Orientation of the muscle biopsy. (a) Larger specimens can sometimes be oriented with the naked eye, but in many cases, the use of a dissecting microscope can ensure that cross sections of the fresh frozen specimen are obtained. (b) A properly oriented muscle segment. The fresh tissue has been mounted on the surface of a small mound of gum tragacanth. Note that the specimen is not fully embedded in the mounting medium. This ensures that a maximum amount of the specimen’s surface area is exposed to the chilled isopentane (2-methlybutane) during the subsequent freezing process

Freezing the muscle biopsy. (a) The basic material for properly freezing muscle biopsies consists of an insulated, wide-mouthed container for liquid nitrogen, a metal beaker that can be suspended into the liquid nitrogen and a supply of isopentane. (b) To freeze the biopsy, the metal beaker is filled with isopentane and partially immersed into the liquid nitrogen, taking care to not allow the liquid nitrogen to come into direct contact with the isopentane. A white rind will form on the inner surface of the metal beaker when the isopentane has been cooled to an appropriate temperature for freezing the specimen. The oriented, mounted muscle segment is then completely immersed in the chilled isopentane and gently stirred for about 50 seconds. Once frozen, the specimen can be transferred to the cryostat for sectioning or, alternatively, stored in a sealed plastic bag at −70 to −80° C for later processing
In order to prepare frozen sections, the cork holding the specimen should be immobilized on a standard cryostat chuck using OCT embedding medium. Sections should be cut in a cryostat maintained at a temperature of −20 to −25° C. Biopsies that have been stored at −70 to −80 °C should be allowed to warm to cryostat temperatures in order to avoid shattering the tissue during sectioning . Sections of tissue destined for routine staining, including enzyme histochemical staining, should be cut at thicknesses of 10 μm, while those used for immunohistochemical staining should be cut a thicknesses of 6 μm. In our laboratory, sections are picked up directly onto commercially prepared positively-charged glass slides (Superfrost™ Plus), although sections can also be placed on standard glass coverslips for staining if desired. If the cryostat sections are not to be stained immediately, the slides are placed in a folder and kept in a 4 °C refrigerator until ready for use. Ideally, cryostat muscle sections should be stained within 24 hours of the time that the sections are cut.
Although skeletal muscle morphology at the light microscopic level is best demonstrated in cryostat sections of properly frozen muscle, fixed muscle can also provide useful diagnostic information in a number of important muscle disorders. In the case of open biopsies, muscle destined for fixation should be clamped in situ using either a single use, disposable Becton Dickinson Rayport® polypropylene clamp or a reusable Price muscle biopsy clamp. The clamped muscle segment is gently excised from the surrounding skeletal muscle, placed into 10% neutral buffered formalin, and transported to the laboratory. Once in the laboratory, the fixed muscle segment is excised from the clamp. Representative longitudinally oriented sections measuring no more than 1 mm in thickness are transferred to 3% buffered glutaraldehyde for resin embedding and, in selected cases, subsequent electron microscopy. The remainder of the skeletal muscle segment is divided into cross and longitudinal sections and placed into standard histological cassettes for paraffin histology, discussed below. Muscle tissue obtained via percutaneous needle biopsy is also suitable for fixation. Muscle fragments obtained via needle biopsy can be placed directly into fixative—either 10% neutral buffered formalin or 3% buffered glutaraldehyde—and transported to the laboratory.
Routine Histological Stains
Non-enzymatic stains (frozen sections)
Hematoxylin and eosin (H&E)
Modified Gomori trichrome
Oil Red O
Non-aqueous periodic acid Schiff (PAS) with and without diastase digestion (usually reserved for cases with suspected glycogen storage abnormalities)
Crystal violet
Congo red
Enzyme histochemical stains (frozen sections)
Myosin adenosine triphosphatases (ATPases) at pH 4.3, 4.6 and 9.4
Nonspecific esterase
Acid phosphatase
Alkaline phosphatase
NADH-tetrazolium reductase
Succinate dehydrogenase (SDH)
Cytochrome-c oxidase (COX)
Sequential COX-SDH
Myophosphorylase
Phosphofructokinase
Myoadenylate deaminase
Non-enzymatic stains (paraffin sections)
H&E
Masson trichrome
Congo red
Morin stain (reserved for cases of suspected aluminum adjuvant-associated myopathies)
Most of the staining of frozen (cryostat) sections is performed by placing the mounted sections in new 5-slide capacity plastic slide mailers. Coplin jars are used for the same purpose in some institutions, but if used, must be scrupulously cleaned between staining runs to avoid cross contamination. The use of new slide mailers minimizes the risk of such cross contamination. Individual stains and their uses are described in the following paragraphs. Technical information about individual staining procedures is available in published protocols included in the references at the end of this chapter [1, 9–18].
Normal skeletal muscle is composed of a mixture of three different types muscle cells, each of which has a fairly predictable staining profile in the panel of stains listed above. Type 1 myofibers , also known as “slow twitch” fibers , generate most of their energy via oxidative metabolism, and, as such, have abundant mitochondria and lipid, and less glycogen. Type 1 fibers are relatively resistant to fatigue, and are adapted to prolonged, endurance-type activities. Type 2 myofibers , or “fast twitch” fibers , generate more of their energy via glycolysis and contain more abundant glycogen and have less lipid and fewer mitochondria than their type I counterparts. Type 2 myofibers can be further subdivided into type 2a (fast twitch mixed oxidative/glycolytic) fibers and type 2b (fast twitch glycolytic) fibers. Type 2a fibers can change into type 2b fibers and vice versa, depending upon an individual’s exercise habits. Whether of myofiber is a type 1 or a type 2 fiber, however, is determined by the lower motor neuron that innervates it and remains constant if its innervation remains intact. The metabolic profile of a given myofiber influences its staining properties, as discussed in the following paragraphs.
Non-enzymatic Stains (Frozen Sections)

Normal muscle (H&E and Gomori trichrome stains) . (a) Normal skeletal muscle, H&E. In a properly prepared skeletal muscle segment, the myofibers have a fairly uniform, polygonal appearance in cross section. The sarcoplasm of normal muscle fibers stains pink H&E stains, due to the presence of contractile proteins (myofibrils). In normal muscle myofiber nuclei characteristically lie just beneath the sarcoplasm except in the vicinity of myotendinous insertion sites, where internalized nuclei are fairly common. (b) Normal skeletal muscle, Gomori trichrome. The Gomori trichrome stain is an indispensable stain in the evaluation of frozen muscle biopsies. The contractile proteins stain green in the Gomori trichrome stain, while the membrane-rich elements that lie between the myofibrils (mitochondria, sarcoplasmic reticulum and T-tubules) stain red-purple, imparting a delicate stippled appearance to the sarcoplasm. Type 1 myofibers have larger numbers of mitochondria than type 2 fibers and stain more darkly in the Gomori trichrome stain
The Gomori trichrome stain is familiar to most pathologists as a useful stain for demonstrating collagen, which stains with a light green color in frozen sections. In frozen sections of skeletal muscle, the modified Gomori trichrome stain developed by Engel and Cunningham [9] plays an even more important role in highlighting a variety of normal and abnormal structures within the sarcoplasm of muscle fibers. Normal mitochondria and sarcotubular elements, which are visible as punctate blue structures in H&E sections, stain red in the Gomori trichrome stain owing to the affinity of sarcoplasmic membranous structures for the Chromotrope 2R dye used in the procedure (Fig. 1.3b). Abnormal collections of mitochondria produce characteristic “ragged red” change in type I myofibers in many patients with mitochondrial disorders (discussed below under “Interpretation of the Biopsy”). Tubular aggregates, discussed below in the paragraphs dealing with biopsy interpretation, are also highlighted in the Gomori trichrome stain . Additional structures that are reliably highlighted in the trichrome stain include nemaline rods and cytoplasmic bodies (both derived from sarcomeric Z-bands), and the membranous debris present in sarcoplasmic vacuoles in patients with inclusion body myositis. Figures 1.18–1.20, which will be discussed later, contain examples of some of the abnormal structures that are highlighted in Gomori trichrome-stained cryostat sections. The appearance of collagen in Gomori trichrome-stained cryostat sections is illustrated in Fig. 1.21, which will also be discussed later.

Oil red O and PAS-stains of frozen muscle . (a) Normal muscle, oil red O. The oil red O stain is a very useful stain for demonstrating neutral lipids in frozen muscle, which appear as delicate red sarcoplasmic droplets. The lipid content of type 1 myofibers is higher than that of type 2 fibers. (b) Lipid storage myopathy, oil red O. Abnormal sarcoplasmic lipid accumulation can be seen in a wide range of conditions, including primary lipid storage myopathies, as illustrated here. Increased amounts of lipid are also seen in mitochondrial disorders and as a nonspecific reaction to myofiber injury. The abnormal lipid deposits appear as larger droplets than those seen in normal muscle. In extreme cases, these large droplets can occupy most of the cross sectional area of the affected myofiber. (c) Normal muscle, PAS. The PAS stain is the standard stain for demonstrating glycogen and basement membrane material. Because type 2 myofibers have a higher concentration of glycogen than type 1 fibers, they stain more intensely in the PAS stain. Incubation of the tissue section with diastase (amylase) will remove particulate glycogen, but will not affect the staining of basement membranes. (d) Glycogen storage disease, PAS. Abnormal glycogen deposits (arrow) stain with a magenta color in the PAS stain. In this biopsy from a patient with McArdle disease (muscle phosphorylase deficiency), particulate glycogen accumulates in subsarcolemmal regions. Pre-treatment with diastase will remove such deposits. In some conditions, exemplified by glycogen storage disease type IV and polyglucosan body disease, the abnormal glycogen deposits are composed of filamentous rather than particulate glycogen and will persist in the tissue after diastase digestion
Periodic Acid-Schiff (PAS) stains have been used for decades to demonstrate carbohydrates in tissues, including glycogen and other molecules with carbohydrate components (e.g., glycoproteins and glycolipids). PAS stains are especially important in the evaluation of biopsies from patients with suspected glycogen storage diseases. Carbohydrate-rich structures, including glycogen, stain magenta in the PAS stain (Fig. 1.4c, d). Incubation of the tissue with diastase before staining will remove particulate glycogen, but will leave other carbohydrate-bearing structures (e.g. basement membranes) intact. The PAS reactivity basement membranes and of abnormal filamentous forms of glycogen of the type that accumulate in type IV glycogenosis and polyglucosan body disease is not affected by diastase digestion. It is worth noting that fixation of tissue in aqueous fixatives prior to staining often removes delicate particulate glycogen from the tissues, preventing adequate demonstration of abnormal glycogen stores.
The Congo Red stain is an essential stain for demonstrating amyloid deposits. The term “amyloid” does not refer to a specific protein, but rather to a heterogeneous group of proteins that have in common a tendency to aggregate into a β-pleated sheet configuration. Amyloid deposits can be encountered in skeletal muscle in patients with sporadic systemic amyloidosis, most commonly associated with plasma cell dyscrasias and abnormal immunoglobulin light chain (particularly lambda light chain) production. Less commonly, amyloid deposits can be seen in hereditary forms of amyloidosis associated with abnormal deposits of transthyretin and, less commonly, a number of other proteins. Interestingly, amyloid deposition is also seen in two forms of autosomal recessive limb girdle muscular dystrophy – LGMD 2B (dysferlinopathy) [20] and LGMD 2 L (anoctamin-5 deficiency) [21]. In all of the preceding conditions, the amyloid is deposited extracellularly – most commonly within vessels walls and around individual myofibers. Intracellular amyloid deposits also occur in some conditions, notably variants of inclusion body myositis (IBM) . In this disorder, the intracellular amyloid deposits are composed of β-amyloid, the type of amyloid encountered in the central nervous system in Alzheimer’s disease and amyloid angiopathy.

Amyloid deposits , Congo red stain . (a) Amyloidosis, Congo red under polarized light. The classic method for the demonstration of amyloid deposits at the light microscopic level is the Congo red stain. When viewed under polarized light, the pink-staining amyloid has a characteristic “apple green” birefringence. (b) Amyloidosis, Congo red with epifluorescence illumination. When Congo red-stained sections are viewed under epifluorescence illumination with Texas red filtration, amyloid deposits have a bright red appearance. This technique is a more sensitive method for detecting amyloid deposits than polarized light microscopy, but is somewhat less specific
Enzyme Histochemical Stains (Frozen Sections)

ATPase stains . (a) Normal muscle, ATPase 9.4. Cryostat sections stained for myosin ATPase activity are the most reliable way to distinguish slow-twitch (type 1) from fast-twitch (type 2) subpopulations. In this section of deltoid muscle stained for ATPase activity at pH 9.4, type 2 fibers are dark, while type 1 fibers are pale. (b) Normal muscle, ATPase 4.3. Staining a section of the same muscle for ATPase activity at pH 4.3 reveals a reverse staining pattern, with the more numerous type 1 fibers staining darkly. (c) Normal muscle, ATPase 4.6. Staining of normal skeletal muscle at pH 4.6 typically reveals three levels of staining intensity: type 1 fibers are dark, type 2A (mixed oxidative-glycolytic) fibers are pale, and type 2B (fast twitch glycolytic) fibers stain with intermediate intensity. (d) In normal skeletal muscle, the different myofiber subtypes intermingle in a random distribution. If a muscle is denervated and then re-innervated, axonal sprouts from regenerating motor axons innervate contiguous myofibers, resulting in the formation of groups of contiguous myofibers with the same histochemical staining profile (“fiber type grouping”)

Hydrolytic stains – esterase, acid phosphatase and alkaline phosphatase stains. (a) Normal skeletal muscle, esterase. The esterase stain highlight normal neuromuscular junctions (arrow). The brick-red reaction product in these structures is caused by the presence of the enzyme acetylcholinesterase, and serves as a reliable internal control for the esterase stain. (b) Denervation atrophy, esterase. The esterase stain is also an effective way to identify atrophic denervated myofibers (arrow), which stain with the same brick-red appearance as normal neuromuscular junctions. (c) Myophagocytosis, acid phosphatase. The acid phosphatase stain produces a bright red reaction product in areas of increased enzyme activity, and serves as a reliable marker for lysosomal activity. Activated macrophages contain numerous lysosomes and are highlighted in the acid phosphatase stain, as in the case of these macrophages engulfing the sarcoplasm of a necrotic muscle fiber (arrow). (d) Hydroxychloroquine myopathy, acid phosphatase. Abnormal lysosomal activity is a feature of some toxic myopathies. The excessive lysosomal activity in this muscle from a patient with hydroxychloroquine myopathy is responsible for the increased acid phosphatase reactivity in the sarcoplasm of many of these myofibers. (e) Myofiber regeneration, alkaline phosphatase. The alkaline phosphatase stain produces a dense black reaction product. This figure highlights areas of abnormal alkaline phosphatase reactivity associated with myofiber regeneration (arrow). (f) Inflammatory myopathy, alkaline phosphatase. Abnormal perimysial connective tissue reactivity (arrow) is a feature of inflammatory myopathies associated with the presence of anti-Jo1 and other anti-synthetase antibodies
The acid phosphatase stain is based on the hydrolysis of naphthol AS-B1 phosphate by endogenous acid phosphatase to form naphthol, which, like the naphthol generated in the esterase stain, forms an insoluble azo dye in the presence of basic fuchsin [1, 11]. The red reaction product highlights macrophages (Fig. 1.7c) and other structures that have lysosomal activity, such as degenerating myofibers and pathological sarcoplasmic inclusions associated with abnormal lysosomal activity (Fig. 1.7d). The stain is generally a more sensitive marker of lysosomal activity than the nonspecific esterase stain.
The alkaline phosphatase stain is another “hydrolytic” stain that relies on the hydrolysis of an alpha-naphthyl acid phosphate substrate by endogenous alkaline phosphatase to generate naphthol, which reacts, in turn, with a diazo salt (fast blue RR salt) to form a black reaction product at sites of alkaline phosphatase activity. Glycerol or a comparable aqueous mounting medium should be used to coverslip the section, as alcohols or xylene dissolve the reaction product [13]. The alkaline phosphatase reaction generates gas that can produce distracting bubbles if the section is coverslipped prematurely. To minimize this, it is advisable to wait at least 45 minutes before placing the coverslip over the stained section. The stain highlights the sarcoplasm of regenerating myofibers (Fig. 1.7e), as well as occasional myofibers in infantile denervation of the type seen in spinal muscular atrophy type 1 (Werdnig-Hoffmann disease); normal muscle fibers lack alkaline phosphatase activity. Increased alkaline phosphatase reactivity can also be seen in connective tissue in many inflammatory disorders of skeletal muscle. Increased perimysial connective tissue reactivity, in particular, is a feature of some inflammatory myopathies associated with the presence of circulating anti-Jo1 or other anti-tRNA synthetase antibodies (Fig. 1.7f).

Oxidative stains – NADH-TR, succinate dehydrogenase (SDH) and cytochrome c oxidase (COX). (a) Normal muscle, NADH-TR . The NADH-TR stain is highlights both mitochondria and sarcoplasmic reticulum. It is a useful stain for highlighting a wide range of structural sarcoplasmic abnormalities. In normal muscle, as illustrated here, type 1 fibers stain more intensely than type 2 fibers. (b) Normal muscle, SDH. The SDH stain has the same reaction product as the NADH-TR stain, and appears very similar to the NADH-TR stain in normal muscle. Unlike the NADH-TR stain, the SDH stain only highlights mitochondria, and is a more sensitive stain for highlighting abnormal mitochondrial aggregates. Owing to their greater numbers of mitochondria, type 1 fibers stain more intensely than type 2 fibers. (c) Mitochondrial myopathy, SDH. Abnormal mitochondrial accumulation is associated with intense blue sarcoplasmic reactivity in the SDH stain (arrow). (d) Normal muscle, cytochrome c oxidase (COX). The COX stain is another stain that highlights only mitochondria, in this case with a brown reaction product. In normal muscle, the pattern of COX reactivity parallels that of SDH reactivity, with type 1 fibers staining more intensely than type 2 fibers. (e) Mitochondrial myopathy, COX. Many mitochondrial myopathies are characterized by abnormalities in COX activity (complex IV in the mitochondrial respiratory chain). Affected muscle fibers in such cases fail to react when stained for COX reactivity (arrows). (f) Mitochondrial myopathy, sequential stain for COX and SDH. The sequential COX-SDH stain increases the sensitivity of detection of COX-deficient myofibers. In patients with mitochondrial myopathies associated with COX deficiency, the myofibers that fail to stain for COX activity do not precipitate a reaction product, and retain enzyme activity. If subsequently stained for SDH reactivity, the COX-deficient myofibers stand out as blue-staining fibers surrounded by brown, COX-reactive fibers
The succinate dehydrogenase (SDH) stain identifies a flavoprotein enzyme that is complex II in the mitochondrial respiratory chain. The enzyme is anchored to the inner mitochondrial membrane and is encoded exclusively by nuclear – as opposed to mitochondrial – DNA. SDH normally catalyzes the conversion of succinate to fumarate and the closely linked transfer of electrons to ubiquinone to form ubiquinol in the mitochondrial electron transport chain. In vitro, the SDH stain relies on the ability of SDH to release hydrogen from its sodium succinate substrate and reduce aqueous nitro blue tetrazolium to form a blue tetrazolium precipitate. The stained sections are coverslipped using an aqueous mounting medium [1, 11, 14, 15] The SDH stain is a specific stain for mitochondria, and a much more sensitive marker for mitochondrial aggregation than either the Gomori trichrome stain or the NADH-TR stain. Myofibers harboring abnormal mitochondrial aggregates appear as “ragged blue” fibers in the SDH stain. A normal SDH stain and an SDH stain in a patient with abnormal mitochondrial accumulation are illustrated in Fig. 1.8b, c.
The enzyme cytochrome c oxidase (COX) is complex IV in the mitochondrial respiratory chain. The enzyme is composed of multiple subunits, three of which are encoded by mitochondrial DNA in mammalian tissue. The COX complex is located in the inner mitochondrial membrane, where it transfers electrons from the cytochrome c protein to molecules of dioxygen to form water. The COX stain is based on the ability of the enzyme to transfer electrons from an artificial diaminobenzidine tetra hydrochloride substrate to produce an insoluble brown diaminobenzidine precipitate (Fig. 1.8d). Stained sections are coverslipped using an organic mounting medium [1, 11, 14, 15]. Like SDH, COX is a specific marker for mitochondria. Its usefulness in diagnostic work is based on the fact that many mitochondrial disorders are associated with COX deficiency, characterized by failure of affected myofibers to stain in the COX preparation (Fig. 1.8e). In most mitochondrial disorders associated with COX deficiency, SDH reactivity is preserved, and staining sections sequentially for COX and then SDH activities [15] makes detection of COX-deficient fibers a bit easier, with COX-deficient fibers standing out as blue fibers surrounded by brown-staining fibers with intact COX activity (Fig. 1.8f). COX-deficient fibers are common in a number of conditions including primary mitochondrial disorders, including inclusion body myositis and polymyositis with mitochondrial abnormalities [23]. COX deficient myofibers are also seen in some cases of dermatomyositis, wherein there is a selective loss COX activity in perifascicular myofibers [23, 24]. COX is a fairly unstable enzyme, and care must be taken to distinguish artifactual loss of COX activity associated with improper handling of muscle tissue from true COX deficiency; this staining pattern is illustrated later in Fig. 1.24c, under the discussion of Artifacts in Skeletal Muscle Biopsies.
Two standard enzyme histochemical procedures are available for the detection of enzymes associated with glycogen metabolism – muscle-specific phosphorylase (myophosphorylase) and phosphofructokinase. Myophosphorylase is an enzyme that catalyzes the conversion of glycosyl residues in glycogen to glucose-1-phosphate, which is then used in the generation of ATP. Detection of myophosphorylase is based on the ability of the enzyme to add glucose residues to a glycogen primer in the presence of a large amount of glucose-1-phosphate substrate (the reverse of the reaction sequence that occurs in vivo). The resultant glycogen is then stained with Lugol’s iodine solution, resulting in a gray-brown reaction product. Sections are coverslipped using an aqueous mounting medium [11, 16]. Patients with myophosphorylase deficiency (type V glycogen storage disease, also known as McArdle’s disease) classically present with histories of cramps, exercise intolerance and episodes of rhabdomyolysis following vigorous exercise. In muscle biopsies from such patients, no phosphorylase reaction product is detectable in intact muscle fibers, which appear yellow under the microscope. Myophosphorylase is a labile enzyme that is subject to artifactual degradation, and it is important to distinguish such artifactual losses of enzyme activity from true phosphorylase deficiency. Smooth muscle cells in intramuscular blood vessels express a different isoform of phosphorylase than skeletal muscle, and the identification of residual phosphorylase activity in blood vessel walls serves as an internal control that distinguishes artifactual loss of enzyme activity from selective myophosphorylase deficiency. Of note, the reaction product in the myophosphorylase stain will fade over time, and it is helpful to make a notation of a “positive” result on the slide label. The color of the reaction product can be restored by re-incubating the section in Lugol’s solution.
Phosphofructokinase (PFK) is a glycolytic pathway enzyme that converts fructose-6-phosphate to fructose-1,6-diphosphate. Patients with PFK deficiency (type VII glycogen storage disease, or Tarui’s disease) have a clinical presentation similar to that of patients with myophosphorylase deficiency. The PFK stain is based on the conversion of exogenous fructose-6-phosphate to fructose-1,6-diphosphate by endogenous PFK. A metabolite of fructose-1,6-diphosphate – diphosphoglyceric acid – is formed in vitro, which then reacts with exogenous nicotinamide adenine dinucleotide to generate reduced nitro blue tetrazolium, the latter forming the usual blue precipitate. Sections are coverslipped using an organic mounting medium [1, 11, 17]. PFK is a labile enzyme that deteriorates rapidly in improperly handled biopsies. Patient sections stained for PFK should include a negative control (no added substrate) and a control section using fructose-1,6-diphosphate as a substrate, the latter always generating a blue reaction product, even if the patient is PFK-deficient. Patient sections stained for PFK activity should always be paired with a normal control section.
A final enzyme histochemical stain that is routinely performed in our laboratory is the myoadenylate deaminase (MAD) stain . MAD is an enzyme that removes an ammonia molecule from adenosine monophosphate (AMP) to convert AMP to inosine monophosphate. Deficiency of the enzyme should be suspected when patients fail to generate a normal amount of ammonia during exercise testing. The clinical significance of MAD deficiency appears to vary from patient to patient. While some patients with MAD deficiency are asymptomatic, MAD deficiency has been associated in other patients with a clinical syndrome of exercise intolerance and exercise-induced muscle cramps and pain, and, in severe cases, rhabdomyolysis. A susceptibility to malignant hyperthermia syndrome has been suggested by some authors [25, 26]. The MAD stain is based on the generation of ammonia from an exogenous AMP substrate and subsequent reduction of nitro blue tetrazolium to form a blue reaction product. Staining for MAD is always paired with a negative control, in which aqueous citrate is added to the reaction in place of AMP substrate. Stained sections are coverslipped using an aqueous mounting medium [1, 18]. In patients with MAD deficiency, no blue reaction product is present in the sections stained with the AMP substrate. In addition to detecting MAD deficiency, the MAD stain reliably highlights tubular aggregates (see discussion of abnormal sarcoplasmic inclusions under “Interpretation of the Biopsy”, below).
Non-enzymatic Staining of Fixed Skeletal Muscle (Paraffin Sections)
Although most of the useful diagnostic information in muscle biopsies at the light microscopic level is provided by the evaluation of stained cryostat sections, properly processed paraffin embedded tissue can also be of help in some conditions, particularly those characterized by the presence of inflammatory infiltrates and microorganisms. The morphology of inflammatory cells is generally better preserved in fixed, paraffin embedded tissue than in cryostat sections, and immunohistochemical markers (discussed below) often label inflammatory cells more clearly in paraffin-embedded tissue than in frozen tissue. Paraffin sections of skeletal muscle biopsies are routinely stained with H&E, Masson trichrome and Congo red stains in our laboratory. The H&E and Congo red stains have the same applications in paraffin sections as they do in cryostat sections. The Masson trichrome stain is a sensitive stain for highlighting connective tissue in paraffin sections. An additional stain, the Morin stain , has proven to be helpful in the detection of aluminum deposits in patients with macrophagic myofasciitis (see discussion of cellular infiltrates under “Interpretation of the Biopsy”, below) [27].
Immunohistochemical Stains
Immunohistochemical staining is a technique that allows one to localize specific proteins in tissue sections. The procedure has broad applications in all areas of anatomic pathology, and now plays an indispensable role in the evaluation of a wide range of muscle disorders [1, 28, 29]. In brief, immunohistochemical staining involves incubation of a tissue section with a primary antibody directed against the protein of interest, followed by incubation of the tissue with a secondary antibody that recognizes the primary immunoglobulin molecule and, finally, labeling the secondary antibody with a molecule that can be visualized under the microscope. Both fluorescent and non-fluorescent labels are commercially available. Technical aspects of immunohistochemical staining are presented in detail in other references [1], and will not be reviewed here. Automated procedures are now available for immunohistochemical staining, greatly improving turnaround time for this procedure.

Immunohistochemical staining of skeletal muscle. (a) Polymyositis, MHC class 1 (MHC1). MHC1 staining has been an invaluable tool in the assessment of suspected inflammatory myopathies. Sarcolemmal MCH1 reactivity can be seen in injured muscle fibers in a wide variety of conditions, but in classical polymyositis, one usually sees generalized reactivity involving both injured and morphologically normal fibers. Similar patterns of diffuse reactivity can be seen in inclusion body myositis and in some cases of dermatomyositis, related “overlap” inflammatory myopathic processes and in some dystrophies. (b) Dermatomyositis, MHC1. In many cases of dermatomyositis, one encounters selective staining of the sarcolemma in perifascicular myofibers, as shown here. In other patients with dermatomyositis, more diffuse sarcolemmal reactivity can be seen. (c) Becker muscular dystrophy, β-spectrin. When staining muscle biopsies for one of the dystrophy-associated membrane proteins, it is important to ensure that abnormalities in sarcolemmal staining are due to a specific protein abnormality rather than a nonspecific loss of membrane integrity due to cell injury or artifact. In this field, sarcolemmal β-spectrin reactivity is well preserved. (d) Becker muscular dystrophy, dystrophin carboxy terminus. In a contiguous section of the muscle biopsy shown in c, there is considerable loss of sarcolemmal dystrophin reactivity . The presence of intact β-spectrin staining in the same area ensures that the loss of dystrophin staining is caused by a true dystrophin abnormality rather than by nonspecific muscle fiber damage or an artifactual loss of immunoreactivity
Antibodies, Preferred tissue, and Significance
Antibody | Preferred tissue | Significance |
---|---|---|
MHC class 1 | Cryostat sections | Diffuse upregulation in polymyositis, inclusion body myositis, some cases of dermatomyositis; selective perifascicular upregulation in some cases of dermatomyositis |
C5b-9 | Cryostat sections | Capillary reactivity seen in dermatomyositis, myopathy with pipestem capillaries, diabetes mellitus |
β-spectrin | Cryostat sections | Essential control stain to ensure membrane integrity when evaluating muscle for sarcolemmal dystrophy-associated proteins |
Dystrophin epitopes (rod domain, carboxy terminus, amino terminus) | Cryostat sections | Evaluation of suspected Duchenne / Becker dystrophy and other dystrophin-related disorders |
Sarcoglycans (α, β, γ, δ) | Cryostat sections | Evaluation of suspected sarcoglycanopathies (LGMD∗ types 2C, 2D, 2E, 2F) |
Caveolin-3 | Cryostat sections | Evaluation of suspected LGMD∗ type 1C, rippling muscle disease, unexplained hyperCKemia |
Dysferlin | Cryostat sections | Evaluation of suspected LGMD∗ type 2B, Miyoshi myopathy and related myopathies |
α-dystroglycan | Cryostat sections | Evaluation of suspected congenital muscular dystrophies, LGMD∗ types 2I and 2M |
Merosin (80 kDa and 300 kDa epitopes) | Cryostat sections | Evaluation of suspected merosin-deficient congenital muscular dystrophies |
Collagen VI | Cryostat sections | Evaluation of suspected Ullrich or Bethlem myopathies |
Collagen IV | Cryostat sections | Control stain for collagen VI staining |
Emerin | Cryostat or paraffin sections | Evaluation of suspected Emery-Dreifuss muscular dystrophy |
CD3 | Paraffin sections | Pan T-lymphocyte marker |
CD4 | Paraffin sections | Helper T-lymphocyte marker |
CD8 | Paraffin sections | Cytotoxic/killer T-lymphocyte marker |
CD20 | Paraffin sections | B-lymphocyte marker |
CD68 | Paraffin sections | Macrophage marker |
Desmin | Cryostat or paraffin sections | Evaluation of suspected myofibrillar myopathies |
αB-crystallin | Cryostat or paraffin sections | Evaluation of suspected myofibrillar myopathies |
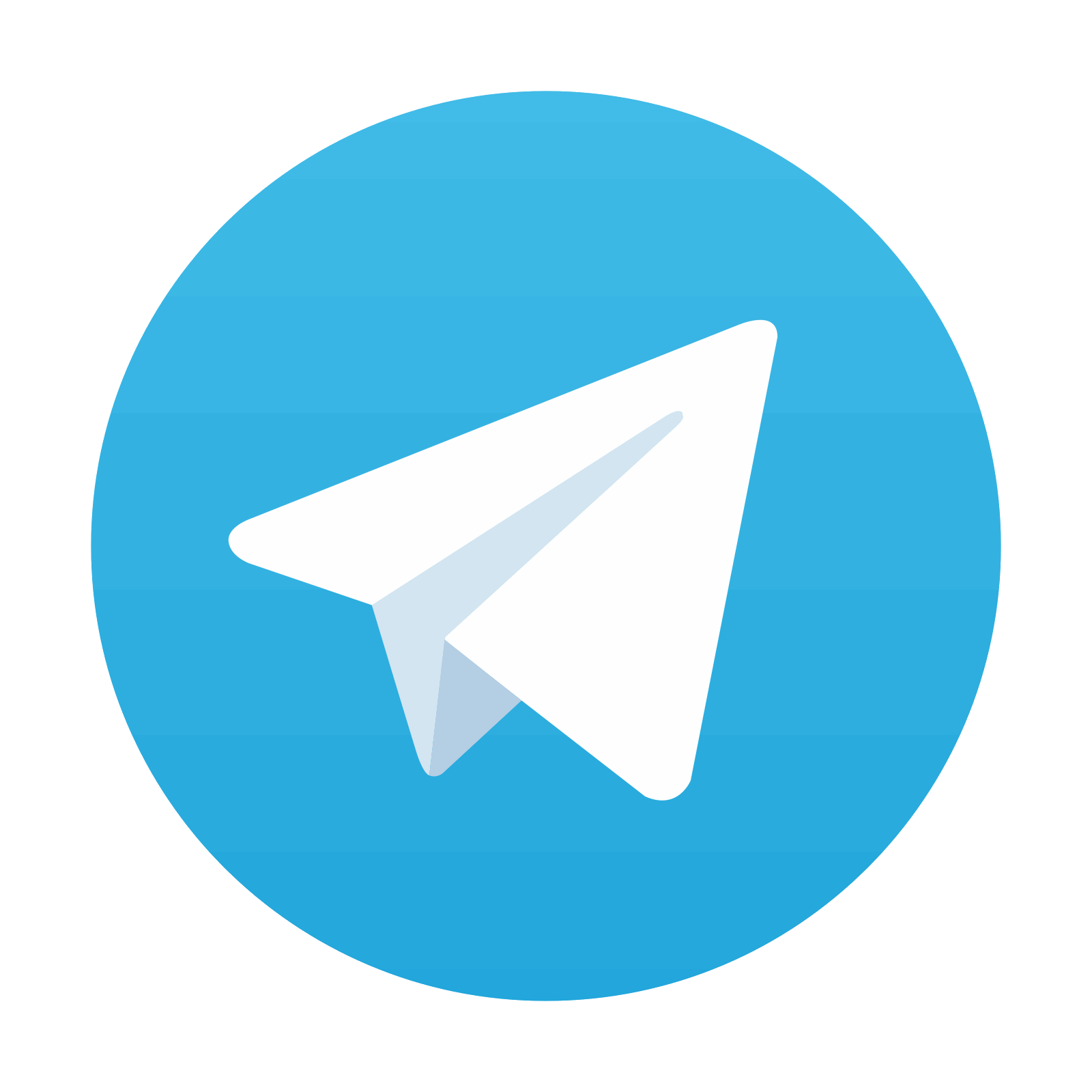
Stay updated, free articles. Join our Telegram channel

Full access? Get Clinical Tree
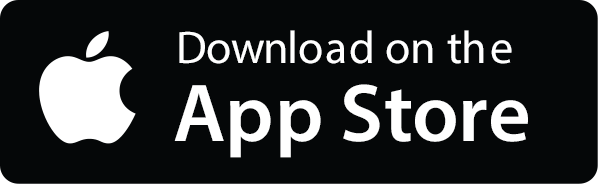
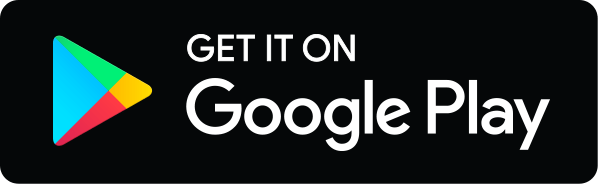