Fig. 3.1
Impact of sympathetic nerve stimulation on hemodynamics in a dog. Random electrical stimulation with an average of 1 Hz enhanced heart rate, left ventricular systolic pressure, and first derivative of left ventricular pressure (Modified from Ushijima R, Joho S. Evaluation for sympathetic nerve activity. Heart View. 2014;18(1):36)
The short-term activation of sympathetic nerve activity is known as the “fight or flight response” that prepares the body to either stay and fight or flee from a situation [1]. By contrast, the long-term excess activation of sympathetic nerves is pathological, being involved at the onset and progression of cardiovascular diseases such as hypertension, cardiac hypertrophy, myocardial ischemia, heart failure, and arrhythmias [2, 3]. The relationship between sympathetic nerve activity and cardiovascular diseases is important not only to understand autonomic status but also to consider its influence on prognosis and therapy. Therefore, sympathetic nerve activity should be accurately evaluated in each individual.
Sympathetic nerve activity in clinical practice can be evaluated by measuring blood or urinary levels of neurotransmitters (norepinephrine), measuring the responses of effectors (heart rate and blood pressure), neurotransmitter imaging (meta-iodobenzylguanidine (MIBG) scintigram), and measuring the electrical activity of nerves. These methods should be selectively used depending on the nature of the studies.
Muscle sympathetic nerve activity (MSNA) is a vasomotor activity that governs the vascular smooth muscles distributed throughout the skeletal muscle. MSNA varies in conjunction with the instantaneous fluctuations of blood pressure via arterial baroreceptors to maintain constant blood pressure. A percutaneously inserted microelectrode is directly placed in muscle nerve fascicles of peripheral nerves. The major advantages of this method are that sympathetic neural activity can be directly and instantaneously recorded unlike any other indirect assessment of sympathetic activity. Thus, variations in sympathetic activity over time can be visualized using this method (Fig. 3.2).


Fig. 3.2
Arterial pressure and muscle sympathetic nerve activity (MSNA). Control individual received phenylephrine injections and inhaled amyl nitrate (Modified from Ushijima R, Joho S. Evaluation for sympathetic nerve activity. Heart View. 2014;18(1):41)
Despite the advantages of MSNA, it is not frequent to apply this method in the clinical tests. The main reason might be mainly due to the difficulty of recording technique. However, to verify findings using MSNA is important for establishing evidence of the relationship between sympathetic nerve activity and cardiovascular diseases. We review recent clinical findings of the relationship between microneurographically recorded MSNA and various cardiovascular diseases as well as the pathophysiology underlying these diseases.
3.2 Ischemic Heart Disease
Animal experiments have shown that cardiac sympathetic nerve activity is enhanced by stimulating sympathetic afferent fibers with myocardial ischemia [4–6]. However, whether myocardial ischemia elicits sympathetic hyperactivity in patients with coronary artery disease has remained unclear. A recent study of MSNA during interventional coronary angioplasty in patients with coronary artery disease found that MSNA was increased by an average of 36 % from baseline during coronary artery occlusion [7] and in 10 of 12 patients regardless the site of such occlusion. Another study found that treating myocardial ischemia by coronary angioplasty can effectively reduce MSNA 1 month after balloon coronary angioplasty [8]. Neither of these studies found any significant changes in heart rate and blood pressure. These results imply that to determine changes in sympathetic activity related to myocardial ischemia from the response to the effector is difficult. Changes in MSNA without a change in blood pressure might occur through changes in input from sympathetic afferent fibers derived from the ischemic myocardium rather than by any alterations in blood pressure.
On the other hand, MSNA peaks during the acute phase (2–4 days after onset) of myocardial infarction and gradually decreases during the chronic stage (several months after onset) [9]. Furthermore, MSNA is significantly higher in patients with heart failure accompanied by ischemic than nonischemic cardiomyopathy [10]. Myocardial ischemia is frequently residual even after coronary angioplasty intervention in patients with coronary heart disease dependently upon the severity of residual coronary artery stenosis. Residual myocardial ischemia stimulates the sympathetic ascending fibers in the myocardium, which might increase the level of MSNA.
3.3 Resistant Hypertension
Resistant hypertension is defined as blood pressure above a target of 140/90 mmHg despite the concomitant administration of at least three antihypertensive agents from at least three classes of such agents including diuretics [11]. The level of MSNA was significantly higher in such patients, compared with those who had nonresistant hypertension even after excluding complications associated with sympathetic hyperactivity such as heart failure, obesity, obstructive sleep apnea, and renal failure [12]. Sympathetic hyperactivity reduces renal blood flow through the renal efferent sympathetic nerve, which could decrease sodium diuresis. Thus, renal sympathetic nerve denervation increases renal blood flow through a blockade of renal sympathetic efferents and probably increases natriuresis [13].
Catheter-based radiofrequency ablation has recently been used to disrupt the renal nerves of patients with resistant hypertension [14]. Renal sympathetic denervation results in an obvious reduction in renal norepinephrine spillover from the kidney, which indicates the effectiveness of the intervention. Importantly, MSNA, whole-body norepinephrine spillover, and blood pressure became decreased after this procedure [15]. Moreover, electrical autonomic nerve stimulation of the renal artery can increase blood pressure in the experimental setting, but this response is completely abolished after radiofrequency ablation of the renal artery [16]. These results could support the possibility that inhibition of afferent renal nerve activity might contribute to a reduction in central sympathetic drive.
So far, several studies examined the effect of renal denervation on blood pressure and MSNA in patients with resistant hypertension [17–20]. However, the findings were inconsistent. Renal denervation substantially reduced MSNA in two reports [18, 20], but did not change in other reports [17, 19].
This could be explained by a lack of sufficient renal sympathetic nerve ablation resulting in unsatisfactory blood pressure and sympathetic responses to renal denervation. Recently, it was investigated that the potential impact of various renal anatomies on blood pressure and MSNA responses to renal denervation in patients with resistant hypertension [20]. Interestingly, renal denervation significantly reduced blood pressure and MSNA in patients with single renal arteries bilaterally, but not in patients with dual renal arteries on either one or both sides [20].
Renal ischemia or injury might stimulate afferent signaling from the kidney to central integrative nuclei, with the consequence of exaggerated efferent sympathetic outflow to vasculature and the kidneys [21]. Besides sustained blood pressure reduction, the regression of left ventricular hypertrophy, a reduction in the size of the left atrium, an increase in left ventricular ejection fraction, and improved left ventricular diastolic function were evident at 6 months after renal denervation [22] (Fig. 3.3). Renal denervation inhibited the progression from cardiac hypertrophy to heart failure in animal models [23]. Therefore, renal denervation might help to interrupt this vicious cycle.


Fig. 3.3
Time course of left ventricular mass (LVMI) and changes in LVMI in group with renal sympathetic nerve ablation (RD) and control group (From reference [22]). LVMI was decreased in RD, but not in control group (upper). Effects of renal denervation on left ventricular hypertrophy were compared among three groups of patients whose blood pressure changed after renal denervation. Left ventricular hypertrophy regressed even in group with minimal antihypertensive effects
3.4 Chronic Heart Failure
3.4.1 Mechanisms of Sympathetic Hyperactivity in Chronic Heart Failure
Sympathetic overactivation is common in patients with chronic heart failure and it is recognized as a cause of exacerbated heart failure. Figure 3.4 shows representative traces of MSNA obtained from a healthy individual and from a patient with chronic heart failure. A heartbeat-synchronized MSNA burst occurs for every heartbeat in patients with heart failure, suggesting obviously increased sympathetic activity. Both plasma norepinephrine levels [24] and MSNA [25] can serve as independent prognosticators of chronic heart failure (Fig. 3.5).



Fig. 3.4
Representative tracing of muscle sympathetic nerve activity (MSNA), electrocardiogram, blood pressure, oxygen saturation, and respiration obtained from healthy individual (left) and patient with NYHA class III chronic heart failure (right). Neural activity is synchronized with heart beat (burst) in patient

Fig. 3.5
Kaplan-Meier analysis of cumulative rates of survival in patients with heart failure stratified into two groups based on muscle sympathetic nerve activity (MSNA, bursts/min) (From reference [25]). Survival rate was significantly lower in patients with MSNA >49 bursts/min (P = 0.001)
The cause of central sympathetic hyperactivity in patients with chronic heart failure has not been established. Recent concepts include factors from the central nervous system that affect the sympathetic activity (Fig. 3.6) [26].


Fig. 3.6
Mechanisms of central sympathetic hyperactivity in chronic heart failure (From reference [26])
A tonic inhibitory restraint on the brain stem centers arises from arterial and cardiopulmonary baroreceptors.
Inactivation of the inhibitory baroreceptors through decrease in cardiac filling pressure or arterial pressure yields an decrease in afferent inhibition of the brain stem vasomotor center with an increase in efferent sympathetic tone. When the pump function of the heart fails, both decreased blood pressure and depressed contractility attenuate afferent impulses from aortic baroreceptors and result in sympathetic hyperactivity. Conversely, stimulating afferent nerves from the aortic baroreceptor using “baroreceptor activation therapy” inhibits MSNA [27].
Other mechanisms of sympathetic activation referred to as excitatory stimuli are independent of baroreceptor unloading and possibly cause a sympathetic reaction that is excessive for maintaining homeostasis through increasing the set point of the central sympathetic activity in the paraventricular nucleus [26]. Excitatory stimulus mechanisms through chemoreceptors located in the carotid sinus and aortic arch, skeletal muscle (muscle metaboreceptors), kidney, and lungs include enhanced mechanisms of sympathetic nerve activity [26]. Activation of these afferent mechanisms via ischemic metabolites in exercising muscle or via arterial hypoxemia, acidemia, and hypercapnia results in an increase in afferent excitatory influences on the brain stem vasomotor centers and causes an increase in efferent sympathetic neural outflow. These mechanisms are activated with the development of heart failure and then form a vicious cycle of exacerbating heart failure.
Thus, sympathetic hyperactivity that characterizes heart failure might reflect the sum of (1) a decrease in the afferent inhibitory restraint arising from cardiopulmonary and arterial baroreceptors (compensatory responses to reduced blood pressure or systolic function) and/or (2) an increase in the afferent excitatory influence of muscle metaboreceptors and/or arterial chemoreceptors.
3.4.2 Effects of Standard Pharmacological Therapy
Considerable improvements in the therapeutic approach to heart failure have led to a reduction in hospitalization and a significant reduction in mortality rates over the past 20 years [28, 29]. These improvements were due to the establishment of a pharmacological approach with beta-adrenergic blockers, angiotensin-converting enzyme (ACE) inhibitors, angiotensin receptor blockers (ARB), and mineralocorticoid receptor blockers. As a current standard treatment for chronic heart failure, β-blockers dose dependently block the action of endogenous epinephrine and norepinephrine on β-receptors and weaken the toxicity of catecholamines to heart muscle cells. On the other hand, β-blockers do not reduce MSNA in the acute phase [30], but do so over the long term [31]. Mineralocorticoid receptor blockers, ACE inhibitors, ARB, and digitalis are also standard treatments for heart failure. Either ACE inhibitors or ARB are usually administered to all patients, and mineralocorticoid receptor blockers are given to ≥50 % of patients. Statins are also administered to many patients with risk factors for coronary artery disease. Importantly, these drugs have a central sympathetic inhibitory effect [32–35]. An increase in reactive oxygen species in the sympathetic central rostral ventrolateral medulla (RVLM) enhances the sympathetic activity [36]. For example, statins attenuate sympathetic activity by suppressing active oxygen [37].
Therefore, these medications should inhibit central sympathetic activity in addition to the original purpose.
3.4.3 Sympathetic Suppression by Treatment for Breathing Abnormalities
Breathing abnormalities such as rapid shallow breathing and periodic breathing during the daytime or sleep apnea during the nighttime are commonly found (50–60%) in patients with chronic heart failure [38–40]. Patients with breathing abnormalities have notably higher sympathetic nerve activity [40, 41]. Decreased lung stretch [42, 43], intermittent hypoxia [44, 45], and hypercapnia [46, 47] might all increase MSNA (Fig. 3.6).
Enhanced sympathetic activity can be suppressed by correcting breathing abnormalities in patients with heart failure. Device-guided slow and deep respiration suppresses steady-state sympathetic nerve activity in patients with heart failure and high levels of resting sympathetic tone [48]. Adaptive servo-ventilation (ASV), which is a novel method of ventilatory support designed for Cheyne-Stokes respiration (CSR) [49], improved respiratory instability and decreased MSNA in patients with heart failure (Fig. 3.7) [50]. The sympathoinhibitory action of ASV might be due mainly to respiratory normalization via a servo-ventilation effect [50]. Although long-term use of continuous positive airway pressure (CPAP) decreases MSNA in heart failure patients with obstructive sleep apnea [51], short-term use (30 min) of CPAP did not always decrease MSNA in patients with heart failure [52–55].


Fig. 3.7
Effect of adaptive servo-ventilation (ASV) on muscle sympathetic nerve activity (MSNA) and respiration (From reference [50]). Respiration became stabilized and MSNA became decreased during application of ASV
Patients with heart failure and obstructive sleep apnea have sympathetic hyperactivity during the daytime [56]. One month of CPAP reduced MSNA by 12 bursts/100 beats in patients with obstructive sleep apnea [51] and CPAP increased their cardiac function [57] while improved their prognosis [58]. On the other hand, sympathetic hyperactivity is also evident in patients with heart failure and central sleep apnea [56]. In patients with heart failure and moderate to severe central sleep apnea, ASV decreased MSNA by 9 bursts/100 beats in association with improved cardiac function [59] (Fig. 3.8). Furthermore, changes in left ventricular ejection fraction and MSNA closely correlated with the suppression of central sleep apnea using ASV. Thus, respiratory stabilization might be associated with improved sympathetic nerve activity and cardiac function [59].


Fig. 3.8
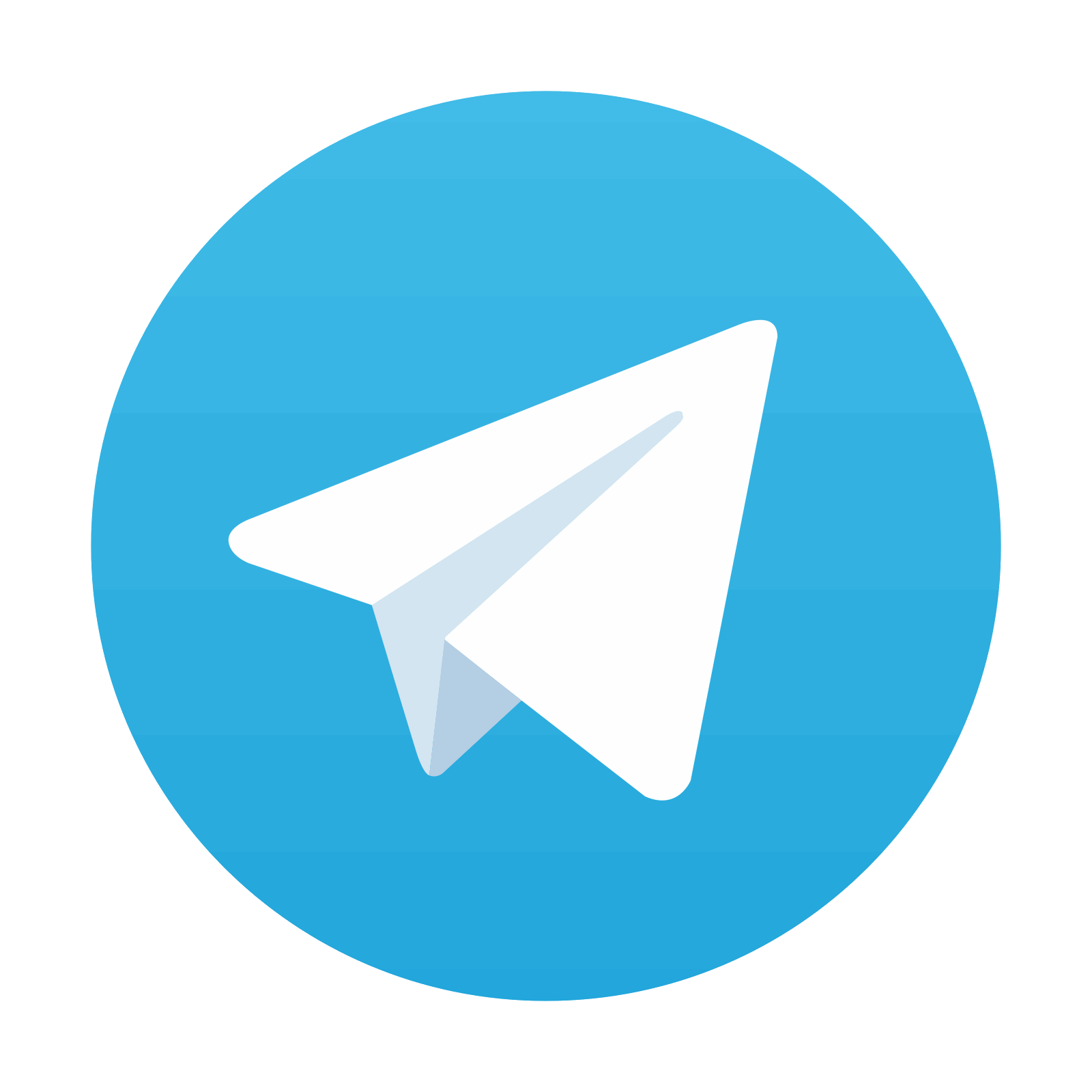
Representative trace of electrocardiogram (ECG), blood pressure (BP), muscle sympathetic nerve activity (MSNA), and oxygen saturation (SpO 2 ) at baseline and at 3 months after adaptive servo-ventilation (ASV) in a patient with chronic heart failure (From reference [59]). Sympathetic nerve activity was reduced and left ventricular ejection fraction (LVEF) was increased in ASV group, whereas MSNA and cardiac function remained unaltered in control group
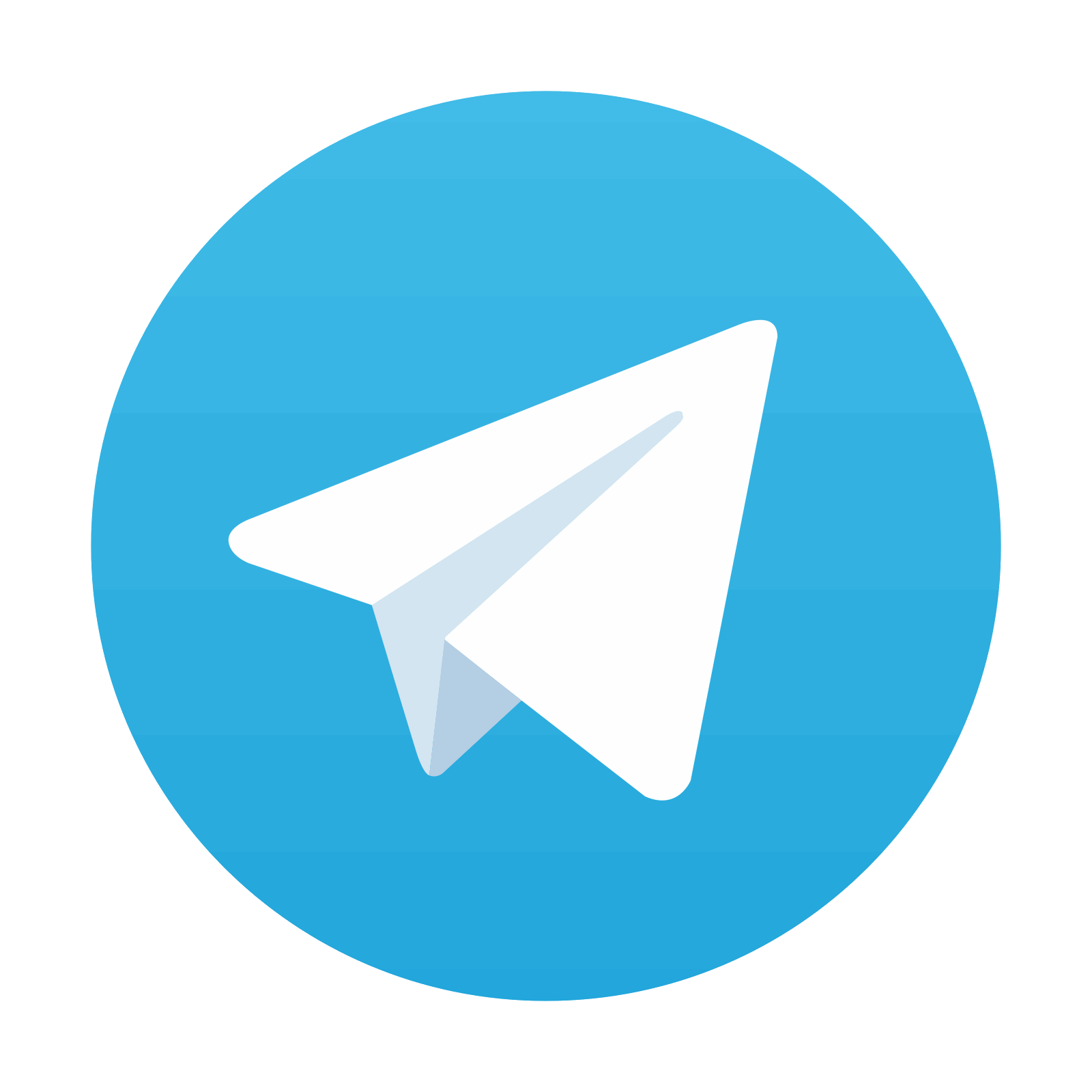
Stay updated, free articles. Join our Telegram channel

Full access? Get Clinical Tree
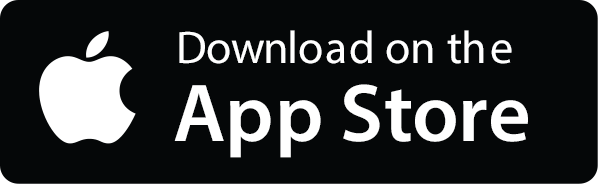
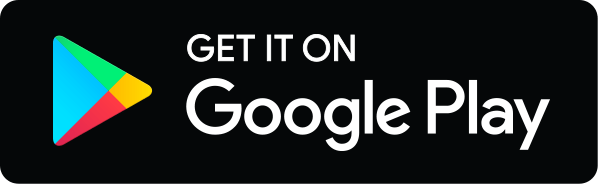
