Abstract
Disorders of muscle account for a substantial proportion of infants affected with hypotonia and weakness. Although a relatively large number of myopathic disorders may cause manifestations in the neonatal period, only a few disorders account for most of the cases observed. Myotonic dystrophy is the most frequent. Myopathic disorders with nondiagnostic histology include congenital muscular dystrophy, facioscapulohumeral dystrophy, polymyositis, and minimal change myopathy; those with a diagnostic histology include core myopathies, nemaline myopathy, myotubular myopathy, congenital fiber type disproportion, mitochondrial myopathies, and metabolic myopathies. Several disorders of the neuromuscular apparatus are restricted to a small portion of the motor system; restricted disorders that are not established as caused principally by trauma are considered in this chapter. Detection of any of these disorders is valuable for purposes of genetic counseling, determination of prognosis, and institution of therapy.
Keywords
neonatal, neuromuscular, hypotonia, myopathy, myotonic dystrophy, histology
Muscle, the final component of the motor system, is the site of abnormalities with those essential clinical manifestations of hypotonia and weakness that unify the other disorders of the motor system (see Chapter 32 ). In this chapter, we deal with important myopathic disorders in the neonatal patient. In addition, certain restricted disorders of the motor system are reviewed.
Level of the Muscle
Disorders of muscle account for a substantial proportion of infants affected with hypotonia and weakness. Although a relatively large number of myopathic disorders may cause manifestations in the neonatal period, only a few disorders account for most of the cases observed. Myotonic dystrophy is the most frequent (see subsequent sections). However, more of the myopathic disorders can be detected in the neonatal period if the physician’s index of suspicion is appropriately high. Detection is valuable for purposes of genetic counseling, determination of prognosis, and institution of therapy.
The major myopathic disorders of interest in newborns may be categorized into two broad groups: those in which the histology, although sometimes impressive, is not distinctive enough to be diagnostic, and those in which the histology is distinctive enough to be diagnostic (with some qualifications) ( Box 33.1 ). In the following discussions, major emphasis is placed on the most common disorders.
Histology Not Diagnostic
Congenital myotonic dystrophy
Congenital muscular dystrophy
Facioscapulohumeral dystrophy
Polymyositis
Minimal change myopathy
Histology Diagnostic
Central core disease
Nemaline (rod body) myopathy
Myotubular (centronuclear) myopathy
Congenital fiber type disproportion
Multiminicore disease
Mitochondrial myopathies
Cytochrome c oxidase deficiency
Mitochondrial DNA depletion
Metabolic myopathies
Glycogen disorders
Lipid disorders
Myopathic Disorders With Nondiagnostic Histology
Several disorders are characterized by neonatal hypotonia and weakness and by muscle histological findings that are sometimes impressive, although not distinctive enough to be diagnostic. This category includes the most frequently encountered examples of myopathic disease with clinical manifestations evident in the neonatal period. Myotonic dystrophy is the most common of all, although congenital muscular dystrophy (CMD) is not rare (see Box 33.1 ). Facioscapulohumeral dystrophy (FSHD) rarely manifests in the neonatal period but is important to recognize. Polymyositis is a rare but treatable muscle disorder in the newborn. Congenital myopathy with minimal or no change as determined by current laboratory investigation (i.e., minimal change myopathy) represents a shrinking group as diagnostic techniques improve.
Congenital Myotonic Dystrophy
Congenital myotonic dystrophy is an inherited disorder of muscle that exhibits numerous distinctive differences from myotonic dystrophy type 1 (DM1) of adult patients. The disorder may be associated with muscle weakness so severe that death results in the newborn period. The hallmark of congenital myotonic dystrophy is hypotonia, rather than myotonia, as observed in adult patients. The congenital form of myotonic dystrophy was first described clearly by Vanier in 1960.
Clinical Features.
The disorder is usually apparent in the first hours and days of life. Certain characteristics of the pregnancy often precede the neonatal disorder ( Table 33.1 ) and tend to be prominent in the most severely affected cases. Thus, spontaneous abortion or premature birth with the associated increased risk of germinal matrix/intraventricular hemorrhage occurs in the most severely affected infants. Polyhydramnios is a common characteristic of the pregnancy (see Table 33.1 ). Indeed, this sign is almost invariable in the most severely affected infants and is thought to be caused by the disturbance in swallowing. Polyhydramnios in a mother with myotonic dystrophy is a fairly reliable indicator of serious involvement of the fetus. Abnormalities of labor, which may be either prolonged or abbreviated, have been attributed to maternal uterine muscle involvement.
CLINICAL FEATURE | CASES EXHIBITING FEATURE a (%) |
---|---|
Reduced fetal movements | 68 |
Polyhydramnios | 80 |
Premature birth (<36 weeks) | 52 |
Facial diplegia | 100 |
Feeding difficulties | 92 |
Hypotonia | 100 |
Muscle atrophy | 100 |
Hyporeflexia or areflexia | 87 |
Respiratory distress | 88 |
Arthrogryposis | 82 |
Edema | 54 |
Elevated right hemidiaphragm | 49 |
Transmission by mother | 100 b |
Neonatal mortality | 41 |
Intellectual disability in survivors | 100 |
b Paternal transmission has been documented, albeit very rarely.
The clinical features in the neonatal period are usually marked and characteristic (see Table 33.1 ). In the well-established case, the most striking clinical features are facial diplegia, respiratory and feeding difficulties, arthrogryposis (especially of the lower extremities), and hypotonia. The facial diplegia imparts the characteristic tent-shaped appearance to the upper lip ( Fig. 33.1 ). The respiratory difficulties relate to weakness of respiratory muscles, impaired swallowing and handling of secretions, and occasionally diaphragmatic disturbance ( Fig. 33.2 ). At least 80% of patients require mechanical ventilation. The respiratory impairment may be so severe that the newborn infant fails to establish adequate ventilation and suffers an asphyxial episode that so dominates the clinical syndrome that the myopathic origin of the respiratory failure is overlooked. The feeding difficulties involve both sucking and swallowing and are related particularly to weakness of the facial, masticatory, and pharyngeal musculature. (A role for myotonia of pharyngeal muscles is possible. ) A disturbance of gastric motility, a manifestation of the smooth muscle involvement in this disorder, may contribute in a major way to the feeding difficulties in some infants. Arthrogryposis ( Fig. 33.3 ) is almost invariable and usually involves at least the ankles, leading to clubfoot deformity. Indeed, in one large series of arthrogryposis multiplex congenita, 50% of the patients with cases related to muscle disease had congenital myotonic dystrophy. Hypotonia is also essentially invariable, accompanied by weakness and, in approximately 90% of the cases, by areflexia or marked hyporeflexia. Atrophy is usually obvious, especially after the first days of life, when edema, which is often excessive in these infants, subsides. Clinical myotonia, elicited by percussion of such muscles as the deltoid, has been detected as early as 3 hours of age, but it is usually not readily elicited until much later in infancy.



Less severely affected infants may go undetected in the newborn period. Facial weakness and hypotonia are the two most common manifestations in such patients.
The clinical course relates clearly to the severity of the disease. Neonatal mortality is generally approximately 15% to 20%, but it is as high as approximately 40% in severely affected patients. Feeding difficulties, which initially may require institution of tube feedings, usually improve, at least in reported survivors. a
a References .
However, approximately 20% of infants surviving to adolescence and young adulthood have major gastrointestinal symptoms (e.g., diarrhea and abdominal pain), presumably reflecting smooth muscle involvement. Moreover, the persistence of some degree of pharyngeal and palatal weakness leads to recurrent otitis media in approximately 25%, occasionally with accompanying hearing loss. Like the neonatal feeding difficulties, the respiratory difficulties subside over the ensuing weeks. However, approximately one third of patients require mechanical ventilation for longer than 30 days. Even after cessation of mechanical ventilation, ventilatory reserve may be compromised in severely affected infants, and death secondary to respiratory complications in association with anesthesia or aspiration may occur later in infancy. Unlike the general improvement in neonatal feeding and in respiratory difficulties, facial diplegia usually becomes more obvious as baby fat disappears; ptosis often becomes apparent as well. Muscle weakness, which is initially generalized or more marked proximally, begins to assume the distal preponderance characteristic of the adult disease. However, in many patients, truncal and appendicular muscle strength improves gradually over time; thus they acquire the ability to sit independently, bear weight on their legs, and eventually walk ( Fig. 33.4 ). Clinical myotonia becomes elicitable later in infancy ( Fig. 33.5 ) and is present in the majority of patients after the age of 5 years. Cardiac muscle involvement, manifested by electrocardiographic abnormalities, also becomes more prominent later in infancy. However, in very severely affected newborns, cardiomyopathy may be apparent in the newborn period and may contribute to neonatal demise. (Cataracts, baldness, gonadal atrophy, and marked facial atrophy develop later in adult life.)

Impairment of motor and intellectual development is apparent in the first weeks of life (see Table 33.1 ). Intellectual disability is essentially invariable in infants with congenital myotonic dystrophy who survive the neonatal period. Intelligence quotient (IQ) scores in the 50 to 65 range have generally been observed. The disturbance of intellectual development is nonprogressive. The only available data on relatively long-term follow-up (i.e., ≈10–30 years) indicated that only 2 of 42 patients managed normal education , and only one was gainfully employed . Some improvement in motor function occurs in late infancy and childhood.
Transmission of myotonic dystrophy in patients with the neonatal form of the disease is essentially always through the mother (see Table 33.1 ). Only approximately seven cases of congenital myotonic dystrophy transmitted through an affected father have been reported. At any rate, it is critical for the physician to be cognizant of the disorder as it appears in adults. Indeed, it is common for the mother with an affected infant to be unaware that she has the disease. Most helpful in recognition of the disease in the mother is the typical facies of myotonic dystrophy ( Fig. 33.6 ), characterized by atrophy of the masseter and temporalis muscles, ptosis, and a straight, stiff smile. Myotonia is prominent and readily elicited. Thus active myotonia is seen in the affected woman when she closes her eyes tightly and then is unable to open them fully for several seconds or more, or when she clenches her fist tightly and then cannot extend her fingers immediately on command. Passive myotonia is demonstrable by percussion of such muscles as the deltoid, thenar eminence, and tongue and by observation of the prolonged dimpling caused by sustained muscle contraction ( to ). Other characteristics (e.g., cataracts, disturbance of intellect, and cardiac abnormality) may also be present. The essential point is that the physician should examine the mother when this diagnosis is considered in a newborn.

Laboratory Studies.
Serum creatine kinase (CK) level, cerebrospinal fluid (CSF) protein concentration, and nerve conduction studies are normal. Slender ribs are commonly observed on chest radiographs (see Fig. 33.2 ) and may suggest the diagnosis in an infant thought initially to have primary asphyxia. This finding can be observed with other congenital myopathic disorders as well as with Werdnig-Hoffmann disease.
The diagnosis is supported particularly by demonstrating myotonic discharges on the electromyogram (EMG). These discharges are difficult to elicit in the neonatal period, although they have been observed as early as 5 days of age. Electrical myotonia is elicited by movement of the recording needle or by direct percussion of the recorded muscle; this procedure results in repetitive electrical potentials of up to 100/second that wax and wane in frequency and amplitude ( Fig. 33.7 ). These potentials produce the characteristic dive bomber sound. Later in infancy, particularly after the age of 2 to 3 years, myotonia is elicited more easily, and small-amplitude, short-duration, and polyphasic potentials, typical of myopathic disease, can also be demonstrated. Fibrillation potentials may also be observed.

Ventricular dilation has been observed in approximately 80% of newborns studied by ultrasonography, computed tomography (CT), or magnetic resonance imaging (MRI). In one study, macrocephaly was present in approximately 70% of the infants in the presence or absence of ventricular dilation. However, head circumference within the upper half of the normal range is more common than is overt macrocephaly. The ventricular dilation is nonprogressive and is not accompanied by rapid head growth or signs of increased intracranial pressure. The occasional occurrence of periventricular echodensities on ultrasound scans or hyperintensity on subsequent T2-weighted MRI scans may reflect periventricular leukomalacia occurring in association with neonatal respiratory disturbance. However, this white matter change could also reflect an abnormality of myelination. A small corpus callosum was documented by MRI in four of seven infants in another study. The possibility of an abnormality in neuronal development was raised by the demonstration by magnetic resonance (MR) spectroscopy of decreased levels of N -acetylaspartic acid, a neuronal marker (see Chapter 4 ), in 5 infants and children with congenital myotonic dystrophy.
Pathology.
Muscle pathology in congenital myotonic dystrophy is different from that observed in the adult with the disease and consists particularly of features of immaturity. In a detailed postmortem analysis of three infants, Sarnat and Silbert and others demonstrated particular involvement of limb muscles around arthrogrypotic joints, pharyngeal muscles, and the diaphragm. The features indicative of a disturbance of maturation included small round muscle fibers with large internal nuclei and sparse myofibrils, reminiscent of fetal myotubes ( Fig. 33.8 ). Moreover, differentiation of fibers into distinct histochemical types was incomplete. Similarly, electron microscopy showed dilated transverse tubules that were aligned longitudinally, like fetal myotubes, as well as poorly formed Z-bands, simple mitochondria, peripheral halo of absent mitochondria, and many satellite cells, which are also features of immature muscle. Investigators have suggested that these changes principally represent an arrest in fetal muscle maturation. Others have viewed these changes as signs of abnormal development. Pathological findings in pancreas (nesidioblastosis), kidney (persistent renal blastema), and other organs (cryptorchidism and patent ductus arteriosus) support the concept of a disturbance of maturation.

The neuropathological substrate for the intellectual failure is unclear. A study of three adult patients with subnormal IQ scores by Rosman and Rebeiz revealed pachygyria in two and disordered cortical architectonics with neuronal heterotopias in all three. These findings were not observed in a later study of four infants from the same institution. However, another report from a different institution also described cerebral cortical neuronal heterotopias in four infants and neuronal heterotopias in two studied postmortem. Thus the neuropathological basis for the consistent impairment of intellect is not currently established conclusively. It seems reasonable to postulate that an aberration of maturation, as in muscle and other organs, is present. A study of the organizational aspects of brain development (see Chapter 7 ) would be of interest.
Pathogenesis and Etiology.
The disorder is inherited as an autosomal dominant trait through the mother in virtually every case . In addition, the risk of congenital myotonic dystrophy bears a distinct relationship with the disease in the mother. The earlier the onset and the more severe the maternal disease, the greater is the risk for the severe congenital form of myotonic dystrophy in the child. This phenomenon is consistent with anticipation (i.e., the occurrence of progressively earlier onset and more severe disease in successive generations).
Molecular genetic studies have provided major insight into the reasons for anticipation and related aspects of congenital myotonic dystrophy ( Box 33.2 ). Thus myotonic dystrophy has been shown to be associated with an increase in the number of specific (CTG [cytosine-thymine-guanine]) trinucleotide repeats in an unstable DNA region of the 3′ untranslated region of the myotonic dystrophy gene, which is located at chromosome 19q13.3. Moreover, the number of repeats increases with maternal transmission of the gene and a correlation of the number of repeats (i.e., the extent of the so-called expansion of the triplet repeat region on chromosome 19) exists with severity and early onset of the disease in the infant. This molecular genetic phenomenon underlies anticipation. Current data suggest that paternal transmission of the unstable trinucleotide repeat is associated with reduced amplification or even contraction of the region of repeats, in contrast to the situation with maternal transmission. This feature of paternal transmission may be related to negative selection of sperm with large repeat numbers and may explain the exclusively maternal transmission of congenital myotonic dystrophy. At any rate, determination of the number of repeats from a DNA sample of a woman can be used to estimate the likelihood of transmitting severe congenital myotonic dystrophy, and, in an affected infant, such a determination can be used to estimate the likely severity of the disorder. However, correlations are not perfect, in part because of somatic mosaicism (i.e., the extent of repeat expansion varies from tissue to tissue, even in postmitotic tissue). Somatic mosaicism has been documented in congenital myotonic dystrophy, although it is less pronounced than in the adult form of the disease.
The disease is associated with an abnormally high number of CTG trinucleotide repeats, usually more than a thousand, in the 3′-untranslated region of the myotonic dystrophy protein kinase gene ( MDPK ) on chromosome 19q13.3.
The increased number of repeats correlates approximately with earlier onset and more severe disease.
The mutation is dynamic (i.e., does not remain of constant size within a pedigree or across generations).
Maternal transmission is associated with expansion (i.e., increased number) of the repeat region (1000–4000 repeats) and with more severe disease in the infant (i.e., anticipation ).
The extent of repeat expansion varies among tissues (i.e., somatic mosaicism ); thus determination of the number of repeats in DNA in blood or chorionic villus cannot predict severity of disease in muscle or other tissues with absolute accuracy.
Sequestration of multiple RNA- and DNA-binding proteins by the region of multiple repeats leads to dysregulation of splicing of pre-mRNAs, such as the insulin receptor and skeletal muscle chloride channel, resulting in insulin resistance and myotonia.
The gene affected in myotonic dystrophy (MDPK) encodes a serine-threonine protein kinase; myogenin, the beta-subunit of the L-type calcium channels, and phospholemman are the substrates for this enzyme, which appears to function normally in patients with DM1. Normal leukocyte DNA contains 5 to 37 copies of the CTG trinucleotide. In permutation carriers , the size of the unstable CTG array is 30 to 50 repeats; however, it varies between 50 and 4000 repeats in symptomatic individuals. Infants with congenital myotonic dystrophy are likely to have more than 1000 repeats in their leukocyte DNA, although a number of these patients may have CTG expansions of less than 1000 repeats. The features of congenital myotonic dystrophy appear to be related to a toxic RNA gain-of-function effect. Thus the trinucleotide repeats appear to bind and sequester multiple RNA- and DNA-binding proteins, such as the muscleblind-like (MBNL) protein family, thereby leading to multiple deleterious effects (see Box 33.2 ), including dysregulation of alternative splicing of pre-mRNAs. Sequestration of MBNL1 leads to increased synthesis of the less responsive neonatal isoform of the insulin receptor and overproduction of the neonatal isoform of the skeletal muscle chloride channel; this imbalance results in insulin resistance and a significant reduction in chloride conductance, causing myotonia.
The diagnosis is suspected if the mother has clinical or EMG features of DM1 or if she has a confirmed genetic diagnosis; the diagnosis is made in the neonate by identifying the abnormally expanded CTG repeat in the DMPK gene.
Management.
Management of the affected infant is, in considerable part, an ethical decision. For optimal development, adequate nutrition and ventilation must be ensured, and respiratory support and tube feedings may be needed for days to weeks. Survivors are usually able to suck and swallow adequately for oral nutrition by 8 to 12 weeks of age. When ventilatory support is required for more than 3 to 4 weeks, the mortality rate exceeds 25%. However, the use of nasal continuous positive airway pressure has facilitated weaning in affected infants after such prolonged mechanical ventilation. In infants with particularly poor gastric motility, metoclopramide, which decreases the smooth muscle threshold for the action of acetylcholine, may be especially useful.
Subsequent problems relate to both the muscle and central nervous system (CNS). Supportive measures for the myopathy are required. Most of the joint deformities can often be managed with a nonsurgical approach, although more aggressive management of the foot and ankle deformities may prevent gait disturbances.
Congenital Muscular Dystrophy
The term CMD refers to a group of disorders that share clinical and myopathological features. In older patients, the term muscular dystrophy has been used for a group of progressive, inherited disorders of muscle that share a striking myopathology. Although some of the reported cases of CMD do not exhibit definite progression and a familial nature cannot always be established, the disorder indeed does generally conform to an inherited involvement of muscle, progressing prenatally (and ultimately also postnatally) and sharing a myopathology similar to that of later-onset dystrophies.
The classification of CMDs has become particularly difficult because, in the past 20 years or so, largely through molecular genetic studies, increasing numbers of clinical phenotypes and responsible genes have been recognized. We have elected to use a fundamentally clinical categorization, and we focus on those disorders that may manifest in the neonatal period ( Table 33.2 ). We find it most useful to distinguish those CMD disorders that involve primarily muscle (i.e., without overt CNS abnormalities, such as those manifested by major neurological deficits) from those CMD disorders that are accompanied by overt CNS abnormalities (with such associated deficits as intellectual disability). Recently, however, CMDs have been classified using a combination of clinical, biochemical, pathological, and genetic features (see Table 33.2 ). Table 33.2 lists the genes mutated in various CMDs and the respective phenotypes. We focus on those disorders that are most likely to be associated with a clear neonatal presentation: primary, merosin-deficient CMD, and the three major CMDs with overt CNS abnormalities: Walker-Warburg syndrome (WWS), muscle-eye-brain disease (MEB), and Fukuyama CMD (FCMD). We describe only briefly those disorders that are particularly unusual or that do not generally result in marked neonatal features (i.e., Ullrich syndrome, congenital laminopathy, and rigid spine syndrome).
BIOCHEMICAL DEFECT | LOCUS | GENE | DISEASE PHENOTYPE(S) |
---|---|---|---|
Extracellular matrix proteins | |||
6q22-23 | LAMA2 | Primary merosin deficiency (MDC1A) | |
21q22.3 | COL6A1 | Ullrich CMD | |
2q37 | COL6A2 | ||
COL6A3 | |||
External sarcolemmal proteins | |||
12q13 | ITGA7 | Integrin α7 – related CMD | |
3p23-21 | ITGA9 | Integrin α9 – related CMD | |
Dystroglycan and glycosyltransferase enzymes | |||
9q34.1 | POMT1 | WWS, MEB, CMD with cerebellar involvement, CMD with intellectual disability and microcephaly | |
1q32-34 | POMGnT1 | WWS, MEB, CMD with cerebellar involvement | |
14q24.3 | POMT2 | WWS, MEB, CMD with cerebellar involvement, CMD with intellectual disability and microcephaly | |
19q13.3 | FKRP | WWS, MEB, CMD with cerebellar involvement, CMD with intellectual disability and microcephaly, CMD with no intellectual disability and normal brain MRI | |
9q31 | FCMD | Fukuyama CMD | |
22q12.3-13.1 | LARGE | WWS, MEB, white matter changes | |
1q12-q21 | DPM2/DPM3 | CMD with intellectual disability and severe epilepsy | |
7p21.2 | ISPD | WWS, LGMD | |
3p22.1 | GTDC2 | WWS | |
11q13.2 | B3GALNT2 | WWS, MEB | |
3p21.23 | GMPPB | CMD with intellectual disability and severe epilepsy, LGMD | |
3p21 | DAG1 | Primary dystroglycanopathy, LGMD with early onset and intellectual disability, normal brain MRI | |
8p11.21 | SGK196 | MEB | |
1q42 | — | MDC1B | |
Endoplasmic reticulum protein | |||
1p35-36 | SEPN1 | CMD with spinal rigidity (RSMD1) | |
Nuclear envelope proteins | |||
6q25 | SYNE1 (nesprin 1) | CMD with adducted thumbs | |
1q21.2 | LMNA | Congenital laminopathy | |
Sarcolemmal and mitochondrial membrane protein | |||
22q13 | CHKB | Mitochondrial CMD (CMDmt) | |
21q22.3 | COL6A1 COL6A2 | Ullrich CMD | |
2q37.3 | COL6A3 | Ullrich CMD |
a The classification is based on combined clinical, genetic, and pathological data.
Congenital Muscular Dystrophy Without Overt Structural or Functional Brain Abnormalities.
CMD caused by a primary deficiency of merosin (i.e., laminin alpha-2 chain; see later), MDC1A, accounts for approximately 20% to 30% of cases of CMD (see Table 33.2 ). In 1979, an initial series of five infants with CMD was reported with no clinical sign of CNS disease (except for one with seizures) but with marked hypodensity of cerebral white matter on CT. Over the next decade, subsequent reports of a similar relationship between CMD and abnormal cerebral white matter, primarily on CT scans, were published. With the discovery that this disorder is associated with a deficiency of a critical basal lamina component (i.e., the laminin alpha-2 chain), recognition of the relative frequency of this disorder increased dramatically. The clinical presentation with weakness and hypotonia in merosin-deficient CMD is more severe than that in other merosin-positive CMDs. Indeed, motor function usually does not evolve past sitting or standing with orthotic support; ambulation is hardly ever achieved ( Table 33.3 ). Contractures are common and involve the hips, knees, elbows, and ankles, although severe neonatal arthrogryposis is rare. Nevertheless, in our experience, merosin-deficient CMD and congenital myotonic dystrophy are the two most common muscle disorders leading to multiple congenital contractures. Weakness tends to affect the upper limbs more severely than the lower limbs. Facial weakness is often prominent. A typical clinical sign is limitation of eye movements, particularly of the upgaze, but this is often noted in the second decade of life. Disturbance of chewing and swallowing, with a tendency for aspiration, is very common; a feeding tube may be needed. As many as 20% to 30% die of cardiopulmonary complications in the first year or so of life. A less severe phenotype, associated with partial deficiency of merosin , has been reported. a
a References .
However, these patients usually have normal milestones in the first years of life and usually do not require enteral feeding and/or ventilator support.FEATURES | |
---|---|
Clinical severity | Marked |
Ambulation | Rare |
Creatine kinase elevation | Marked |
Myopathology | Severe |
Nerve conduction velocity | Slow |
White matter abnormality | Marked |
Structural brain abnormalities | ≈<10% |
Epilepsy | ≈20% |
Chromosomal locus | 6q2 |
Laminin alpha-2 (merosin) | Absent b |
b Partial but not total deficiency of laminin alpha-2 chain is associated with less severe phenotype (see text).
Despite the uniform occurrence of diffusely abnormal cerebral white matter by brain imaging in merosin-deficient CMD (see later discussion), clinical neurological abnormalities are unusual. Approximately 20% to 30% of patients develop seizure disorders, usually later in childhood, and approximately 5% to 10% of patients exhibit cognitive deficits.
Muscle biopsy shows dystrophic changes, and immunofluorescence with antimerosin antibodies demonstrates decreased or absent staining; in severe infantile cases, there is total absence or only traces of the laminin alpha-2 chain protein. Sometimes an extensive inflammatory infiltrate may be seen.
The diagnosis of merosin-deficient CMD is confirmed by detecting a recessive mutation(s) (homozygous or compound heterozygous state) in the laminin alpha-2 chain ( LAMA2 ) gene.
Partial merosin deficiency may occur in the setting of other CMDs, such as dystroglycanopathies. CMD with secondary deficiency of merosin is rare in the neonatal period and likely represents a heterogeneous disorder. Formerly, the best characterized of this group was CMD related to FKRP mutations (see later). However, the more recent recognition that this disorder may be associated with overt CNS abnormalities makes it more appropriate to discuss later.
Merosin-positive CMD encompasses multiple clinical phenotypes, but the three most likely to be encountered in the neonatal period are Ullrich syndrome, congenital laminopathy, and rigid spine syndrome (see Table 33.2 ). The term classic merosin-positive CMD has become obsolete as molecular genetic studies characterize distinct disorders.
Ullrich CMD is a type of merosin-positive CMD with a characteristic clinical phenotype. Neonatal presentation with hypotonia, weakness, proximal joint contractures, torticollis, hip dislocation, spine kyphosis, and marked distal hyperlaxity is characteristic. In older infants and children, other typical features include prominent calcanei, keratosis pilaris, and hypertrophic scars (keloids). Subsequent motor development is severely delayed, and walking independently occurs only in the minority. The defect involves the gene (COL6A) for collagen VI, an extracellular matrix protein. Most cases of neonatal onset are autosomal recessive. Collagen VI is composed of three alpha chains encoded by three genes: COLA1, COLA2 , and COLA3 .
Congenital laminopathy is another CMD (L-CMD) related to recessive or dominant de novo mutations in the lamin A/C gene ( LMNA ) ( Table 33.4 ). LMNA mutations cause the dominant form of Emery-Dreifuss muscular dystrophy. Early onset in infancy has been described with severe axial weakness, wasting of cervicoaxial muscles, limited spontaneous movement, and dropped head syndrome as well as cardiac arrhythmias and feeding and respiratory difficulties. Muscle biopsy usually shows a dystrophic pattern and sometimes inflammatory infiltrates.
CLINICAL FEATURE | TYPE OF CMD | ||
---|---|---|---|
FUKUYAMA | WALKER-WARBURG | MUSCLE-EYE-BRAIN | |
Neurological deficits | + | + | + |
Microcephaly | + | − | − |
Macrocephaly | − | + | + |
Hypotonia | + | + | + |
Ocular abnormalities (severe) | − | + | + |
White matter abnormality on neuroimaging (persistent or progressive) | + | + | − |
Lissencephaly-pachygyria | + | + | + |
Cortical mantle thin | ± | + | − |
Corpus callosum absent | ± | + | − |
Hydrocephalus | − | + | ± |
Encephalocele | − | + a | − |
Cerebellar malformation | − | + | ± |
a Characteristic when present but described in less than 50% of cases.
Rigid spine syndrome is a third merosin-positive CMD that usually presents clinically after the neonatal period with hypotonia and axial weakness. Neonatal onset has been reported. Subsequently, the characteristic spinal extensor contractures become apparent, with resulting spinal rigidity, scoliosis, and restrictive respiratory disease. The defect involves the gene (SEPN1) for an endoplasmic reticulum protein, selenoprotein N, the function of which is unknown.
Congenital Muscular Dystrophy With Overt Structural or Functional Brain Abnormalities.
CMD may be accompanied by prominent involvement of the CNS, manifested by severe neurological deficits (e.g., intellectual disability; see Table 33.2 ). Although primary merosin deficiency is associated with a morphological disturbance of cerebral white matter (see later), no clinical neurological abnormalities occur in most cases. Because these disorders are related to abnormalities of glycosylation of alpha-dystroglycan, they are often grouped as dystroglycanopathies . The term dystroglycanopathies includes a group of muscle diseases, with hypoglycosylation of alpha-dystroglycan on muscle biopsy, often associated with central nervous system structural abnormalities and less frequently with eye pathology. Some of the entities in this category are associated with disorders of neuronal migration, cerebral white matter abnormalities, hindbrain anomalies, and marked clinical neurological abnormalities; they are termed FCMD, WWS, and MEB. There are also mild dystroglycanopathies with late onset and no structural or functional brain or eye involvement; these are not discussed here. The Online Mendelian Inheritance in Man (OMIM) database subdivides the group of muscular dystrophies with deficit of dystroglycan glycosylation or muscular dystrophy-dystroglycanopathy (MDDG) into three broad phenotypical groups: A, B, and C. Primarily the type A severe phenotypes are discussed here.
FCMD (see Tables 33.2 and 33.4 ) has been described in several hundred Japanese children and in a much smaller number of children in North America, Europe, and Australia. The disorder exhibits the same basic clinical features referable to muscle involvement as the CMDs without CNS involvement: (1) onset in the neonatal period, (2) marked diffuse weakness and hypotonia, (3) facial weakness, and (4) joint contractures that develop particularly in infancy after the neonatal period. The clinical course of the motor function is generally characterized by slow acquisition of skills, usually the ability to crawl or to stand with support, until approximately the age of 8 years, when motor functions begin to deteriorate, ultimately to a lower level. The progression relates both to worsening of contractures and to apparent increasing weakness. Most patients become bedridden by the age of 10 years, and life expectancy is usually approximately 20 years.
The hallmark of FCMD is the evidence of major CNS disease. Intellectual disability is essentially constant, and the usual IQ level is between 30 and 50. a
a References .
Moreover, febrile and afebrile seizures occur in approximately 60% of the cases. CT and especially MRI scanning and neuropathological studies (see subsequent discussions of diagnosis and pathology) establish the causes of these neural phenomena (i.e., major neuronal migrational and other abnormalities).WWS is the more severe of the two CMDs, with prominent ocular as well as CNS abnormalities (see Tables 33.2 and 33.4 ; see Chapter 6 ). Indeed, the life expectancy in this disorder is less than 3 years. In the newborn, the muscle disorder is often overshadowed by the severe CNS (cobblestone lissencephaly, pontocerebellar hypoplasia, severe white matter abnormality) and ocular (microphthalmia, hypoplastic optic nerves, colobomata, retinal detachment) abnormalities. Severe disturbances of feeding and tube or gastrostomy feeding are nearly invariable.
MEB is a similar CMD, with ocular and CNS abnormalities, although it is milder than WWS (see Tables 33.2 and 33.4 ). Thus an initial report from Finland describes 18 affected patients with the clinical features referable to CMD, intellectual disability of variable severity in 15 of the 18 reported patients, occasional seizures, and, distinctively, a wide variety of ocular abnormalities. These ocular defects include high myopia, congenital or infantile glaucoma, hypoplasia of the retina and optic nerve, coloboma of the optic nerve, and cataracts. Many subsequent reports further delineate this type of CMD. b
b References .
Although patients with MEB that is overt at birth may exhibit severe abnormalities, in general the features of this disorder are milder than those observed with WWS, and both of these cerebro-ocular types of CMD exhibit differences from FCMD (see Table 33.4 ).
There is also a fourth group of CMD patients (OMIM classification type B) with posterior fossa/cerebellar abnormalities on imaging or a normal MRI; these patients may be intellectually normal or have an associated intellectual disability. In this group, cerebellar abnormalities may be the only structural brain defects; they include cerebellar cysts and hypoplasia or dysplasia. Albeit supratentorial defects may also occur, cerebellar cysts in the absence of supratentorial white matter or cortical involvement have been described mostly in patients with mutations in the fukutin-related protein (FKRP) gene. Although FKRP-related CMD usually manifests beyond the neonatal period and is not considered in detail, neonatal onset is well documented. Weakness and hypotonia are apparent in the first weeks or months of life, although a phenotype as severe as primary deficiency of merosin has been observed. Subsequent motor development is markedly impaired, and respiratory muscle weakness leading to ventilatory failure occurs in the first or second decade of life. In neonatal-onset cases, severe cognitive deficits are common. Muscle biopsy shows a partial deficiency of laminin alpha-2 protein; thus this disorder was previously classified as CMD with secondary deficiency of merosin. However, the principal defect involves FKRP, presumed to be a glycosyl transferase, with alpha-dystroglycan the likely substrate. More than half of 25 reported cases with FKRP mutations have had prominent CNS abnormalities and thus should be included with the CMD group with overt CNS abnormalities. The neurological abnormalities have included intellectual disability, and the CNS structural deficits have included subependymal heterotopias, focal pachygyria, white matter abnormalities, cerebellar cysts, marked cerebellar dysplasia, and pontine hypoplasia. These CNS deficits are consistent, at least in part, with neuronal migrational disturbance and overlap with those observed in FCMD, WWS, and MEB. This similarity is noteworthy because, as discussed earlier, the last three types of CMD with overt CNS abnormalities all also involve disturbances of glycosylation of alpha-dystroglycan.
Laboratory Studies.
When all types of CMD (see Table 33.2 ) are considered, the serum CK level is elevated in most cases, particularly early in the course of the disease. However, the presence and degree of elevation vary with the type of disorder. In the largest series of cases ( n = 50) of the now obsolete entity known as merosin-positive classic (pure) CMD , 54% of infants had elevated CK levels, and the average elevation was approximately three times the upper limit of normal. In another series of 24 cases, CK elevation was 2.5-fold above the normal upper limit. The important clinical point is that a normal CK level does not exclude the diagnosis. By contrast, in merosin-negative CMD , the CK levels are consistently and markedly elevated to approximately 10-fold to 20-fold higher than the upper limit of normal. In FCMD, CK levels generally range from 10-fold to 50-fold higher than the normal upper limit. Levels are similarly very high in MEB and in WWS. In all cases, CK levels tend to decline with the patient’s age. The EMG reveals the changes of myopathy (see Table 30.4 ), including small-amplitude, brief-duration, and polyphasic motor unit potentials.
CT and, preferably, MRI scans of the head are useful in identifying the cerebral white matter abnormality characteristic of primary merosin-deficient CMD ( Fig. 33.9 ). The appearance on CT is decreased attenuation of cerebral white matter and, on MRI, increased signal on T2-weighted images. The cerebral white matter is affected diffusely, whereas the cerebellar white matter is unaffected. The cerebral white matter abnormality is generally not apparent in the first weeks of life and becomes apparent by approximately 6 months of age (see Fig. 33.9 ). However, utilization of T2-weighted images with a fast spin-echo sequence has demonstrated the white matter abnormality at 5 days of age. The abnormal white matter signal is most prominent in structures myelinated after birth (e.g., cerebral white matter) rather than in those structures that are myelinated before birth (e.g., brain stem and cerebellar white matter). Thus the abnormality may reflect a delay or arrest in myelination, and the finding in the affected white matter of abnormally increased apparent diffusion coefficients, characteristic of immature white matter, is perhaps consistent with this notion. Although the cerebral white matter abnormality is the unifying feature of the primary merosin-negative cases, several reports document the occurrence of cortical gyral dysplasia, especially in the occipital region, in isolated cases (~ <10%). Hypoplasia of the cerebellum was observed by MRI to varying degrees in 6 of 14 well-studied cases, and associated cerebellar cysts have also been described.

In FCMD , the most striking abnormality is the gyral disturbance ( Fig. 33.10 ). The two major findings detectable by MRI are as follows: (1) a thick cortex with shallow sulci in the frontal regions, consistent with polymicrogyria, and (2) a thick cortex with a smooth surface in the temporo-occipital regions, consistent with lissencephaly-pachygyria. These findings correlate well with the neuropathology (see later discussion). Additional consistent features are white matter changes similar to those described earlier for merosin-deficient CMD. However, distinction of these two entities by MRI is straightforward because of the marked gyral abnormalities in FCMD. Moreover, the white matter abnormality in the latter diminishes with age. The corpus callosum is slightly hypoplastic in most of the Fukuyama cases.

In WWS and MEB , the dominant finding is the gyral abnormality. In its full form, as in WWS, the appearance of type II lissencephaly ( cobblestone lissencephaly) and pachygyria is striking and is associated with diffuse white matter abnormality of the type observed in merosin-deficient CMD. Again, the distinction by MRI from the latter disorder is straightforward because of the presence of the gyral disturbance as well as marked dysgenesis of cerebellum and hypoplasia of the pons, especially in WWS.
Additional laboratory studies of interest include measurements of cerebral visual and somatosensory evoked responses and of peripheral nerve conduction velocities in merosin-deficient CMD (see Table 33.3 ). Thus, for somatosensory evoked responses, latencies have been delayed in all patients studied, and for visual evoked responses, latencies have been delayed in approximately 90% of patients. Nerve conduction velocity studies have shown moderately slow values in more than 80% of merosin-deficient cases studied thus far. One infant studied in the newborn period had normal values but exhibited slowed conduction when studied again at 6 months of age. No abnormalities in cerebral evoked responses or motor nerve conduction velocities have been observed in merosin-positive CMD.
The demonstration of laminin alpha-2 deficiency in skin biopsy and in cultured fibroblasts in primary merosin-deficient cases indicates the potential value of this approach for the diagnosis of merosin-deficient CMD. In addition, the prenatal demonstration of merosin deficiency in trophoblastic tissue obtained from chorionic villus samples indicates the value of this approach (with simultaneous linkage analysis) in prenatal diagnosis.
Pathology.
Muscle biopsy in all types of CMDs shows striking changes, consistent with a dystrophic process. Notable are marked variations in fiber size in a nongrouped distribution, internal nuclei, and marked replacement of muscle by fat and connective tissue ( Fig. 33.11 ). a
a References .
Necrosis of fibers and evidence of regeneration are common. Striking changes not apparent on initial biopsies may appear on subsequent biopsies. The fiber-type pattern is retained; the muscle does not exhibit the prominent signs of maturational disturbance observed in myotonic dystrophy (see earlier discussion). Although comparisons are difficult, the myopathic changes reported in primary merosin-deficient CMD are more striking than those in merosin-positive CMD. Several reports have emphasized the presence of inflammatory cellular infiltrates, especially early in the disease and perhaps related to necrosis ; this finding should not lead to the mistaken diagnosis of infantile polymyositis, a treatable and self-limited disorder (see later discussion). The diagnostic feature critical for merosin-deficient CMD is the lack of staining for this molecule on immunocytochemical study ( Fig. 33.12 ). Partial absence is observed in the infants with the less severe, partial type of merosin-deficient CMD or in secondary merosin deficiencies. aa References .


The neuropathology in infants with the merosin-deficient CMD with cerebral white matter abnormalities is not clearly known. The little neuropathological information available is inconclusive. The findings that the white matter abnormality becomes most apparent later in infancy and is associated with disturbances of visual and somatosensory evoked potentials and the MRI findings suggesting a defect in postnatal myelin development (see earlier discussions) suggest that myelination is altered in some way. Laminin alpha-2 chain is expressed in the basement membrane of blood vessels that form the blood-brain barrier and also along developing axonal tracts, where interaction with oligodendrocytes may occur. Disturbances at either or both loci could lead to disturbed myelin development and the abnormal signal with increased water diffusion on MRI or the edema by MR spectroscopy.
The neuropathology in infants with FCMD is striking. a
a References .
The dominant finding is cerebral gyral abnormality, most consistently polymicrogyria ( Fig. 33.13A ), pachygyria, or agyria (type II lissencephaly). The disorder indicates a disturbance of neuronal migration, as described in Chapter 2 . Other neuropathological abnormalities include subpial neuronal – glial heterotopias, cerebellar polymicrogyria (see Fig. 33.13B ), aberrant myelinated fascicles over the surface of the cerebellum, hypoplasia and aberrant course of the pyramidal tracts, leptomeningeal thickening, and occasional hydrocephalus. No distinct changes have been described in cerebral white matter except for a delay in myelination; hence the basis for the white matter abnormality detected by CT or MRI is unclear (see earlier discussion).
The neuropathology in the two CMDs with prominent ocular and brain abnormalities has been described. b
b References .
The findings bear similarities to those described in FCMD, including the following: type II lissencephaly; polymicrogyria, pachygyria, and heterotopias in the basal meninges; hypoplasia of the pyramidal tracts; and aqueductal stenosis. Distinguishing features in the more severe form of cerebro-ocular CMD, WWS, include more severe cortical migrational disturbances, hydrocephalus, cerebellar dysplasia-hypoplasia (including absence of vermis), and encephalocele (see Table 33.4 ). In general the findings in MEB are milder than in WWS.Pathogenesis and Etiology.
The pathogenesis and etiology of this group of disorders focus on the sarcolemmal membrane and the related proteins that link the extracellular matrix to the actin filaments of the myocytic cytoskeleton. c
c References .
The key sarcolemmal protein involved in linking to the extracellular matrix is alpha-dystroglycan; it interacts with laminin, the key extracellular matrix protein, which, in turn, interacts with collagen type VI. The intracellular connection is mediated by the interaction of alpha-dystroglycan with sarcoglycans, the sarcolemmal proteins, which are linked to dystrophin and thereby to the actin cytoskeleton ( Fig. 33.14 ). In the CMDs, the key proteins affected are alpha-dystroglycan , laminin , and collagen type VI , defects of which interrupt the vital relationship between the extracellular matrix and the muscle cytoskeleton and result in the muscle degeneration of CMD.
The molecular basis of primary merosin-deficient CMD is well established. Laminin-2 or merosin is the critical alpha-dystroglycan – binding basement membrane protein of the muscle extracellular matrix. The critical laminin alpha-2 chain of laminin 2 is encoded by the LAMA2 gene on chromosome 6q2; this is the gene that is defective in merosin-deficient CMD. Laminin alpha-2 chain is also present in basement membrane of Schwann cells, and thus its lack may underlie the defect of peripheral nerve described earlier. Moreover, as noted earlier, laminin alpha-2 is present in blood vessels and along developing axons in human brain (see earlier). How these observations relate to the cerebral white matter abnormality remains to be clearly elucidated. Demonstration of the deficient protein in muscle and skin facilitates diagnosis in the infant, and demonstration of the defect in chorionic villus samples facilitates prenatal diagnosis.
The molecular basis of classic (pure) merosin-positive CMD is heterogeneous and reflects the nature of the underlying genetic defects.
As discussed earlier, the molecular basis of Ullrich CMD involves the three genes (COL6A1, COL6A2, COL6A3) for collagen VI (see earlier). This defect results in a prominent loss of the basal lamina of muscle fibers and interrupts the vital link between the extracellular space and the intracellular cytoskeleton, as described earlier.
The molecular basis of rigid spine syndrome involves a deficiency of the gene (SEPN1) selenoprotein N, an endoplasmic reticulum protein (see earlier). The function of this protein remains elusive; however, it may be involved in protecting cells against oxidative stress.
The molecular bases for the CMDs with overt CNS abnormalities (i.e., FCMD, WWS, MEB and others), the dystroglycanopathies, all involve defects in dystroglycan and multiple glycosyl transferase genes catalyzing the O -linked glycosylation of alpha-dystroglycan (see Table 33.2 ). a
a References .
POMT1 encodes the protein O -mannosyl transferase; POMGnT1 encodes the protein O -mannosyl- N -acetylglucosaminyl transferase. Multiple O -linked glycosylation sites are present in certain serine-threonine – rich domains of alpha-dystroglycan. FCMD is caused by a defect in a gene (FCMD) that encodes fukutin (FKTN), a putative glycosyltransferase, the exact function of which is yet unclear.Mutations in the FKTN , FKRP , and ISPD genes can result in severe WWS or MEB phenotypes, in intermediate phenotypes such as those associated with cerebellar cysts or normal brain MRI, or even in milder limb-girdle muscular dystrophies. Although the mutated gene cannot be predicted from the clinical phenotype, there are certain features that may suggest a particular gene or number of genes.
- •
In WWS, although POMT1 was the first genetic defect to be described, mutations in all known dystroglycanopathy genes have been described.
- •
In MEB, mutations have been identified in POMGnT1 (the first linked to MEB), POMT1, POMT2, ISPD, and FKRP.
- •
Cerebellar cysts have been described in association with mutations in FKRP, POMGnT1, and ISPD genes but may rarely also be seen with POMT1 and POMT2 mutations.
Disturbances in the glycosylation of alpha-dystroglycan impair its interaction with laminin and sarcolemmal proteins, especially beta-dystroglycan. Muscle degeneration is the result. Because alpha-dystroglycan is also present in the basement membrane of the glia limitans in the developing cerebral cortex, defective glycosylation results in the disturbances in neuronal migration that underlie the occurrence of cobblestone lissencephaly (see Chapter 6 ). As noted earlier, more than half of the cases of CMD related to FKRP mutations and FKRP deficiency, which exhibit CNS abnormalities milder but mechanistically similar to those of the three main disorders discussed here (WWS, MEB, FCMD), are relevant in this context, because the FKRP also appears to be a glycosyltransferase for alpha-dystroglycan.
Management.
Because the course in most patients with any of the types of CMD is nonprogressive for months to years, it is important to attempt to correct existing contractures by physical therapy (i.e., passive stretching or serial splints) and to prevent the development of contractures by active and passive exercises. The development of fixed deformities is often more deleterious to future motor abilities than is muscle weakness. Although no specific therapies are available, more details concerning supportive management are available in specialized sources.
Facioscapulohumeral Dystrophy
FSHD is usually recognized as an autosomal dominantly inherited myopathy with prominent involvement of the face and upper extremities, onset in adolescence or early adulthood, and a slowly progressive or apparently static course. A rare type of FSHD with onset in early infancy and a more severe course have been delineated.
Clinical Features.
The infantile clinical syndrome , first described by Carroll and Brooke in 1979, is characterized by the onset of facial weakness in early infancy. The weakness is distinctive in that most patients are unable to move the upper lip and later have a peculiar horizontal smile. Difficulty in closing the eyes is prominent, and the infant may sleep with the eyes open. The whole face tends to be smooth and expressionless. Difficulty in sucking is common. However, motor milestones are usually not significantly delayed, and 8 of 11 patients in the largest series walked alone by 15 months of age. Later, in infancy and early childhood, progressive weakness and wasting of axial and limb muscles supervene, particularly in the proximal upper extremities but then also in the lower ones. Of five patients who had reached 15 years of age or more, four were no longer walking, and the remaining patient was walking with difficulty. A similarly progressive disease course has been documented by other investigators. In a report of seven infantile-onset cases, the age at onset was between infancy and 4.5 years and the mean duration between onset of first symptoms and wheelchair dependency was 9.9 years. In a recently reported series of patients enrolled in the Italian FSHD registry, 54.5% showed clinical signs during the first 10 years; however, no patients had perinatal onset. The other clinical accompaniment, observed in the series of Carroll and Brooke, was sensorineural hearing loss in 6 of 11 children; this loss was severe in 2 children. Approximately 50% of subsequently reported cases have exhibited sensorineural hearing loss. Later in childhood, some patients have developed retinal telangiectasia, exudation, and detachment (Coats syndrome) in addition to sensorineural hearing loss. Intellectual disability was observed in 30% of cases in one series ; it was also documented with epilepsy in other cases.
Pathology.
Muscle pathology was striking in six of the nine patients studied in detail by Carroll and Brooke, and it exhibited an inflammatory response suggestive of polymyositis ( Fig. 33.15 ). Inflammatory changes in FSHD have been noted by others. a
a References .
This finding may lead to treatment with steroids, which results in no objective benefit. Indeed, it is clear from the relative frequencies of infantile FSHD and of polymyositis (see later) that inflammatory changes in muscle in an infant should raise the question of FSHD (or other congenital myopathy) rather than polymyositis, especially if the face is particularly involved.
Pathogenesis and Etiology.
The genetic abnormality in this dominantly inherited disease is located at chromosome 4q35. The frequency of de novo mutations is high. However, on careful examination, as many as half of the apparently sporadic cases were found to have an affected parent. Frequently parents are carriers with somatic mosaicism of the mutation and thus may appear unaffected. The defect is particularly interesting and involves a reduction in the number of repeats of a 3.3-kb repeat sequence, D4Z4, at the 4q35 locus. Normal individuals carry 11 or more D4Z4 repeats; patients with FSHD have 1 to 10 copies. There is an inverse and coarse relationship between the onset and severity of the disease and the size of the D4Z4 repeat array. Most patients with 1 to 3 D4Z4 units are usually severely affected with seizures, cognitive delay, and hearing loss and are typically sporadic cases. However, only about half of the contractions are pathogenic, because the array needs to be on an FSHD-permissive chromosome 4 that contains a polyadenylation signal for DUX4 . This gene is embedded within each D4Z4 repeat and encodes a germline transcription factor. In somatic cells, the D4Z4 repeat is epigenetically repressed, which results in silencing of the DUX4 gene. When the repeat contracts in FSHD1 patients, the epigenetic silencing is released, causing the expression of the DUX4 gene and protein, which is toxic to mature skeletal muscle. Normal-sized D4Z4 repeat arrays can also be depressed by heterozygous mutations in the structural maintenance of chromosomes flexible hinge domain containing protein 1 (SMCHD1) gene on chromosome 18, which encodes for a chromatin modifier; this explains the pathogenesis of FSHD2, in which the D4Z4 repeat is of normal size. SMCHD1 mutations in patients with FSHD1-sized D4Z4 repeat arrays of 9 to 10 units cause a more severe phenotype ( Fig. 33.16 ).

Laboratory Studies.
The serum CK level is moderately elevated in nearly all patients. The EMG reveals low-amplitude and short-duration motor unit potentials, indicative of myopathy. Nerve conduction studies are normal. However, these tests have become obsolete because, in suspected cases, the diagnosis can be confirmed by examining the leukocyte DNA for the D4Z4 repeat contraction and SMCHD1 mutations.
Management.
Management is similar to that for progressive myopathy in late childhood and adolescence. Of importance for the physician evaluating the newborn with facial weakness is the recognition that FSHD may be present; family members must be examined and perhaps have their leukocyte DNA evaluated. The parents should be counseled that, although the disorder in the affected parent is mild, a distinct possibility exists of a more severe form in the child (i.e., the phenomenon of anticipation). a
a References .
Polymyositis
Previously reported in infants no younger than 3 months of age, polymyositis was described in two newborns in 1982. The newborns exhibited marked hypotonia and weakness, weak cry, and poor sucking and feeding ability. Neck flexors were affected markedly, as in older patients with polymyositis. Particularly striking were markedly elevated levels of creatine phosphokinase (CK; 2500 and 3600 mU/mL) and muscle biopsy findings that included lymphocytic infiltration as well as myopathic changes. Improvement in overall strength and a decrease in CK levels followed treatment with steroids at 6 and 15 months of age. The observations suggested that polymyositis may occur in the newborn, that the diagnosis should be suspected with the findings of markedly elevated CK levels and inflammatory cells in the muscle biopsy specimens, and that treatment with steroids may be beneficial. Subsequent reports supported these general conclusions. The myopathic changes are consistent with an inflammatory myopathy with an immunological basis ( Fig. 33.17 ). The finding of perifascicular myopathy, generally considered a feature of such autoimmune myopathies as dermatomyositis, is particularly characteristic. It is important to rule out other neonatal myopathic disorders with inflammatory changes (e.g., FSHD, merosin-deficient CMD, or infantile-onset LMNA-associated myopathy), but other features of these latter disorders should make this distinction possible (see earlier discussions).

Minimal Change Myopathy
Minimal change myopathy , or nonspecific congenital myopathy , is the designation used for those infants with congenital hypotonia and weakness who exhibit minor (or even no) abnormalities of serum enzyme levels, EMG, or muscle biopsy. Myopathic illness is inferred from the clinical features (e.g., proximal more than distal weakness, preserved tendon reflexes, normal sensation, and absence of fasciculations) as well as from the occasional myopathic changes observed on the EMG (e.g., small-amplitude, brief duration, and polyphasic motor unit potentials) and abnormal muscle biopsy (e.g., modest variation in fiber size). The clinical features are not as prominent as those observed in more common myopathies (e.g., congenital myotonic dystrophy) and in most cases of CMD. Many of these infants have good prognoses and improve over time. Considering the lack of pathological markers, the molecular basis for most of these myopathies may become easier to delineate with the use of extensive panel-based genetic testing. Therefore this category will become increasingly less common.
Myopathic Disorders With Diagnostic Histology
The histology-diagnostic myopathic disorders are characterized by histological changes so striking and distinctive that a specific diagnosis can usually be made. An enormous literature has developed in this area since the 1970s, and several books and reviews deal with the details and complexities of the problem. Because most of the designations for these disorders are derived from histological and not clinical criteria, the clinical syndromes associated with each histological change vary. Moreover, considerable overlap exists among the disorders on both clinical and histological grounds. Nevertheless, certain specific congenital myopathies are well defined clinically, morphologically, and genetically, and several conclusions relevant to the neonatal patient can be drawn.
In the following discussion, we emphasize the most common features of each disorder, particularly those disorders with prominent clinical manifestations in the neonatal period. Of the seven categories listed in Box 33.1 , the first five refer to the disorders with morphologically distinctive changes by standard histological and histochemical techniques (i.e., specific congenital myopathies ) ( Fig. 33.18 ). Certain features are commonly observed among these disorders ( Box 33.3 ). Central core disease (CCD), nemaline myopathy, myotubular myopathy, and congenital fiber-type disproportion are emphasized because, albeit uncommon, they are the most common in this category. Multiminicore myopathy is rare in the newborn and is described only briefly. The mitochondrial myopathies may be suspected by standard histological techniques, but electron microscopic studies are needed to define the distinctive mitochondrial abnormalities if present. Biochemical studies may identify a specific abnormality of a mitochondrial enzyme. The metabolic myopathies may show no distinctive morphological change; thus one could argue that these myopathies should be classified as histology not diagnostic . However, they are included here because the biopsy specimen may indicate glycogen or lipid deposition in muscle, which is usually the critical initial finding in the definition of the specific biochemical lesion.

Inheritance: autosomal dominant > recessive > X-linked recessive
Onset in neonatal period
Recognition often after the neonatal period
Hypotonia
Weakness: proximal > distal
Tendon reflexes decreased in proportion to weakness
Muscle enzymes: normal or mildly/slightly elevated
Electromyogram: myopathic or normal
Muscle biopsy: diagnostic
Genetic testing: may be diagnostic
Core Myopathies
In core myopathies, the defining pathological feature is the presence of large regions devoid of oxidative staining in the center of myofibers (in CCD) or the presence of multiple smaller minicores (in multiminicore disease). Central cores or minicores may be seen in association with other pathological findings, such as nemaline rods, in the so-called core-rod myopathies often associated with mutations in various genes, including RYR1 ( Table 33.5 ).
SUBTYPE | GENE | OMIM DESIGNATION | CHROMOSOME LOCATION | INHERITANCE PATTERN |
---|---|---|---|---|
Nemaline myopathy | ACTA1 | NEM3 | 1q42.1 | AD, AR |
CFL2 | NEM7 | 14q13.1 | AR | |
KBTBD13 | NEM6 | 15q22.31 | AD | |
KLHL40 | NEM8 | 3p33.1 | AR | |
KLHL41 | NEM9 | 2q31.1 | AR | |
NEB | NEM2 | 2q23.3 | AR | |
RYR1 | 19q13.2 | AR | ||
TNNT1 | NEM5 | 19q13.42 | AR | |
TPM2 | NEM4 | 9p13.3 | AD | |
TPM3 | NEM1 | 1q21.3 | AD, AR | |
LMOD3 | NEM10 | 3p14.1 | AR | |
Cap disease (NM variant) | ACTA1 | AD | ||
TPM2 | AD | |||
TPM3 | AD | |||
Zebra body myopathy | ACTA1 | AD | ||
Hyaline body myopathy (myosin storage myopathy) | MYH7 | 14q11.2 | AD | |
Core-rod myopathy | KBTBD13 | AD | ||
NEB RYR1 | AR AD, AR | |||
TPM2 | AD | |||
Central core disease | RYR1 | CCD | 19q13.2 | AD, AR |
Multiminicore disease | RYR1 | AR | ||
SEPN1 | 1p36.11 | AR | ||
MYH7 | AD | |||
Centronuclear myopathy | BIN1 | CNM2 | 2q14.3 | AR |
CCDC78 | CNM4 | 16p13.3 | AD | |
DNM2 | CNM1 | 19p13.2 | AD | |
MTM1 | CNMX | Xq28 | XL | |
MYF6 | CNM3 | 12q21.31 | AD | |
RYR1 | 19q13.2 | AR | ||
TTN | 2q31.2 | AR | ||
Congenital fiber-type disproportion | ACTA1 | AD | ||
MYH7 | AD | |||
RYR1 | AR | |||
SEPN1 | AR | |||
TPM2 | AD | |||
TPM3 | AD | |||
Autophagic vacuolar myopathies | VMA21 | MEAX | Xq28 | XL |
LAMP2 | Danon disease | Xq24 | XL | |
GAA | Pompe disease | 17q25.3 | AR | |
Myofibrillar myopathies | DES | MFM1 | 2q35 | AD, AR |
CRYAB | MFM2 | 11q23.1 | AD | |
MYOT | MFM3 | 5q31.2 | AD | |
ZASP | MFM4 | 10q23.2 | AD | |
FLNC | MFM5 | 7q32.1 | AD | |
BAG3 | MFM6 | 10q26.11 | AD |
Central Core Disease.
Central core disease , originally described by Shy and Magee in 1956, was the first of the specific congenital myopathies to be defined. Subsequent reports further delineated a fairly discrete clinical syndrome.
Clinical Features.
The clinical syndrome consists of hypotonia and weakness, apparent usually in the neonatal period. However, many infants are not recognized as exhibiting muscle disease until months later. Muscle weakness is usually more prominent in the lower limbs and the proximal muscles ( Fig. 33.19A ), and tendon reflexes are usually preserved, although diminished or absent in proportion to the weakness. Congenital hip dislocation is also common. Mild facial weakness is not unusual. Ptosis, extraocular muscle weakness, dysphagia, and respiratory difficulties have not been features. However, severe neonatal weakness, arthrogryposis, and respiratory failure have been described in a subset of infants with CCD.

With the exception of the last subgroup of infants with severe neonatal presentation who do not walk independently and continue to require respiratory support, the course of the disease is usually nonprogressive, and infants attain motor milestones albeit at a slow rate. Slow progression of weakness may occur, but even without this progression, contractures, pes cavus or planus, and kyphoscoliosis may result. In a series of 11 patients followed to the age of 4 to 20 years, 2 were unable to walk alone and 2 had difficulty climbing stairs. An increased risk of malignant hyperthermia (e.g., during anesthesia) must be recognized to avoid unexpected death.
Laboratory Studies.
Laboratory investigations , aside from muscle biopsy and genetic testing, are not particularly helpful. The serum CK level is usually normal or slightly raised, and the EMG is usually normal or shows myopathic changes, although frequently minor.
Pathology.
Muscle pathology is distinctive and diagnostic in the presence of the clinical findings. Many muscle fibers (20%–100%) exhibit cores that are central or somewhat eccentric in location (see Fig. 33.19B ). Single or multiple cores may be observed in a given fiber and are well demonstrated with the histochemical stains for oxidative enzymes (e.g., reduced nicotinamide adenine dinucleotide-tetrazolium reductase [NADH-TR] or succinic dehydrogenase [SDH]), which are absent in the core region. Indeed, electron microscopy shows an absence of mitochondria and sarcoplasmic reticulum in the core region. The cores contain densely packed and disorganized myofibrils. They have a particular predilection for type I fibers and, in some cases, type I fibers predominate or are the only fiber type observable.
Pathogenesis and Etiology.
The pathogenesis relates to a genetic defect that is usually inherited in an autosomal dominant manner, although rarely autosomal recessive inheritance has been documented. The gene involved is RYR1 , which is located on chromosome 19q13.2 and encodes the skeletal muscle ryanodine receptor (see Table 33.5 ). The latter is a ligand-related release channel for internally stored calcium and thereby plays a crucial role in excitation-contraction coupling. More than 90% of CCD patients have mutations in RYR1 , which are most commonly dominant or de novo dominant; de novo dominant and autosomal recessive mutations are more common in the severe cases.
Management.
Management is the same as that of nonprogressive or slowly progressive myopathy. The prevention of contractures is important.
Multiminicore Disease.
Multiminicore disease (MmD) should be discussed briefly in this context of core myopathies. The classic form of MmD typically has its clinical onset in the neonatal period or early infancy. Neonatal hypotonia and weakness, although generalized, tend to predominate in the axial muscles. Mild facial weakness is common. Respiratory difficulties generally appear later, as do spinal abnormalities, especially scoliosis. Some patients with MmD become ventilator-dependent while still ambulant. Motor development is prominently delayed. The clinical course is static in most patients, although approximately 20% of patients exhibit mild progression. The pathology is distinctive and consists of the occurrence of multifocal core-like areas, best seen as areas of reduced activity on oxidative enzyme stains ( Fig. 33.20 ).

Pathogenesis is likely heterogeneous, with primarily sporadic and autosomal recessive cases reported. In separate cohorts, defects in the SEPN1 gene, the gene mutated in rigid spine syndrome (see discussion of CMD earlier) and in some later-onset forms of congenital fiber-type disproportion, and in the RYR1 gene, the gene mutated in CCD, have been identified. In addition, mutations in the beta-myosin heavy chain gene ( MYH7 ) and the giant sarcomeric protein titin (TTN) have been described in patients with MmD (see Table 33.5 ). The clinical overlap with CMD with rigid spine syndrome and the histological overlap with CCD are consistent with these initial genetic findings.
Nemaline Myopathy
Nemaline (rod body) myopathy , described initially by Shy and colleagues in 1963, was the second of the specific congenital myopathies to be defined. The term nemaline is derived from the Greek word nema , meaning “thread.” Numerous subsequent reports emphasize a considerable variation in clinical expression.
Clinical Features.
The clinical presentation in infants with manifestations from the neonatal period can be divided into three syndromes. First, as in typical CCD, hypotonia and weakness are relatively mild and are sometimes even overlooked in the newborn period. This variety is termed the typical congenital or classical form of nemaline myopathy . Second, marked neonatal hypotonia and weakness occur with no spontaneous movements or respiration at birth. Severe contractures or arthrogryposis and sometimes fractures complete this syndrome of severe congenital nemaline myopathy. Cardiac involvement in the form of dilated or hypertrophic cardiomyopathy may occur rarely, but in general cardiac contractility is normal. The pregnancy may be complicated by decreased fetal movements and polyhydramnios, and death in utero associated with fetal akinesia may occur. Third, marked neonatal hypotonia and weakness occur, but with some movement and respiratory effort and no severe contractures. This serious disorder, although not so marked as the severe cases, is termed intermediate congenital nemaline myopathy . In one large series of nemaline myopathy ( n = 143), approximately 50% of patients presented in the neonatal period; of these, 35% had the severe congenital form, 45% had the intermediate congenital form, and 20% had the typical congenital form. Accompanying the severe and intermediate forms were marked facial diplegia and feeding disturbances. The milder, typical form is associated with varying degrees of facial diparesis and feeding difficulties as well as distal involvement in addition to the proximal muscle weakness. Various skeletal anomalies—including high-arched palate, long dysmorphic face, prognathism malocclusion, pectus excavatum, and pigeon chest —may be seen. In surviving infants, pes cavus, kyphoscoliosis, and lumbar lordosis evolve.
The course of the typical, milder nemaline myopathy is generally considered to be nonprogressive or slowly progressive. However, in a study with a 5- to 25-year follow-up, 10 of 12 patients exhibited clinical deterioration, and only 8 of 12 were walking unsupported. Ten of 12 patients had developed scoliosis. Similar findings with 3- to 24-year follow-up were reported in a later study.
The course in those infants with the two more severe forms of the disease (i.e., the severe and intermediate forms) is generally unfavorable. Thus, in one series, 74% of patients with severe congenital disease died, generally before 1 year of age, and 28% of patients with intermediate congenital disease died. Among the infants with the severe form, a requirement for ventilation at birth or in the first month was followed by death in more than 90% of cases. Such infants fail to establish adequate ventilation after birth because of their weak respiratory muscles. This weakness results in failure of full expansion of the lungs, and aspiration is often added secondary to difficulty with secretions. Infants usually die in the neonatal period or in the first months of life. However, long-term survival in these patients, who are usually bedridden and receiving mechanical ventilation, has been observed. Moreover, rare affected infants with the need for mechanical ventilation at birth, severe weakness, and markedly impaired feeding have improved over the ensuing 2 to 3 years to become independent of mechanical ventilation and to achieve the ability to stand or even walk.
Laboratory Studies.
Laboratory investigations have included either normal or slightly elevated values for the serum CK level. The EMG may be normal but often exhibits short-duration and small-amplitude potentials of myopathy; signs of denervation have also been observed, particularly late in the disease, a
a References .
but nerve conduction studies are normal. Ultrasonography usually shows increased echogenicity in affected muscles. MRI reveals fatty infiltration of muscle tissue and can be valuable in the selection of a muscle to biopsy.Pathology.
Muscle pathology is distinctive and diagnostic in the presence of the clinical features. Although the rod bodies are readily overlooked on routine hematoxylin and eosin staining, Gomori trichrome, toluidine blue, and other selected stains demonstrate them well ( Fig. 33.21 ). Predominance of type I fibers, which are also small, is consistent. b
b References .

The rod body is derived from lateral expansion of the Z-disk, the band found normally at either end of the sarcomere. c
c References .
Thus the rod bodies have been shown by electron microscopy to be in structural continuity with the Z-disk ( Fig. 33.22 ). The rod’s major protein component is alpha-actin, the main protein of the Z-disk, and the bodies are penetrated by actin filaments, which normally penetrate the Z-disk. The rods are surrounded by desmin, the structural component of muscle-specific intermediate filaments. A combination of cores and rods has been reported in certain patients (core-rod myopathy). Intranuclear rods have been detected in some severe fatal neonatal cases ( Fig. 33.23 ). In general the severity of the myopathological features correlates only imperfectly with the clinical course. However, in nebulin-related nemaline myopathy a correlation between the severity of the disease and genotype has been reported.

Pathogenesis and Etiology.
The pathogenesis of nemaline myopathy relates to defects in one of 11 genes, 7 of which encode proteins of skeletal muscle thin filaments (see Table 33.5 ). Approximately 60% to 75% of cases of severe congenital nemaline myopathy are related to defects of ACTA1 , the gene for alpha-actin. Most of the remaining cases are related to nebulin, KLHL40, KLHL41, and leiomodin-3 defects and are principally autosomal recessive. Mutations in the genes for alpha-tropomyosin, beta-tropomyosin, and troponin are rare causes of severe disease. KLHL40 and KLHL41, which belong to the kelch protein family, are thought to be involved in ubiquitination and protein degradation, while leiomodin-3 is considered essential for the organization of sarcomeric thin filaments ( Fig. 33.24 ).

Management.
Management of the typical nonprogressive or slowly progressive form of nemaline myopathy depends principally on physical therapy and related techniques. However, respiratory failure may occur during infancy, rapidly and unexpectedly, with a fatal outcome, even in apparently stable or improving patients. a
a References .
Thus, careful follow-up is necessary. Infants with the severe neonatal form require prompt recognition and vigorous ventilatory and nutritional support if they are to survive. However, on the basis of the data available, appreciable improvement, despite diligent supportive care, is unlikely.Myotubular Myopathy
Myotubular myopathy , described initially in 1966 by Spiro and co-workers, was the third specific congenital myopathy to be described. The name is derived from the morphological appearance of the affected muscle fibers, which resemble fetal myotubes. Subsequent reports describe considerable variation in clinical expression. a
a References .
Clinical Features.
The clinical features of myotubular myopathy can be divided roughly into two syndromes. Less commonly, hypotonia and weakness are relatively mild and are sometimes overlooked in the newborn period. These autosomal varieties, also known as centronuclear myopathies, generally do not manifest frequently in the neonatal period. The more common disorder affects male infants and is characterized by marked hypotonia and weakness with respiratory failure. Many examples of this severe, malignant, X-linked neonatal form of myotubular myopathy (XLMTM) have been described. b
b References .
Polyhydramnios and decreased movement are noted in 50% to 60% of these fetuses during pregnancy. Failure to breathe effectively at birth often leads to asphyxia (and the mistaken diagnosis of hypoxic-ischemic encephalopathy for the subsequent motor deficits), and striking impairments of neonatal axial, appendicular, and facial movements well as sucking and breathing are apparent. Family history may reveal miscarriages and neonatal deaths in the maternal line. Ptosis is common, and extraocular muscle weakness is also often present. Indeed, the constellation of marked facial weakness, ptosis, and ophthalmoplegia with generalized hypotonia and weakness is highly suggestive of myotubular myopathy rather than other congenital myopathies ( Table 33.6 ) of either the mild or severe varieties. Cardiomyopathy does not occur, and cognition is normal in survivors.
NEONATAL HYPOTONIA AND WEAKNESS | SEVERE FORM WITH NEONATAL DEATH | FACIAL WEAKNESS | PTOSIS | EXTRAOCULAR MUSCULAR WEAKNESS | |
---|---|---|---|---|---|
Central core disease | + | 0 | ± | 0 | 0 |
Nemaline myopathy | + | + | + | 0 | 0 |
Myotubular myopathy | + | + | + | + | + |
Congenital fiber-type disproportion | + | ± | ± | 0 | + |
The course in the severely affected male infants with the X-linked syndrome has been considered to be nearly uniform evolution to a fatal outcome. However, a more recent series of 55 affected male infants shows that 64% survived beyond 1 year of age, although 60% of these long-term survivors were entirely ventilator-dependent. Only 13% of the long-term survivors did not require at least intermittent mechanical ventilation. Similar data have been reported in other series. In the severely affected infant, because of the failure of lung expansion and the superimposed aspiration secondary to swallowing difficulties, atelectasis and pneumonia are the usual causes of death in the first days or weeks of life. Motor outcome in long-term survivors is very unfavorable in most, who are often bedridden or in wheelchairs. However, in a series of 36 patients, 3 were said to exhibit “no significant disability at 6 months, 5 years, and 7 years, respectively,” and in another group of 55 cases, 7 had only slightly delayed motor milestones. Several patients have developed other medical conditions—such as gallstones, nephrocalcinosis, vitamin K-responsive bleeding diathesis, spherocytosis, and hepatic peliosis—with associated fatal abdominal hemorrhages.
Pathology.
The muscle pathology is distinctive and diagnostic in the presence of the clinical findings. a
a References .
Muscle fibers contain one or more centrally placed nuclei, usually surrounded by an area devoid of myofibrils. With adenosine triphosphatase staining, the region around the nucleus appears as a clear zone or halo ( Fig. 33.25 ). Ultrastructurally, the muscle fibers bear some resemblance to fetal myotubes, with each fiber containing a longitudinal chain of central nuclei ( Fig. 33.26 ). Consistent with these findings suggestive of a disturbance of maturation are other features characteristic of fetal but not mature muscle (i.e., abundant vimentin and desmin [filamentous proteins], neural cell adhesion molecules, and intracytoplasmic distribution of dystrophin). Type I fibers tend to be small, and this type I fiber hypotrophy is usually a very prominent feature. bb References .


Pathogenesis and Etiology.
The pathogenesis of myotubular myopathy is not entirely understood; a maturational arrest has been suggested because of the resemblance of the abnormal fibers to fetal myotubes and the persistence of other features of fetal myotubes, as noted in the previous section. Insight into the potential mechanism of this maturational defect has been gained by identification of the gene at Xq28 (MTM1). MTM1 encodes a protein, myotubularin, that is a phosphatidylinositol phosphatase. Mutations in MTM1 are the most common cause of XLMTM. Myotubularin is involved in diverse and numerous cellular processes, including excitation-contraction coupling, neuromuscular junction structure, satellite cell proliferation and survival, endosomal trafficking, autophagy, and cytoskeletal organization. Which of these functions is altered and how the disturbance leads to the disease remain unclear. Mutations in other genes can also cause myotubular or centronuclear myopathy, with mutations in dynamin-2 ( DNM2 ) recognized as an autosomal dominant cause of the disease (see Table 33.5 ). Autosomal recessive mutations have been identified in BIN1, RYR1 , and TTN genes. In an Italian cohort of 30 patients with centronuclear myopathy of congenital onset, mutations were identified in 25 (83%): 15 in MTM1 , 6 in DNM2 , 3 in RYR1 , and 1 in TTN . The DNM2 mutations causing a presentation in the first years of life are de novo. The clinical picture of infants and children with autosomal recessive centronuclear myopathy may be indistinguishable from the X-linked form, hence the need for genetic diagnosis, especially in the case of male infants.
Laboratory Studies.
Laboratory investigations have included only an occasionally elevated serum CK level. The EMG is usually myopathic, although not impressively so; as with nemaline myopathy, fibrillation potentials are occasionally observed. Chest radiographs often show very thin ribs. A histological diagnosis is usually made by muscle biopsy, and genetic testing is then undertaken to detect a mutation in one of the genes associated with centronuclear myopathy. However, if the phenotype is obvious, the clinician may not order a muscle biopsy and instead proceed directly with genetic testing.
Management.
Management of the severe X-linked form of myotubular myopathy is as discussed for the severe congenital forms of nemaline myopathy (see previous section). The ethical issues concerning ventilatory support of the severely affected infants are obvious.
Congenital Fiber-Type Disproportion
Congenital fiber-type disproportion (CFTD) , initially described by Brooke in 1973, was the fourth identified among the relatively common specific congenital myopathies. Subsequent reports confirmed and amplified the original observations of Brooke. a
a References .
Indeed, variability of the clinical spectrum has been demonstrated conclusively.Clinical Features.
The clinical syndrome of the more than 70 reported cases has included particularly hypotonia and weakness of limb, neck, respiratory, trunk, facial, bulbar, and extraocular muscles. The last feature, ophthalmoparesis, is present in more than half of the patients who are overtly symptomatic in the neonatal period but in fewer than 10% of those who present later. In most cases the deficits in the cases of early neonatal onset are severe. Other musculoskeletal abnormalities are common, such as a long thin face, a high-arched palate, a tented upper lip, limb contractures, short stature, congenital hip dislocations, foot deformities, torticollis, and, later, scoliosis. These abnormalities represent primarily postural deformities, generated either prenatally or postnatally.
The course is variable, but in general it varies according to the time of onset. Thus, among the infants with overt disease of neonatal onset, fully 40% have died because of the combination of bulbar and respiratory muscle weakness. Most surviving infants have had a static course, with generally severe weakness. Infants with slightly later onset are more likely to have the static and then improving course, often considered characteristic of the disorder or, more precisely, group of disorders.
Laboratory Studies.
The serum CK level is usually normal. The EMG can be normal, but it usually exhibits myopathic changes, albeit often not severe.
Pathology.
Muscle pathology establishes the diagnosis. The essential features are the predominance of type I fibers (i.e., type I fibers account for more than 55% of the total [normally, type I fibers account for 30%–55% of the total]) and the small size of type I fibers (i.e., a disparity of 12% or more in the mean diameters of type I and II fibers [normally, the diameters are approximately equal]; Fig. 33.27 ). Subsequently, hypertrophy of type II fibers appears to occur, and the discrepancy between the size of the fibers may become marked. (In several cases, type II fibers were hypotrophic. )

Fiber-type disproportion of the variety observed with CFTD may be observed with other congenital myopathies , particular nemaline myopathy, myotubular myopathy, and congenital myotonic dystrophy. However, in these disorders, additional changes in muscle coexist and serve to emphasize the nosological status of the disorders as well as to lead to the correct diagnosis. Moreover, fiber-type disproportion has been observed in spondyloepimetaphyseal dysplasia and in CNS disorders, such as Krabbe leukodystrophy, adrenoleukodystrophy, and perinatal asphyxia ; in one series, cerebellar hypoplasia was the most common CNS abnormality. Once the known causes of CFTD have been excluded on clinical and pathological grounds, there appears to remain a group of patients in which CFTD is the primary pathological condition.
Pathogenesis and Etiology.
The pathogenesis of CFTD is likely heterogeneous. A functional abnormality in maturation of the motor unit has been suggested for several reasons: (1) similar myopathology can be produced by denervation or cross innervation; (2) type IIc fibers, the fetal precursor of types IIa and IIb fibers, are often present in increased numbers; and (3) fiber-type disproportions of various types may occur with developmental disturbances of brain. The nature of the postulated disturbance in motor unit development is unclear. Numerous documentations of normal anterior horn cells and peripheral nerves in congenital fiber-type disproportion are available.
Approximately 45% of cases have a positive family history; approximately two thirds of these suggest autosomal dominant inheritance, and one third suggest autosomal recessive. In a series of neonatal-onset cases ( n = 3), mutations in the ACTA1 gene (different from those for nemaline myopathy) were detected (see Table 33.5 ). The clinical features were similar to those in the severely affected cases noted earlier except for the absence of ophthalmoparesis. A report of eight cases with onset in the first year or later in infancy and childhood noted defects in the SEPN1 gene (see the description of MmD, next). Mutations of the TPM3 gene, which encodes slow alpha-tropomyosin, are the most common cause of CFTD. This may explain the selective involvement of type 1 fibers in CFTD, since TPM3 is a type 1 fiber–specific protein. Although the mechanism remains unknown, recessive mutations in RYR1 have also been detected in a relatively large subset of CFTD patients with extreme fiber-size disproportion and may be responsible for the observed ophthalmoparesis in certain cases. In several Japanese patients with CFTD, mutations of the LMNA gene were recently identified ; such patients may be at risk for developing cardiomyopathy.
Management.
The most essential aspect of management is recognition that this disorder may be associated with improvement. Thus even severely affected infants should receive vigorous supportive care, and every effort should be made to prevent contractures.
Mitochondrial Myopathies
Mitochondrial myopathy , in this context, refers to a disorder with prominent involvement of muscle and a primary (not secondary) abnormality of mitochondrial structure and function. Abnormalities of mitochondrial structure can sometimes be demonstrated initially on muscle biopsy by the modified Gomori trichrome stain, which reveals accumulations of red-staining material within the fibers (i.e., ragged-red fibers) ( Fig. 33.28A and B ). Further definition of the structural disturbance in mitochondrial number, configuration, or both is made by electron microscopy. Of additional importance is documentation of the abnormalities in mitochondrial biochemical function that occur in mitochondrial myopathies. Finally, as discussed later, study of the ratio of mitochondrial to nuclear DNA is important in identifying the mitochondrial DNA depletion syndrome.

Classification of mitochondrial myopathies according to biochemical defects is valuable , and clear definition of such defects has progressed rapidly. The major metabolic functions of mitochondria in muscle can be categorized relatively simply as follows: (1) substrate transport, (2) substrate utilization, (3) function of the Krebs cycle, and (4) function of the respiratory (electron transport) chain ( Table 33.7 and Fig. 33.29 ). Of these, only myopathic disease related to defects in the respiratory chain is clearly relevant to the newborn (see subsequent discussion).
BIOCHEMICAL DISORDER | CLINICAL FEATURES | |
---|---|---|
PRIMARILY MYOPATHY | PRIMARILY ENCEPHALOPATHY | |
Substrate transport | ||
Carnitine deficiency | + | − |
Substrate utilization | ||
Pyruvate carboxylase | − | + |
Pyruvate dehydrogenase complex | − | + |
Fatty acid oxidation | − | + |
Krebs cycle | ||
Fumarase deficiency | − | + |
Respiratory chain | ||
Complex I | + | + |
Complex II | + | + |
Complex III | + | + |
Complex IV (cytochrome c oxidase) | + | + |
Complex V (adenosine triphosphate synthase) | + | + |
Multiple defects (mitochondrial DNA depletion) | + | + |
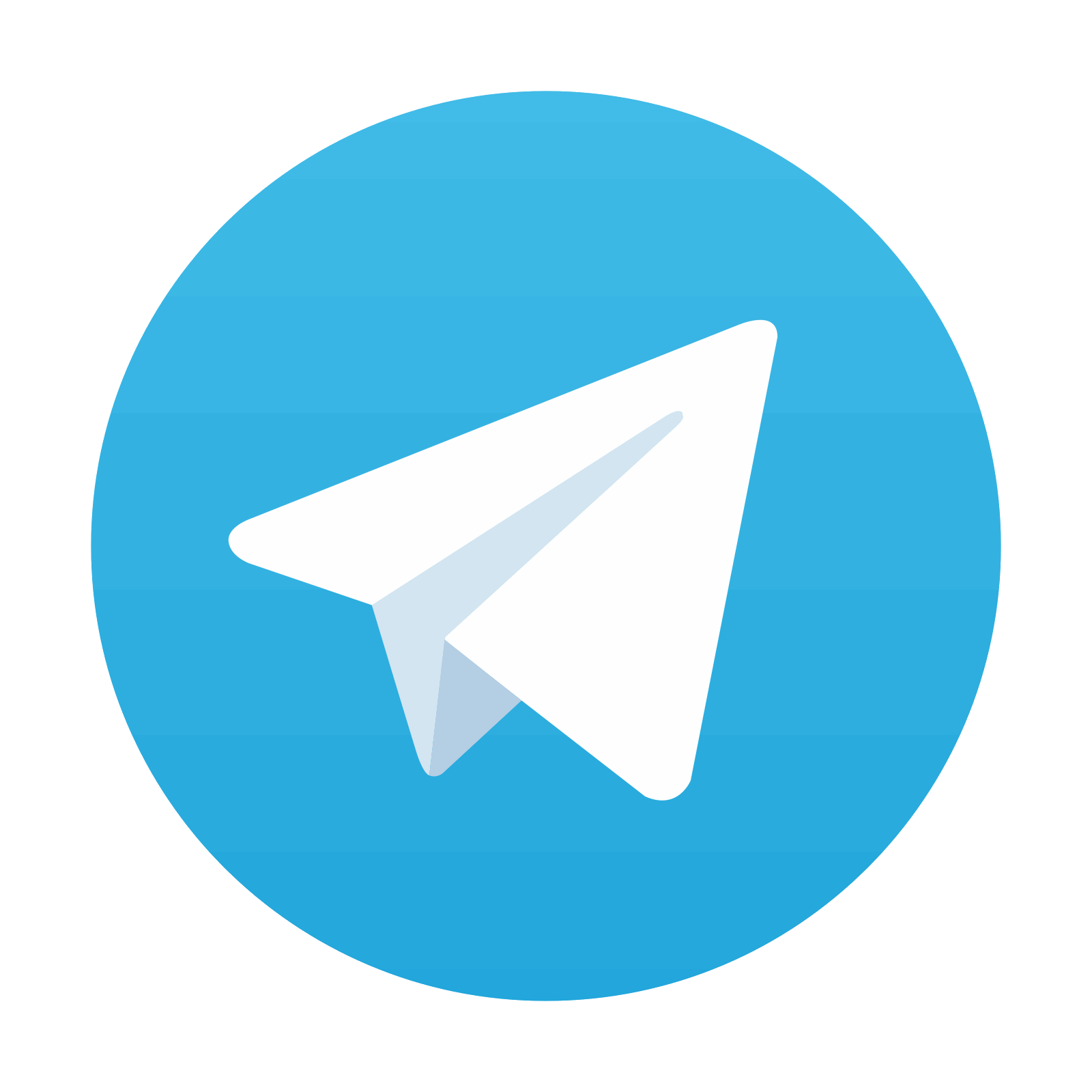
Stay updated, free articles. Join our Telegram channel

Full access? Get Clinical Tree
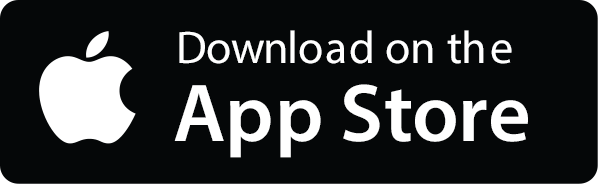
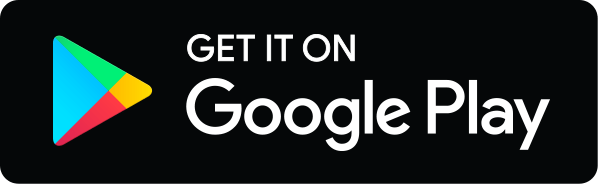
