Muscle and nerve biopsies can be extremely useful in the evaluation of patients with myopathies and neuropathies. That said, not everyone suspected of having a muscle or nerve disorder needs a biopsy. In this chapter, we discuss the indications and limitations for muscle and nerve biopsies, how specific muscle or nerves are selected for biopsy, and various aspects of specimen handling. Further, we review the routine stains that are performed on muscle and nerve tissue, other stains or studies that can be performed on the tissue, and when to order them. We also mention the role of skin biopsy to assess epidermal nerve fibers in the evaluation of patients with peripheral neuropathy. This chapter is not designed to make the reader a neuropathologist. However, clinicians who take care of patients with neuromuscular disease and order biopsies should have at least a working knowledge of muscle and nerve histopathology.
Muscle biopsies are studied through a combination of various histochemistry stains on frozen sections and paraffin-embedded tissue, electron microscopy (EM), and molecular studies (e.g., enzyme assay, protein analysis by Western blot, mitochondrial DNA mutations).1–5 It is important to correlate the histopathologic findings with clinical history, neuromuscular examination, and electrodiagnostic findings.
A muscle biopsy may be helpful when the patient has objective muscle weakness, abnormal muscle enzymes (e.g., elevated serum creatine kinase [CK] levels), abnormal skeletal muscle magnetic resonance imaging, or myopathic electromyography (EMG) findings. These findings may point to a myopathy but not the exact etiology, and therefore a muscle biopsy may be indicated. That said, if the diagnosis is suspected on the basis of the phenotype and can be made by less invasive means, we generally opt for this first. For example, in a young boy with proximal weakness and large calves, we would first do genetic testing for a dystrophinopathy. Muscle biopsies are less helpful in evaluating patients with only myalgias, subjective weakness, or just slight elevations of CK in the absence of objective abnormalities.6
Muscle tissue can be obtained through an open (minor surgical procedure) or needle biopsy. A larger sample of tissue can be biopsied by the open surgery technique, and we prefer this method in patients who may have patchy pathology (e.g., inflammatory myopathies) or myopathies that require metabolic analysis (e.g., mitochondrial disorders or glycogen storage diseases), molecular studies (e.g., Western blotting and direct genetic analysis), or EM. Needle biopsy can also be technically difficult in patients with substantial subcutaneous tissue or whose muscles are atrophic and/or fibrotic. However, the yield of a needle biopsy can be quite high in laboratories that are experienced in handling the small amount of tissue obtained by this technique.7–10 The advantage of a needle biopsy is that it allows for the examination of multiple sites within the muscle and it is a less invasive procedure than an open muscle biopsy.
We select the specific muscle to biopsy based on the clinical examination, or occasionally based on skeletal muscle magnetic resonance imaging or EMG guidance. If the requesting physician is not the person who performs the surgery (the usual situation), communication between the two is essential to ensure that the proper site is chosen. Preferably, one should biopsy a mildly weak muscle in the Medical Research Council (MRC) grade 4/5 range to increase the yield. If the muscle is too weak (i.e., MRC grade 3 or less), the tissue typically has end-stage damage. It is often impossible to discern a myopathic process from severe neurogenic atrophy under these conditions. In patients with little, if any, weakness on examination, or those who might only have weakness in muscles that are not easily accessible to biopsy (e.g., iliopsoas muscle in a patient with only hip flexor weakness), needle EMG or skeletal muscle magnetic resonance imaging are used to select the muscle to biopsy. However, it is important to biopsy the contralateral muscle to the needle examination in order to avoid artifact from needle EMG.
We find that the easiest muscle to biopsy with open surgery is the biceps brachii and is our first choice if clinically affected. Other muscles that are commonly biopsied are the deltoid, triceps, and quadriceps. We occasionally biopsy the cervical paraspinals in patients with isolated neck extensor weakness or bent spine syndrome. The peroneus brevis muscle is useful to biopsy along with the overlying superficial peroneal nerve in patients suspected of having vasculitis. In patients with suspected distal myopathies, we have found the tibialis anterior, gastrocnemius, and forearm extensor muscles easy to biopsy. Otherwise, we tend to avoid the gastrocnemius or tibialis anterior muscle, because asymptomatic radiculopathies or unrelated axonal polyneuropathies may give a false impression that the primary abnormality is a neurogenic process and therefore overshadow an underlying myopathy.
In adults, muscle biopsies are performed under local anesthesia, but young children require sedation or general anesthesia. The biopsies are taken from the belly of the muscle, and it is important to avoid the region of the tendon. Each specimen should be about 1–2 cm in length and 0.5 cm in width. The specimens should be wrapped in slightly moist gauze and placed in separate labeled sterile containers until they reach the laboratory. It is important not to place the specimens in a container of saline else this will lead to artifact. Nor should the entire specimen be placed in fixative else the important histochemistry stains, protein/enzyme analysis, and mutation analysis cannot be performed. Again, this information needs to be communicated with the surgeon and the pathology laboratory. Because muscle disorders can be multifocal (e.g., inflammatory myopathies), we obtain at least two separate specimens, which are immediately frozen in isopentane cooled in liquid nitrogen. The frozen tissue is then sectioned and stained for routine histochemistry. In patients with prominent myalgias and tenderness, we may biopsy a piece of the overlying fascia to assess for fasciitis. Separate specimens may also be taken and again frozen immediately for biochemical analysis (e.g., for glycogen or lipid storage disorders and mitochondrial myopathies), mitochondrial DNA analysis, or for Western blot (e.g., in various forms of muscular dystrophy).
In addition, a separate piece of muscle tissue is fixed in formalin or Bouin’s fluid for paraffin sections. Paraffin sections can be particularly useful in inflammatory myopathies and vasculitis, as it allows for the examination of a somewhat larger piece of tissue than that used for histochemistry in cross section and longitudinal section and assesses inflammatory cells and vasculature more effectively (Fig. 3-1). However, due to shrinkage of the muscle tissue associated with the processing, the muscle fibers in paraffin sections are often appear cracked and are not ideal for the evaluation of histochemical abnormalities. Finally, an additional piece of muscle is usually taken for possible ultrastructural examination by EM. This small piece of muscle tissue is secured on a clamp or stretched out by suturing the muscle over a tongue blade, in order to prevent hypercontraction artifact. This tissue is fixed in glutaraldehyde for plastic (resin) embedding for EM.
Figure 3-1.
Paraffin sections are useful because large, longitudinal segments of muscle fibers can be cut and stained compared to frozen sections. Marked endomysial inflammatory cell infiltrate in this biopsy of a patient with polymyositis (A). On higher power, inflammatory cell infiltrates can be seen to invade the necrotic segments (B). Paraffin sections, hematoxylin and eosin (H&E).

A standard battery of histochemical stains is used for light-microscopic evaluation of frozen sections (Table 3-1).1–5 Hematoxylin and eosin (H&E) and modified Gomori-trichrome stains assess the size, shape, and cytoarchitecture of the muscle fibers, presence of internalized nuclei, destruction of fibers (e.g., necrosis) and regeneration, as well as the supporting connective tissue (e.g., increased endomysial connective tissue as seen in dystrophies) and vasculature (vasculitis) (Figs. 3-2 and 3-3). Inflammatory cell infiltration is easily appreciated with these stains. In addition, some specific abnormalities are well demonstrated with modified Gomori-trichrome stain: ragged red fibers associated with mitochondrial myopathies (Fig. 3-4A), nemaline rods (Fig. 3-4B), tubular aggregates (Fig. 3-4C), rimmed vacuoles (Fig. 3-4D), and features of myofibrillar myopathy (Fig. 3-4E).
Type 1 | Type 2A | Type 2B | |
---|---|---|---|
Gross appearance | |||
Color | Dark | Dark | Pale |
Capillary density | High | High | Low |
Muscle fiber diameter | Smallest | Large | Largest |
Comparative histochemical activity | |||
ATPase 4.3 | Strong | Weak | Weak |
ATPase 4.6 | Strong | Weak | Strong |
ATPase 9.4 | Weak | Strong | Strong |
Adult myosin heavy chain—fast | Weak | Strong | Strong |
Adult myosin heavy chain—slow | Strong | Weak | Weak |
NADH-TR | Strong | Strong | Weak |
SDH | Strong | Weak | Weak |
Cytochrome oxidase | Strong | Weak | Weak |
Glycogen content | Low | High | High |
Glycogen phosphorylase | Weak | Strong | Strong |
Myoglobin content | High | High | Low |
Lipid content | High | High | Low |
Modified Gomori trichrome | Strong | Weak | Weak |
Electron microscopy | |||
Mitochondria | Numerous | Numerous | Few |
Z-disc | Intermediate | Wide | Narrow |
Physiologic characteristics | |||
Twitch speed | Slow | Fast | Fast |
Fatigability | Resistant | Resistant | Susceptible |
Axons | |||
Diameter | Smallest | Largest | |
Conduction velocity | Slowest | Fastest | |
Other classifications | |||
Intermediate (Red) | Red | White | |
S | FR | FF | |
SO | FOG | FG | |
B | C | A |
Figure 3-4.
Modified Gomori-trichrome stain reveals a ragged red fiber in a patient with a mitochondrial myopathy (A), nemaline rods in a patient with congenital myopathy (B), tubular aggregates in a patient with myalgias (C), and rimmed vacuoles filled with debri in a patient with IBM (D). Myofibrillar myopathy is best recognized on the modified Gomori-trichrome stain as amorphous accumulation of dark green or bluish-purple debri and more distinct, denser cytoplasmic inclusions (E).

The myofibrillar adenosine triphosphatase (ATPase) is typically performed at three pHs, 4.3, 4.6, and 9.4, in order to assess the size and distribution of different muscle fiber types (Table 3-1 and Fig. 3-5). Individual muscle fibers can be classified into four different fiber types on the basis of their staining characteristics and physiologic properties: types 1 (slow twitch, fatigue resistant, and oxidative metabolism), 2A (fast twitch, intermediate fatigue resistance, and oxidative and glycolytic metabolism), 2B (fast twitch, poor fatigue resistance, and glycolytic metabolism), and 2C (undifferentiated and embryonic). In adults, only about 1–2% of muscle fibers are the undifferentiated type 2C fibers.11 The specific muscle fiber type is determined by the innervating motor neuron. The different muscle fiber types are normally distributed randomly, forming a so-called checkerboard pattern. Alterations in the random distribution of fiber such as seen with fiber-type grouping are a sign of denervation with subsequent reinnervation. Some myopathies are associated with a predominance or atrophy of a specific fiber type. For example, some congenital myopathies are associated with a predominance of type 1 fibers, which are also smaller in diameter than normal. Disuse and steroid myopathy are associated with preferential atrophy of type 2B fibers.
Figure 3-5.
The myofibrillar adenosine triphosphatase (ATPase) is typically performed at three pHs: 4.3, 4.6, and 9.4. Type 1 fibers are lightly stained, whereas type 2 fibers are dark on ATPase 9.4 stain (A). Type 1 fibers are dark, whereas type 2 fibers are light on ATPase 4.3 stain (B). The ATPase 4.6 stains type 1 fibers dark, type 2 A fibers light, and type 2B fibers in between (C).

Periodic acid–Schiff (PAS) stain is used to assess glycogen content, which may be increased in the glycogen storage disorders (Fig. 3-6). If there is abnormal PAS staining then a PAS with diastase should be performed, as glycogen is removed with diastase but more complex carbohydrates (such as polyglucosan bodies) are resistant to digestion with diastase. Loss of some enzyme activities associated with some metabolic myopathies can be detected by specific staining protocols (e.g., myophosphorylase and phosphofructokinase). Sometimes in polyglucosan body neuropathy, PAS-positive inclusions are evident in small intramuscular nerves on muscle biopsies (Fig. 3-7). Acid phosphatase stains can highlight lysosomes that are increased in certain disorders (e.g., Pompe disease) as well as macrophages that may be present in muscle tissue (Fig. 3-8). In addition, oil red O or Sudan black can evaluate lipid content, which may be increased in patients with lipid storage myopathies (Fig. 3-9). Oxidative enzyme stains (nicotinamide adenine dinucleotide tetrazolium reductase or NADH-TR, succinate dehydrogenase or SDH, cytochrome-C oxidase or COX) are useful for identifying mitochondrial and intermyofibrillar network abnormalities (Figs. 3-10 A and B). The SDH and COX stains can be combined to highly SDH-positive, COX-negative fibers characteristic of disorders associated with mitochondrial DNA mutations (Fig. 3-10C). Target fibers and central cores are also particularly well seen with the NADH-TR stain (discussed later). In addition, a so-called trabeculated or lobulated staining pattern is seen on NADH-TR in some dystrophies, although this is not a disease-specific abnormality (Fig. 3-10D). Various stains (Congo red, crystal violet, cresyl violet, and Alcian blue) can be performed to assess for amyloid deposition (Fig. 3-11).
Figure 3-6.
Scattered muscle fibers have small foci of increased glycogen deposition in subsarcolemmal regions in a patient with McArdle disease (A), periodic acid–Schiff (PAS) stain. When diastase is added to the PAS stain the abnormal accumulations are no longer evident, suggesting that the deposits were glycogen (B). PAS stain may also detect polyglucosan bodies in intramuscular nerve twigs in muscle biopsy in patients with polyglucosan body neuropathy (C).

Figure 3-8.
Acid phosphatase stains macrophages and muscle fibers lysosomes. In patients with Pompe disease, a lysosomal glycogen storage disorder, increased lysosomes are evident and brought out by acid phosphatase stain even when vacuoles may be difficult to appreciate on other routine stains such as H&E and modified Gomori trichrome.

Figure 3-10.
In addition to ragged red fibers seen on modified Gomori-trichrome stain (Fig. 3-4 A), mitochondrial myopathies may demonstrate muscle fibers with absent or reduced cytochrome oxidase staining (COX) (A) or increased succinic dehydrogenase staining (SDH) (B). The COX and SDH stains can be combined such that COX-negative fibers that are SDH-positive show up intensely blue (C). These stains are useful because ragged red fibers that are COX negative but SDH positive are usually associated with mitochondrial DNA mutations—though the primary mutation may still involve nuclear encoded genes that govern mitochondrial DNA. NADH-TR stain also highlights trabeculated or lobulated fibers as seen in this biopsy in a patient with muscular dystrophy (D).

Immunohistochemistry is important in evaluating specific types of muscular dystrophies (e.g., dystrophin staining for Duchenne and Becker muscular dystrophy; merosin and alpha-dystroglycan staining for congenital muscular dystrophy; sarcoglycans, caveolin, and dysferlin for limb girdle muscular dystrophies; and emerin for X-linked Emery-Dreifuss muscular dystrophy) (Fig. 3-12). Immunohistochemistry can also be valuable in inflammatory myopathies and vasculitis (e.g., stains for major histocompatibility antigens, complement, membrane attack complex, immunoglobulins, and appropriate inflammatory cell markers) (Fig. 3-13).
EM is used to assess the ultrastructural components of muscle fibers (e.g., the sarcolemma, sarcomeres, nuclei, and mitochondria) and vasculature (e.g., tubulofilaments in capillaries in dermatomyositis).12 Various myopathies have specific ultrastructural abnormalities that are more readily characterized by EM (e.g., nemaline rods, central cores, proliferation of abnormal appearing mitochondria, myofibrillar degeneration, vacuoles, and filamentous inclusions in nuclei and sarcoplasm) (Fig. 3-14).
Figure 3-14.
Electron microscopy is useful in assessing ultrastructural abnormalities. Normal sarcomere can be appreciated with Z-disc, thick and thin filaments, glycogen granules and mitochondria (A). In critical illness myopathy, severe disruption of the sarcomere is evident with loss of the myosin thick filaments (B). Abnormal proliferation of mitochondrial with paracrystalline inclusions in this muscle biopsy of a patient with mitochondrial myopathy (C) and rods as evident in a biopsy of a patient with nemaline myopathy (D).

Skeletal muscle is a syncytial tissue composed of sheets of individual muscle fibers with multiple nuclei. The connective tissue within muscles include the endomysium that surrounds individual muscle fibers, the perimysium that groups muscle fibers into primary and secondary bundles (fasciculi), and the epimysium that envelops single muscles or large groups of fibers. Normally, myonuclei are located adjacent to the muscle membrane (sarcolemma) and are oriented parallel to the length of the fiber. These are oval in shape and contain evenly distributed chromatin and inconspicuous nucleoli. In approximately 3% of normal adult fibers, the myonuclei lie more internal within the cytoplasm (sarcoplasm). Increased internalized nuclei are a nonspecific abnormality, as these are seen in different types of myopathies as well as in neurogenic disorders. Satellite cells are present next to the sarcolemma and are enveloped by basement membrane that surrounds the muscle fibers. Most of the sarcoplasm of the muscle fiber contains myofilaments, which form the contractile apparatus and supporting structures. Individual muscle fibers contain repeating units (sarcomeres) of interlaced, longitudinally directed thin filaments and thick filaments and perpendicularly oriented Z bands to which the thin filaments are connected (Fig. 3-14A). The sarcomere is connected to the sarcolemma via filamentous actin. The sarcolemma is composed of various protein complexes and is connected to the extracellular matrix. Greater detail of the sarcolemmal proteins and extracellular matrix is discussed in Chapter 27.
The T tubules are composed of invaginations of the sarcolemmal membrane into the interior of the muscle fibers. Their course is parallel to the Z bands and they are surrounded on each site by the sarcoplasmic reticulum. The T tubules allow for rapid depolarization of muscle membrane deep within muscle fiber cells and the accelerated release of calcium from the sarcoplasmic reticulum during excitation.
Adult muscle fibers are polygonal in appearance but are more rounded in shape in infancy and early childhood. The cross-sectional diameter of individual fibers varies depending on the specific muscle, fiber type, and age of the individual. The motor unit comprises the motor neuron and the muscle fibers it innervates. The individual muscle fibers of a motor unit are normally randomly distributed as previously mentioned, within a sector approximating 30% of the muscle’s cross-sectional diameter.
The percentages of type 1, 2A, and 2B fibers differ in various muscle groups, and it is important to be aware of the normal percentages of these fibers in the biopsied muscle for accurate assessment.13 The most commonly biopsied muscles (i.e., biceps brachii, triceps, and quadriceps) have approximately equal amounts of the three major fiber types, although the deltoid muscle has more type 1 fibers than type 2A and 2B. Because muscle fibers from a single motor unit are randomly distributed among muscle fibers of different motor units and fiber types, a checkerboard or mosaic pattern is appreciated on ATPase stains (Fig. 3-5).
Although ATPase stain is primarily used to assess fiber type, we can often ascertain the fiber types from other standard stains (Table 3-1). For example, type 1 fibers stain more intensely with modified Gomori-trichrome, lipid, and oxidative enzyme stains than type 2 fibers because of the increased number of mitochondria and oxidative metabolism associated with type 1 fibers. In contrast, type 2 fibers, which are involved with glycolytic metabolism, stain more intensely with PAS, as these contain more glycogen but are lighter staining on modified Gomori-trichrome, lipid, and oxidative enzyme stains.
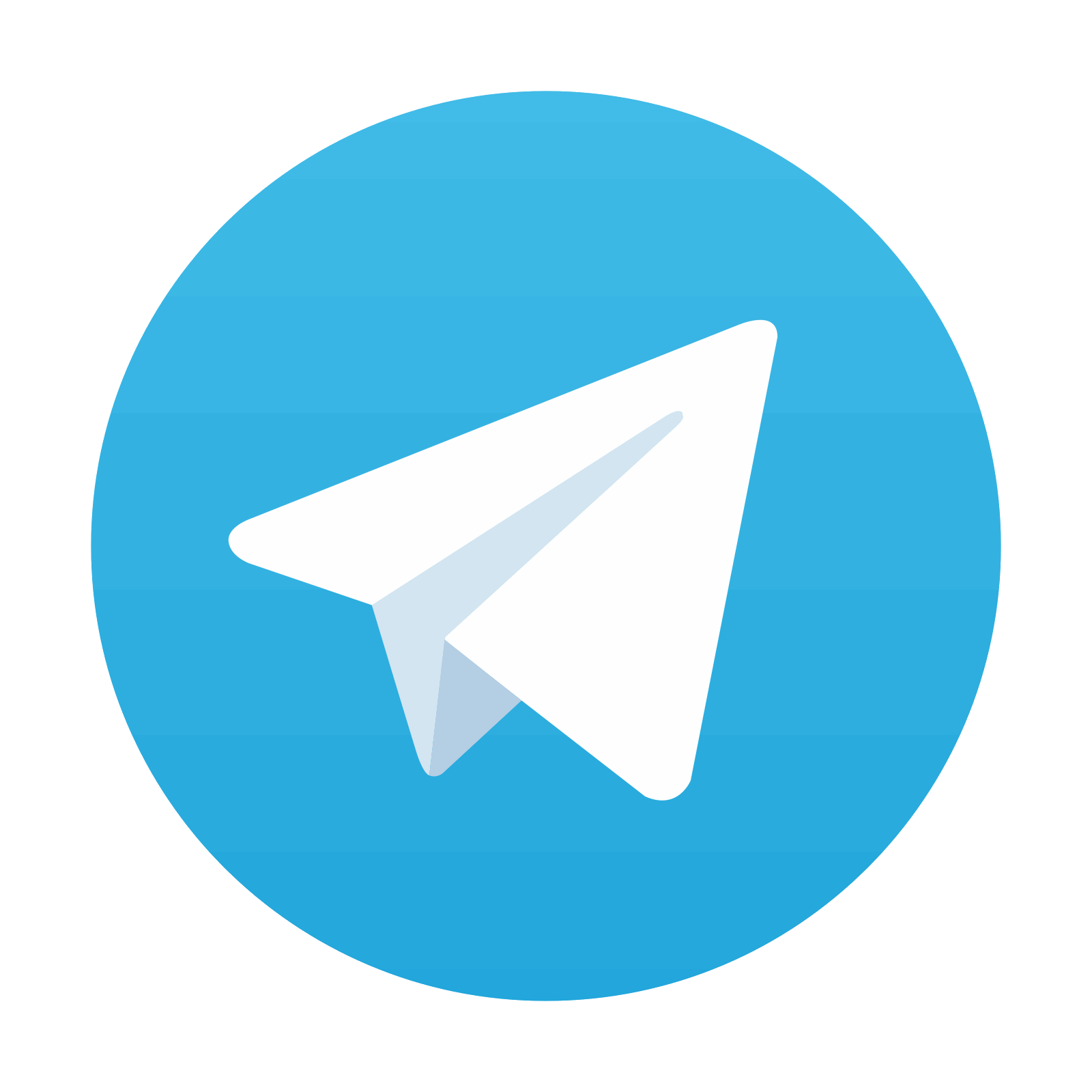
Stay updated, free articles. Join our Telegram channel

Full access? Get Clinical Tree
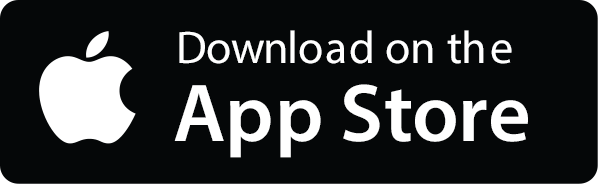
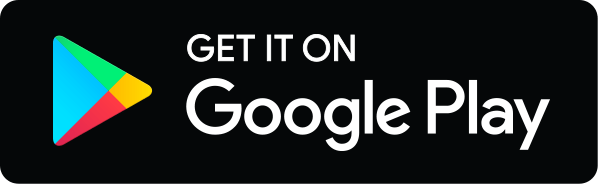