. The montage includes the referential electroencephalogram (EEG) from a central (C3 or C4) placement, two horizontal referential electrooculograms (EOGs) from right and left outer canthi, and a mental or submental electromyogram. Also helpful in the determination of sleep onset is a referential occipital EEG lead, which shows alpha activity in relaxed wakefulness with eyes closed and is followed by a characteristic change to mixed-frequency EEG activity at the onset of sleep. An EOG recording with filters set to allow visualization of slow rolling eye movements (e.g., 250 ms) is another sign of sleep onset.
General Procedures
As indicated in the guidelines, to ensure a reliable and valid MSLT, a number of general procedures are necessary [16]. A 1- or 2-week sleep diary recorded before the test that includes information on usual bedtime, time of arising, napping, and drug use (i.e., caffeine, alcohol, and illicit and licit drugs) is very helpful. Deviations from the subject’s habitual sleep behavior should be noted, because sleep time accumulated or lost over the week before an MSLT can significantly affect the result. Some controversy has arisen regarding what defines adequate sleep prior to the MSLT [17, 18]. The critical point is that the sleep prior to the MSLT represents a patient’s habitual sleep at home. Central nervous system (CNS)-active drugs, as well as their discontinuation, can alter sleep and REM latencies and therefore should be discontinued sufficiently well in advance of the test. The sleep of the night preceding an MSLT should be documented with a standard nocturnal sleep recording. This nocturnal sleep recording should be scheduled to coincide with the timing and amount of the subject’s usual sleep, as revealed in the diary.
The reliability of the MSLT is based on multiple determinations of sleep latency, as the studies discussed in the following sections have shown. Consequently, as indicated in the guidelines, four or five tests of sleep latency at 2-h intervals throughout the day should be conducted [16]. Testing should be initiated from 1.5 to 3 h after the nocturnal sleep period has been terminated, typically at 9:30 or 10:00 AM. The MSLT should be conducted in a sleep-conducive environment that is quiet, dark, and controlled at a comfortable temperature. Any potentially arousing stimuli should be removed from the test area.
Specific Procedures
The following procedures are specified by the guidelines for conducting the MSLT [16]. After arising from nocturnal sleep, the subject should toilet, dress in street clothes, and eat the usual breakfast (avoiding caffeinated beverages). Between the latency tests, the subject should be kept out of the bed and monitored by technical staff to assure that no napping occurs. A small retrospective study of patients being evaluated for excessive daytime sleepiness monitored the patients telemetrically between tests and brief inadvertent napping did occur [19]. But, the naps occurred among the sleepiest patients and did not alter the clinical results appreciably. Preparations before each latency test include smoking cessation 30 min before lights out, bedtime preparation (removing shoes and restrictive clothes such as belts or neckties) at 10 min before lights out, all electrode connections and calibrations completed at 5 min before lights out, and the instructions to relax and fall asleep given 5 s before lights out.
Ending a Test
According to the guidelines, if sleep does not occur, each test is concluded 20 min after lights out [16]. For the clinical version of the MSLT, in which the occurrence of REM sleep is at question, the test is concluded 15 min after the first 30-s epoch of sleep. In a research version of the MSLT, the test is concluded after three consecutive 30-s epochs of stage I sleep or one 30-s epoch of another sleep stage. When the recording is equivocal, it is safer to allow clearer signs of sleep (i.e., spindles and K-complexes) to emerge rather than to terminate the test prematurely.
Scoring and Interpretation
Criteria for scoring sleep onset differ from criteria for test termination [16]. There has been some confusion in the MSLT literature in this regard. Furthermore, it should be noted that the MSLT sleep latency criteria differ from the typical definition of nocturnal sleep onset (stage II sleep or 10 continuous minutes of sleep) in much of the all-night sleep literature. MSLT sleep latency is the elapsed time in minutes from lights out to the first 30-s epoch scored as sleep. According to the scoring criteria of Rechtschaffen and Kales [20], this implies that 16 s of sleep (i.e., >50 % on a given epoch of the recording) is sufficient to score a sleep onset. REM sleep latency in a clinical test is scored as minutes from sleep onset (as defined earlier) to the first epoch of REM sleep.
Average sleep latency (in minutes) for the four or five latency tests is the parameter typically used to express the level of sleepiness. In some of the clinical literature, the MSLT result is expressed as a median sleep latency or a sleepiness index, which is merely the average latency subtracted from 100 and multiplied by 100 % (and corrected if fewer than five tests are conducted). In population studies, survival analyzes have been used to examine the predictors of sleep onset during the MSLT [21]. The occurrence of REM sleep within 15 min of sleep onset is generally defined as a sleep-onset REM period (SOREMP), and the frequency of such SOREMPs is also tabulated.
Sources of Error
The level of sleepiness (defined as the average sleep latency) observed on the MSLT is affected by the sleep of the previous night and weeks. Any deviation from the subject’s habitual sleep schedule (as revealed in the sleep diary) and sleep quality (as seen in the nocturnal sleep recording and the individual’s estimate of its consistency with usual sleep) is likely to overestimate or underestimate the usual level of daytime sleepiness. Similarly, the timing of the nocturnal sleep and daytime MSLT assessment relative to the subject’s circadian phase is a potential source of error, that is, an issue in studying shift and night workers. Out-of-phase sleep is likely to be disturbed and associated with shorter MSLT latencies. Moreover, sleep latency itself varies as a function of circadian phase.
Sedating or alerting effects of drugs or discontinuation of long-term drug use can also be a source of error in documenting sleepiness. For some patients, a urine drug screen may be necessary to confirm the absence of drugs. A noisy, bright, or stimulating test environment invalidates the MSLT result. In addition, stimulating activity immediately prior to a latency test will increase sleep latency on the subsequent test [22]. Instructions given to the subject at the initiation of the test are also important. Subjects should be aware that they are to close their eyes, lie still, and allow sleep to occur. Excessive tossing and turning are to be avoided. It should be recognized that the instruction “relax and fall asleep” may be emotionally loaded for patients with insomnia. This issue is discussed under the Determinants of Sleepiness section.
Finally, a “last test” effect might be observed (this has not yet been systematically studied). In anticipation of going home for the day, subjects may remain awake for the 20 min of the last latency test. This last test effect could elevate the average sleep latency for the day. It can be avoided by scheduling other non-arousing activities after the last latency test. Patient-feedback sessions with the clinician either before or after the last test can be disruptive to that test and should be avoided.
REM sleep can occur on latency tests of the MSLT as a result of a number of factors. Many drugs suppress REM sleep, and discontinuing them increases the likelihood of SOREMPs on an MSLT. For example, a study of cocaine addicts found an average of 2.8 SOREMPs on five-test MSLTs conducted on the first two days after discontinuing the cocaine use [23]. By days 13 and 14 of discontinuation, the average number had dropped to 0.2 SOREMPs. The circadian-phase timing of the MSLT is also important with respect to the occurrence of REM sleep. REM sleep can occur on early morning latency tests in a person who is a late-morning sleeper. Excessive disturbance of the sleep of the previous night also has the potential to result in REM sleep on early morning latency tests. For example, it has been suggested that apnea patients with highly fragmented sleep may have more SOREMPs than the general population. SOREMPs should be re-evaluated in patients suspected of narcolepsy, but who show apneas in the nocturnal sleep recording. It is rare for sleep restriction the week previous to an MSLT to alter REM occurrence on the MSLT.
Reliability and Validity
Reliability
Several studies of the reliability of the MSLT have been conducted. In healthy controls who maintained consistent sleep–wake schedules, the test–retest reliability of a four-test MSLT was 0.97 over a 4- to 14-month test–retest interval [24]. The test–retest interval (~6 months vs. >6 months) and the level of sleepiness (average latency of ~5 min vs. 2 min) did not affect this MSLT reliability. The number of latency tests did alter MSLT reliability: The coefficient dropped to 0.85 for three tests and 0.65 for two tests. Another study of patients with insomnia over an interval of 3–90 weeks found a test–retest correlation of 0.65 on a five-test MSLT [25].
There has also been interest in the test–retest reliability of SOREMPs in patients with narcolepsy. The current criteria require two or more SOREMPs out of five possible sleep onsets. In the only one study done to date, 28 of 30 patients had two or more SOREMPs when retested (K = 0.93, P < 0.05). Of particular interest is the finding that the REM latency on SOREMPs during the initial evaluation was also correlated with that during retesting (r == 0.64, P < 0.02) [26].
Strength of test–retest reliability for mean sleep latency and SOREMPs on the MSLT is dependent on test–retest scoring reliability. Several studies have demonstrated strong scoring reliability in clinical populations. The intra-rater scoring reliability for mean sleep latency on MSLTs from 200 patients was 0.87, and the inter-rater coefficient was 0.90 [27]. The intra-rater and inter-rater scoring reliabilities for one or more SOREMPs were 0.78 and 0.91, respectively.
Validity
The MSLT measures the speed of falling asleep on repeated tests conducted in a sleep-conducive setting, as described above. The validity of the MSLT as a measure of sleepiness rests on its response characteristics to known determinants of sleepiness, which are discussed in the following section. Additionally, in evaluating its validity, the parametric limits of average sleep latency as measured on the MSLT must be discussed. The first issue is the two anchors of the scale. By scoring definition (i.e., sleep onset is scored in 30 s epochs) one cannot fall asleep in less than 30 s, often referred to as a “floor” effect. Thus, a brief <15 s attention lapse or micro-sleep, which emerges in continuous performance assessments of excessively sleepy individuals [28], is not scored on the MSLT. Similarly, by the standard MSLT procedure, the test is terminated after 20 min of continuous wake. Thus, average sleep latencies can be no longer than 20 min, which has been referred to as a “ceiling” effect. Consequently, alerting drug effects in non-sleepy individuals can be difficult to detect, although as discussed below studies have shown alerting drug effects in volunteers whose basal average sleep latency is within a standard deviation of population norms.
Secondly, the linearity of average sleep latency across the 0.5–20 min range of the MSLT cannot be assumed, an issue that has received little investigation. A study in healthy controls compared the alerting effects of caffeine 0, 75, and 150 mg after 8 h or 5 h time-in-bed (TIB) the previous night [29]. Average sleep latency was increased by 2 min with 75 mg and 4 min with 150 mg regardless of the placebo baseline, which was 6 min and 10 min, respectively, for the 5 and 8 h TIBs. On the other hand, in patients with excessive sleepiness and MSLT scores of <6 min, an increase due to treatment in average sleep latency from 2 to 6 min may be quite different than that from 6 to 10 min.
The validity and clinical utility of the MSLT were recently reviewed [30]. It was concluded that average sleep latency and multiple SOREMPs “do not discriminate well between patients with sleep disorders and normal populations.” As the next section indicates, a number of factors cause sleepiness and multiple SOREMPs in both patients and controls. Furthermore, the excessive sleepiness of a given patient may have multiple causes. Thus, the MSLT would not be expected to have good diagnostic specificity. As to sensitivity, the review suggested that most patients with complaints of excessive sleepiness do show short average sleep latencies, although establishing the limits of normal and pathological is complex (see the section on Norms).
Determinants of Daytime Sleepiness
Quantity and Continuity of Sleep
A number of different causes of sleepiness have been identified. The degree of daytime sleepiness is directly related to the amount of nocturnal sleep. Habitual bedtime is predictive of median sleep latency on the MSLT with short bedtimes predictive of short latencies [31]. Partial or total sleep deprivation in normal subjects is followed by increased sleepiness the following day, which can reach pathologic levels [32]. Furthermore, modest sleep deprivation (as little as 1 h per night) accumulates over time to progressively increase daytime sleepiness, again to pathologic levels [33]. In normal young adults, however, increased sleep time extending TIB beyond the usual 7 or 8 h per night produces increased alertness (i.e., reduction in sleepiness) [34]. Daytime sleepiness also relates to the quality and continuity of a previous night’s sleep. Sleep in patients with a number of sleep disorders is punctuated by frequent, brief arousals of 3–15 s duration. The arousals typically do not result in awakening, as judged by Rechtschaffen and Kales sleep staging criteria [20] or by behavioral indicators, and the arousals recur in some conditions as often as one to four times per minute. The arousing stimulus differs in various disorders and can be identified in some cases (e.g., apneas, leg movements, and pain). The critical point is that the arousals generally do not result in shorter sleep, but rather in fragmented or discontinuous sleep, and this fragmentation produces daytime sleepiness [35].
Correlational evidence suggests a relationship between sleep fragmentation and daytime sleepiness. Fragmentation, as indexed by the number of brief EEG arousals, number of shifts from other sleep stages to stage 1 sleep or wake, and the percentage of stage 1 sleep, correlates with excessive sleepiness in various patient groups [35]. Fragmentation of the sleep of healthy controls has been produced by inducing 3–15 s arousals with an auditory stimulus. Studies have shown that subjects aroused at various intervals during the night demonstrate the performance decrements and increased sleepiness on the following day [36–39] and that fragmented sleep is non-recuperative [40].
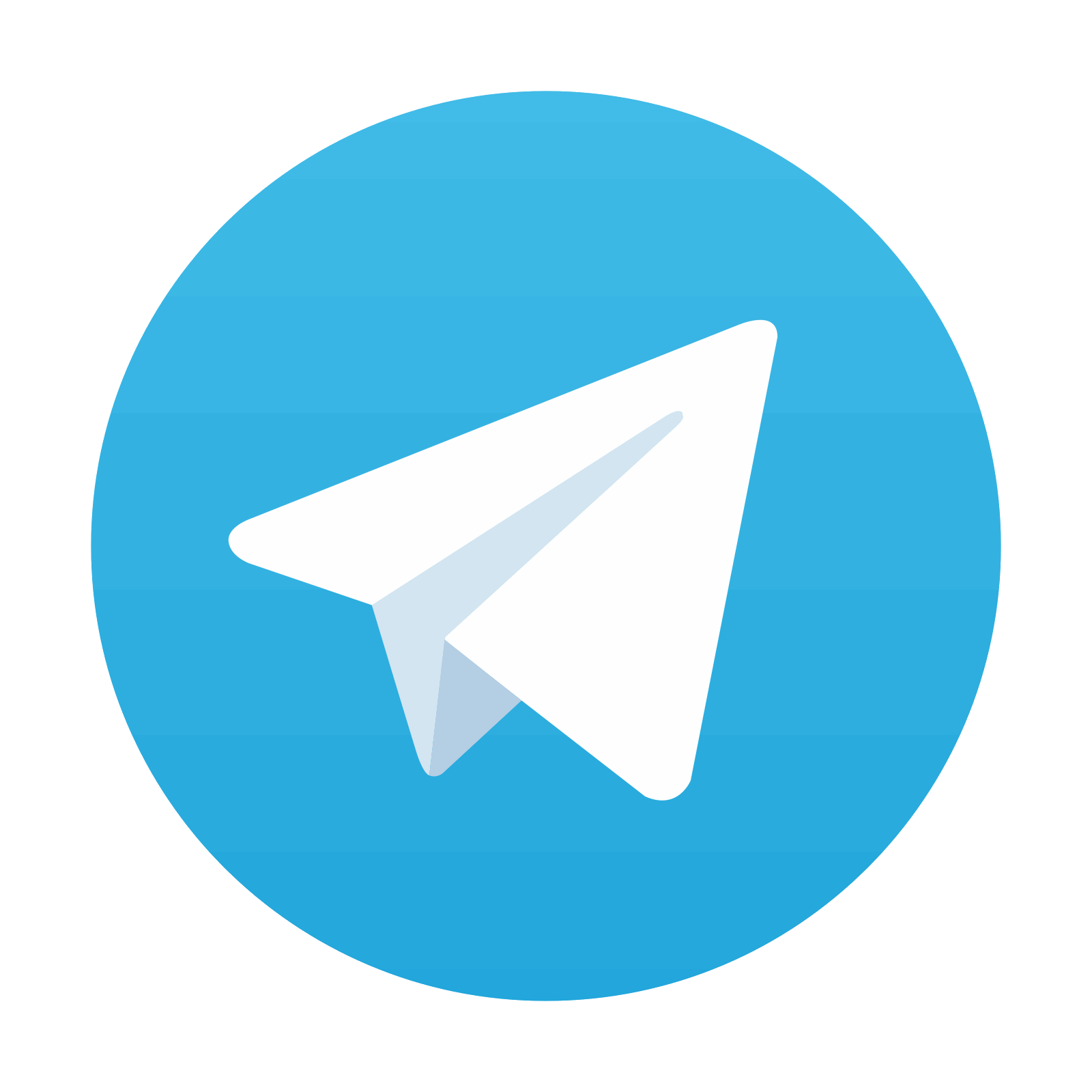
Stay updated, free articles. Join our Telegram channel

Full access? Get Clinical Tree
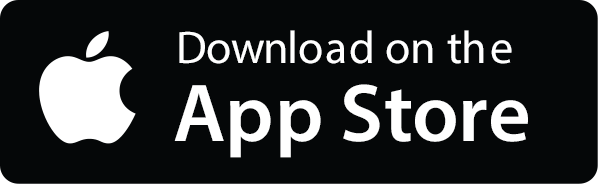
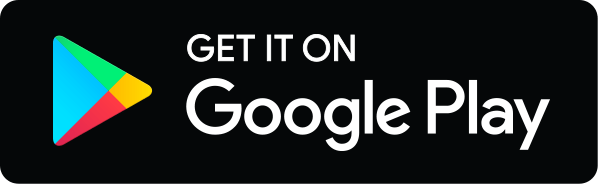