Myopathies are diseases of skeletal muscle. Chronic or subacute weakness from myopathy is usually evaluated in the clinic. In the hospital setting, myopathy is most likely to present as a new condition for evaluation in the critically ill patient or the patient with an acute myopathy with rapidly progressive weakness. These situations will be discussed separately.
There are many disorders that may present as a subacute or acute undiagnosed problem in the hospital setting. These include inflammatory myopathy, metabolic myopathy, toxic myopathy, and myopathy due to a systemic disorder (Table 42-1).
Causes of Myopathy in the Hospitalized Patient
|
When considering the weak patient in the hospital, other disorders besides myopathy must be considered. Disorders of the neuromuscular junction, motor nerves, anterior horn cells, and motor tracts of the brain or spinal cord may mimic a myopathy.
The approach to diagnosis of myopathy begins with a history. This includes medications and other supplements and symptoms and signs of involvement of the heart, pulmonary system, skin, and joints.23
Symptoms are usually considered to be either positive or negative symptoms. The most common symptom, weakness, is a negative symptom. Exercise intolerance, fatigue, and muscle atrophy are other negative symptoms. Positive symptoms include cramps, contractures, muscle hypertrophy, muscle stiffness, myalgia, and myoglobulinuria.
Most patients with myopathy report weakness. Proximal weakness is the most common pattern seen in myopathy. Proximal weakness of the lower extremities is seen in rising from a chair and in climbing and descending stairs. Proximal weakness in the upper extremities is seen particularly while raising one’s arms above the head to reach for an object or for grooming. If the patient complains of foot drop or hand weakness, distal involvement is present. This can occur in myopathy, but may be a clue of an alternative diagnosis. Cranial muscle involvement may occur with dysphagia, dysarthria, double vision, and ptosis, but this pattern of involvement is much less common.
A feature of metabolic and mitochondrial myopathies is especially fatigue and sometimes pain after exercise. Myalgias may occur in myopathy, but most patients with myopathy do not have pain. Myopathies associated with pain are listed in Table 42-2.
Myopathies Associated with Myalgia
|
Identifying the onset of symptoms is a crucial step in diagnosing a patient, but may be surprisingly difficult, particularly if a disorder has been slowly progressive. In the hospital patient, most myopathies have an acute or subacute presentation, as is seen in dermatomyositis or a toxic myopathy. Slowly progressive weakness is generally seen in the muscular dystrophies and in inclusion body myositis. Nonprogressive static weakness is seen in congenital myopathies.
A family history is most consistent with a muscular dystrophy, congenital myopathy, or hereditary channelopathy.
The patient should be asked about the history of medication and recreational drug use. Myotonic disorders often present with stiffness worsened by cold exposure. Exercise-induced pain, weakness, or myoglobinuria raises the possibility of a metabolic myopathy. Periodic paralysis is provoked by exercise and a high-calorie meal.
Has the patient had weight loss or gain, night sweats, rash, change in hair or skin texture, edema, or myoglobulinuria?
Most myopathic disorders have predominantly proximal limb muscle weakness. If present, bulbar or ocular involvement, neck flexor or extensor weakness, and distal muscle involvement particularly may be helpful in suggesting the underlying diagnosis.
CASE 42-1
A 64-year-old woman was admitted to the intensive care unit (ICU) with sepsis and respiratory failure requiring intubation. According to her daughter, she was under treatment for chronic obstructive pulmonary disease and hypertension, but lived independently. On admission, her chest radiography showed a right lower lobe infiltrate consistent with pneumonia. She was treated with intravenous (IV) methylprednisolone, antibiotics, and IV fluids. Light sedation was used to maintain comfort, and she did not receive ongoing neuromuscular blockade. On the second day of admission, she developed acute renal insufficiency and new bilateral pulmonary infiltrates on chest radiography with worsening hypoxia. She was diagnosed with acute respiratory distress syndrome and acute tubular necrosis. With treatment, the pulmonary infiltrates and acute tubular necrosis improved; however, the patient did not make progress weaning from the ventilator. The nurses and ICU physicians noted that she grimaced with discomfort during their assessments, but did not withdraw to painful stimulation. Her neurologic examination showed that she was alert when sedation was held and reliably blinked and grimaced to command. She had intact pupillary reflexes to light, and extraocular movements were intact. No ptosis was noted. She had weak neck flexion and extension, with muscle weakness both proximally and distally. Her strength in wrist flexion, forearm flexion, shoulder abduction, ankle dorsiflexion, knee extension, and hip flexion was 0 using the Medical Research Council (MRC) scale. Patellar reflexes were normal, and ankle reflexes were absent. Muscle tone was flaccid. The plantar responses were flexor, and no upper motor neuron findings were noted. She grimaced with light, painful stimulation of the extremities but no limb movement was seen.
ICU-acquired weakness has a reported prevalence of 25–50%. ICU patients usually are noted to have weakness either when the patient is difficult to wean from the ventilator or if the patient develops quadriparesis early in an admission, raising the possibility of Guillain-Barré syndrome (GBS) or other primary neurologic cause for respiratory failure.25
In the aforementioned case, the patient initially presented to the ICU for treatment of sepsis with a history of independent living prior to admission. A previously unrecognized, underlying neurologic etiology for weakness is therefore much less likely.
In this situation, the patient usually has critical illness polyneuropathy (CIP), critical illness myopathy (CIM), or a combination of both, i.e., critical illness neuromyopathy (CINM). Some investigators have advocated for the use of the term “ICU-acquired weakness” in this situation if no other plausible cause is noted, as the diagnoses often overlap. The underlying pathogenesis of these disorders remains poorly understood. Although other diagnoses are less frequent, evidence of an alternative diagnosis should be considered in every patient.
The patient with flaccid quadriparesis on admission, or developing shortly after admission, is usually suspected of having GBS; however, there is potentially a broad differential diagnosis (Table 42-3).
Causes of Flaccid Quadriparesis
|
Sepsis
Multiple organ failure
Mechanical ventilation
Hyperglycemia
Exposure to corticosteroids
Exposure to neuromuscular blocking agents
The diagnostic approach begins with the history and the physical examination. A standard examination of muscle strength is the MRC scale for muscle examination, testing in the upper extremities wrist flexion, forearm flexion, and shoulder abduction, and in the lower extremities, ankle dorsiflexion, knee extension, and hip flexion (Table 42-4). Initially the presence of delirium or sedation may make the definite diagnosis of weakness difficult. If a patient does not move limbs to painful stimulation but grimaces, this is an important clinical clue to the presence of weakness. Initially a broad differential diagnosis of the causes of quadriparesis should be considered, particularly if the patient arrived at the hospital in a comatose or obtunded state. The presence of Babinski signs or other upper motor neuron sign is a clear-cut finding of central nervous system involvement and is not compatible with a diagnosis of isolated CIP/CIM or GBS. If upper motor neuron findings are present, appropriate imaging is needed.
If the history and examination are compatible with a peripheral cause, nerve conduction studies (NCSs) and EMG study should be performed.
There has been controversy about the necessity for these studies in a clinical situation suggestive of CIP/CIM as the studies are technically difficult; however, our view is that the studies should be performed. The studies help confirm the presence of findings consistent with CIP, CIM, or both, and can exclude mimics such as a neuromuscular junction (NMJ) disorder.
In the patient with flaccid quadriparesis, the studies are likewise critical.
Electrodiagnostic findings in CIM/CIP are listed in Table 42-5. CIM is suggested electrodiagnostically by a prolonged CMAP duration. CIM is an acute primary myopathy with or without fibrillation potentials. Muscle biopsy reveals preferential loss of myosin filaments, type II fiber atrophy, and muscle necrosis. Underlying etiology and pathogenesis are not well understood. The CK levels are normal in this condition. Muscle biopsy is required for a definitive diagnosis.
Nerve Conduction Studies of ICU-Acquired Weakness
|
In patients with CIP, electrodiagnostic testing shows sensorimotor axonopathy with decreased compound muscle action potentials (CMAPs) and sensory nerve action potentials (SNAPs) but relatively normal conduction velocities. Associated CIM is extremely common. It is usually difficult to determine if superimposed CIM is present based on a bedside examination alone.
Strict diagnostic criteria have not been developed for this disorder. Recently criteria for the diagnosis of “ICU associated weakness” have been proposed (Table 42-6). This allows patients to be presumptively diagnosed pending the results of electrodiagnostic testing and muscle biopsy.
Diagnostic Criteria for ICU-Associated Weakness
|
Studies on the prevention of CIM/CIP suffer from the lack of strict diagnostic criteria. Intensive insulin therapy significantly reduced CIM/CIP and reduced the duration of mechanical ventilation, ICU stay, and 180-day mortality. Corticosteroids did not have an effect on CIM/CIP. Early physical therapy reduced CIM/CIP in the evaluable patients in the ICU, but statistical significance was lost when a full intention-to-treat analysis was performed. Electrical muscle stimulation has not been shown to have a significant effect on the incidence of CIM/CIP. Although intensive insulin therapy reduced the incidence of CIM/CIP, the risk of adverse events is higher and carries significant risk.
Most toxic myopathies are caused by prescription medications.
Statin myopathy is the most common toxic myopathy currently, and will be discussed first and as a separate topic.
A toxic myopathy is recognized by the subacute or acute onset of myopathic symptoms or signs that occur in patients without muscle disease when exposed to therapeutic doses of a drug.
In most cases, muscle symptoms and signs usually improve with cessation of the medication, with the important exception of statin-triggered autoimmune necrotizing myopathy.
A toxic or drug-induced myopathy should be suspected when a patient develops myalgia, weakness, myoglobinuria, or fatigue in association with exposure to a drug.
It is important to diagnose a toxic myopathy to prevent further muscle damage.
Statin muscle toxicity can be divided into two types: self-limited symptoms (mild or severe) that improve with cessation of the medication and a statin-triggered autoimmune necrotizing myopathy.
Statin toxicity includes the following presentations:
Asymptomatic CK elevation
Myalgia with or without CK elevation
Necrotizing myopathy
Rhabdomyolysis
In addition, statin use may worsen an undiagnosed, preexisting myopathy.
Statin-triggered autoimmune necrotizing myopathy is an infrequent, severe, and progressive disorder that requires immunosuppression for treatment.
The diagnosis of statin-associated myopathy is hampered by lack of generally accepted diagnostic criteria.
The incidence of statin-associated myalgias has been estimated to be approximately 5–10%. This is higher than that seen in randomized-controlled trials of statin use, possibly due to exclusion of higher-risk patients from these trials. Diagnostic criteria are not standardized, and many patients likely have discomfort due to other problems.
The rate of rhabdomyolysis due to statin use has been reported to be 0.44 cases per 10,000 patient-years. Due to the large number of patients taking statins, rhabdomyolysis is regularly seen in neurology practice.29
Statins inhibit HMG-CoA reductase and decrease levels of mevalonate, a cholesterol precursor. The mechanism of muscle damage in self-limiting statin myopathy is hypothesized to be due to disruption of the muscle membranes, caused either directly by decreased cholesterol or by a decrease in other downstream products of mevalonate.
The mechanisms by which statins trigger autoimmune necrotizing myopathy are incompletely understood; however, most patients with this condition have MHC-1 expression on the sarcolemma and also have antibodies to HMG-CoA reductase.1
Risk factors include the following:
Type of statin (increased risk if metabolized by CYP3A4 P450)
Atorvastatin, simvastatin > fluvastatin, pravastatin > rosuvastatin
Co-administration of other medications metabolized by CYP3A4 P450 (see text below)
Higher dose of statin
Older patient14
Liver disease
Hypothyroidism
Obesity
Homozygosity of the SLCO1B1 polymorphism (approximately 2% of the general population)
The statin medications simvastatin, atorvastatin, and lovastatin are metabolized by the CYP3A4 P450 system, while pravastatin, fluvastatin, and rosuvastatin are not. Based on reporting to the United States Food and Drug Administration (FDA) of cases of statin-associated rhabdomyolysis, the risk of statin-associated myopathy is higher with simvastatin and atorvastatin than with pravastatin and fluvastatin.
The risk of statin-associated myopathy is considered to be dose-related.
Co-administration of medications metabolized by the CYP3A4 P450 system potentiates toxicity from simvastatin, atorvastin, and lovastatin. These include tricyclic antidepressants, verapamil, diltiazem, gemfibrozil and other fibrates, warfarin, cyclosporine, tacrolimus, amiodarone, macrolide antibiotics, azole antifungal agents, HIV-protease inhibitors, colchicines, and tamoxifen. Coadministration of ezetimibe and niacin also has been reported to raise the risk of statin-associated myopathy.
A polymorphism in the SLCO1B1 gene was identified as a significant risk factor for self-limited statin-associated myopathy.29,35 The SLCO1B1 gene encodes the protein for hepatic uptake of statins. Homozygosity of a mutant allele occurs in about 2% of the general population. The risk of self-limited statin-associated myopathy in these patients is markedly elevated. In contrast, the risk of statin-triggered autoimmune necrotizing myopathy is not increased in these patients.
What are the known risk factors for the development of statin-triggered immune-mediated necrotizing myopathy?
Class II HLA allele DRB1*11:01 in whites and African Americans.
Lower risk is found in association with Class II DQA1 and DQB6.
A patient with complaints of myalgias or weakness requires an examination for weakness and a creatinine kinase (CK) level. If these are normal, the symptoms likely represent a mild disorder that may or may not be statin-associated. The statin should be discontinued if possible. If the symptoms subsequently have resolved, a trial of treatment with a different statin at low dose may be considered.
If a patient has weakness and elevated CK level, a statin-associated myopathy should be suspected. CK level may be very high if necrotizing myopathy is present. Nerve conduction studies (NCVs) and electromyography (EMG) are appropriate to confirm the clinical impression of myopathy and exclude other diagnoses. Typically needle EMG shows short-duration, small-amplitude motor units with excessive polyphasia. There is often increased insertional activity.
The statin should be discontinued with close follow-up. If a self-limited statin-associated myopathy is present, weakness and CK level should begin to improve within several weeks.
If, however, the weakness worsens or does not improve after several weeks, further evaluation is needed. Muscle biopsy should be performed to confirm that a necrotizing myopathy is present, and exclude other muscle diseases that may have been exacerbated by steroid use.
An anti-HMG-CoA reductase antibody is present in most patients with statin-triggered immune-mediated necrotizing myopathy. A test for this antibody is now commercially available. As the antibody test has a high specificity for this disorder, a positive result may be very helpful in confirming the diagnosis.
Self-limited statin myopathy often does not require a muscle biopsy. In patients with statin myopathy with weakness, muscle biopsy has been reported to show the following:
Increased cytoplasmic lipid droplets
Occasional cytochrome-oxidase negative fibers
Occasional ragged-red fibers
Findings consistent with necrotizing myopathy (see below)
Resolution of abnormalities on subsequent biopsy following cessation of the statin has been described.
Statin-triggered autoimmune necrotizing myopathy typically shows the following features consistent with necrotizing myopathy:
Myofiber degeneration
Myofiber necrosis
Myophagocytosis
Myofiber regeneration
Absence of prominent lymphocytic infiltrates
In addition, biopsies from patients with autoimmune necrotizing myopathy may show MHC-1 expression and complement fixation on the sarcolemma.
The treatment of self-limited statin-associated myopathy is statin withdrawal, regardless of whether the weakness is mild, moderate, or severe. Improvement should begin within a few weeks. Complete or nearly complete recovery is expected. While improvement begins quickly following cessation of statins, complete resolution may take as long as 14 months.
Statin-triggered immune-mediated necrotizing myopathy is a progressive disorder. The progression of proximal weakness occurs over a variable time period, from weeks to years. Treatment is immunosuppression; however, large studies have not been performed. Anecdotally, the myopathy often requires aggressive immunosuppression, and relapse often occurs when immunosuppression is tapered. Combined treatment with high-dose prednisone, a steroid-sparing immunosuppressant (ie, methotrexate, azathioprine, mycophenolate mofetil), and IVIG has been used and reported to be successful. All immunosuppression for this condition is off-label. The optimum duration of therapy and dosing of these treatments are unknown.
Necrotizing myopathy occurs as a toxic myopathy with use of fibrate medications, and also may occur rarely with labetalol and propofol. If a toxic myopathy has been excluded, necrotizing myopathy may be paraneoplastic, but is often due to a primary autoimmune process and requires immunotherapy.
Multiple medications and other substances may cause toxic myopathy. Painful myopathy may be seen with use of amiodarone, labetalol, nifedipine, cyclosporine, D-penicillamine, epsilon-aminocaproic acid, procainamide, emetine, salbutamol, gemfibrozil, L-tryptophan, gold, vincristine, heroin, and zidovudine. Steroid myopathy is a common adverse effect of corticosteroid use and is usually not painful.
Selected drugs are discussed at greater length below:
Corticosteroids:
Chronic use of high-dose corticosteroids (>30 mg per day of prednisone or equivalent corticosteroid) may lead to steroid myopathy, usually over the course of months of treatment. The patient develops atrophy of the type II muscle fibers, but the underlying pathophysiology is not well understood. Clinically the patient presents with painless progressive proximal weakness and usually a normal creatinine kinase level. Dexamethasone is more likely to cause myopathy than prednisone or hydrocortisone. Women are more susceptible than men. EMG typically shows subtle myopathic features without fibrillation potentials, but is often normal. Improvement occurs with cessation of the drug.
Hydroxychloroquine/chloroquine:
These medications are used to treat systemic lupus erythematosus (SLE) and other autoimmune diseases, and act by inhibiting intracellular toll-like receptors. They cause myopathy by disrupting muscle membranes. Clinically the patient presents with painless progressive proximal weakness and elevation of CK level. EMG typically shows a myopathy with fibrillation potentials. Abnormalities of sensory and motor nerve conduction studies may also be seen. Muscle biopsy may show a vacuolar myopathy due to lipid deposition; with electron microscopy, myeloid bodies and curvilinear bodies are seen. Improvement occurs with cessation of the drug.
Amiodarone:
Amiodarone may cause both a neuropathy and myopathy. Amiodarone disrupts cellular membranes. Clinically the patient presents with painful proximal weakness and superimposed peripheral neuropathy. Amiodarone myopathy is more common in patients with renal insufficiency. EMG typically shows a myopathy with active denervation. Abnormalities of sensory and motor nerve conduction studies may also be seen. Muscle biopsy may show a vacuolar myopathy due to lipid deposition. Improvement occurs with cessation of the drug.
Colchicine:
Colchicine disrupts the assembly of microtubules, and may lead to both myopathy and neuropathy with chronic use. Typically the patient develops progressive proximal weakness with an elevated CK. Colchicine myopathy and neuropathy are more common in patients with renal insufficiency. EMG typically shows a myopathy with myotonia. Muscle biopsy shows autophagic vacuoles and lysosomal accumulation. Improvement occurs with cessation of the drug.
Zidovudine and other nucleoside reverse-transcriptase inhibitors:
This group of medications treat HIV infection by interfering with the viral reverse transcriptase. They also interfere with mitochondrial DNA polymerase, leading to abnormal mitochondrial function. Typically the patient presents with myalgias, progressive weakness, and mildly elevated creatine kinase level. EMG shows findings consistent with a myopathy with increased insertional activity. Muscle biopsy demonstrates ragged-red fibers on Gomoritrichrome staining due to accumulation of abnormal mitochondria. This finding and the absence of inflammation distinguish this from HIV-associated polymyositis or inclusion body myositis. Improvement occurs with cessation of the medication.
Alcohol:
Excessive alcohol use may cause a toxic myopathy with elevated CK and a myopathic EMG with increased insertional activity. Improvement occurs if alcohol is avoided.
Muscle biopsy is often indicated in a toxic myopathy if it is necessary to exclude other causes of myopathy (ie, inflammatory myopathy), and confirm the presence of myopathy. If the myopathy is mild, the muscle biopsy may be normal. A wide variety of histologic changes may be seen depending on the mechanism of the myotoxicity (Table 42-7).
Histologic Changes in Toxic Myopathies
|
Metabolic myopathies result from a disorder of muscle energy production. This may result from a mitochondrial metabolism defect, or from cytoplasmic lipid or carbohydrate/glycogen problems. Although the majority of patients with metabolic myopathy have a genetic disorder, no family history may be apparent.
Patients often report symptoms with exercise. Symptoms include cramps, exercise intolerance, myalgias, and hemoglobinuria. A metabolic myopathy should be considered in the differential diagnosis of painful myalgias.
In addition, other associated findings raise the possibility of a metabolic myopathy:
Weakness of the extraocular muscles with ptosis
Respiratory weakness
Systemic involvement
Cardiomyopathy
Endocrinopathy
Brain involvement
Stroke
Encephalopathy
Seizures
Hypotonia (in infants and young children)
Metabolic myopathies are categorized by the cellular location and type of the underlying metabolic defect. Onset in both childhood and adulthood may be seen for this group of disorders, often with a different degree of severity and different prognosis. Disorders of carbohydrate/glycogen metabolism, lipid metabolism, and mitochondrial metabolism will be discussed in turn.
Muscle may use multiple sources of energy. During muscle activity, glycogen is broken down (glycogenolysis) and the liberated glucose enters the glycolytic pathway (Figure 42-1). Disorders of glycogenolysis include the following:
Pompe disease has infantile, juvenile-onset, and adult-onset forms. The combined incidence in the United States is 1:40,000, and it is more common in African Americans. The adult-onset form is the most likely to present undiagnosed and is the only form discussed in this review. Usual onset is in the third or fourth decade, with generalized proximal greater than distal weakness. Half of the patients complain of pain. Respiratory muscle involvement with hypoventilation is frequent and causes most mortality related to the disease. Hepatomegaly and cardiomegaly do not usually occur in the adult-onset form; however, abnormal cardiac conduction may occur. Osteoporosis is an associated condition.
McArdle disease causes exercise intolerance. Onset is usually in the first and second decades of life, but the diagnosis may not be made until adulthood. Early in exercise the patient has weakness and stiffness with muscle pain. If severe, the stiffness results in a muscle contracture. This phase of exercise uses anaerobic metabolism from glycogenolysis and glycolysis as the only source of energy. If the patient then continues to exercise, they may report a “second wind,” corresponding to the onset of aerobic fatty acid oxidation. In McArdle disease, there is muscle damage occurring during exercise. Muscle pain occurs prior to myoglobinuria, so patients can learn to avoid the episodes. The recurrent myoglobinuria may eventually cause renal failure.
The muscle is also able to use lipids as an energy source. A lipid metabolism defect may be primary or secondary to systemic disease (malnutrition, chronic hemodialysis) or medication (ie, zidovudine).
Primary disorders of lipid metabolism include the following:
The adult-onset myopathic form of CPTII deficiency is the most common cause of hereditary myoglobinuria and is the most common form of lipid metabolic disorder. The only known gene associated with the condition is CPT2.37 The disorder is autosomal recessive, so there is often no family history unless there are many siblings or consanguity. The disease causes recurrent bouts of myoglobinuria with myalgias triggered by prolonged exercise, especially if the patient was fasting. In the adult form, hepatic involvement is not present.
Mitochondrial proteins are partly coded in mitochondrial DNA (mtDNA) and partly in nuclear DNA. Etiologies of mitochondrial disease include:
Defects of the nuclear genome affecting
Mitochondrial protein subunits
Transport of proteins into the mitochondria
Regulation of mitochondrial genome function
Defects of the mitochondrial genome
Mitochondrial protein subunits
Acquired defects of mitochondrial function
Multiple energy pathways converge on the mitochondria. Pyruvate from glycolysis enters the mitochondria to enter the Krebs cycle. Fatty acids cross into the mitochondria to undergo β-oxidation, and the resulting acetyl-CoA also enters the Krebs cycle. The resulting NADH2 and FADH2 allow oxidative phosphorylation to regenerate ATP from ADP via the electron transport chain.
Of these pathways, disorders of oxidative phosphorylation cause the features typical of mitochondrial myopathies. A schematic of oxidative phosphorylation is shown in Figure 42-2. Oxidative phosphorylation occurs along the inner mitochondrial membrane and oxidizes the NADH2 and FADH2 from the Krebs cycle to regenerate ATP from ADP. The respiratory chain subunits Complexes I–IV pass electrons down an energy gradient while pumping protons across the mitochondrial inner membrane. This produces a gradient that allows complex V to phosphorylate ADP to ATP. Of the protein subunits in the chain, 13 are encoded by mtDNA, including components of NADH-ubiquinone reductase, cytochrome C reductase, cytochrome C oxidase, and ATP synthetase. The remainder are encoded by nuclear DNA, as are all the regulatory proteins of the mitochondria. Importation of proteins into the mitochondria is controlled by the cell nucleus. In addition, mtDNA encodes 2rRNAs and 22 tRNAs. MtDNA is unique in the body; the mtDNA has a circular form and does not have the normal repair mechanisms present in cell nuclei, leading to a high mutation rate. Every cell has multiple mitochondria, with a copy number of 8–10 mtDNA in each mitochondrion. There is variability of the mtDNA even within a single muscle fiber and also between tissues (heteroplasmy). One implication of this is that leukocyte mtDNA may not reflect the mtDNA content of the skeletal muscles. Inheritance of mtDNA is solely maternal.
Some mitochondrial syndromes that affect muscle result from specific mutations; however, some may result from multiple mutations. Specific diagnoses are discussed below.
CPEO is a very common manifestation of mitochondrial myopathy. There is restriction of eye movements in all directions of gaze, with or without eyelid ptosis. When present, ptosis is usually bilateral. The patient typically has no diplopia and often cannot identify onset, as the onset is insidious and often asymptomatic. Associated symptoms may be myopathy of the limb muscles, cardiac conduction deficits, short stature, sensorineural hearing loss (SNHL), endocrine abnormalities, other cranial neuropathies, and cerebellar ataxia. Pigmentary retinopathy with visual field peripheral deficits may be present. CSF protein is often elevated.
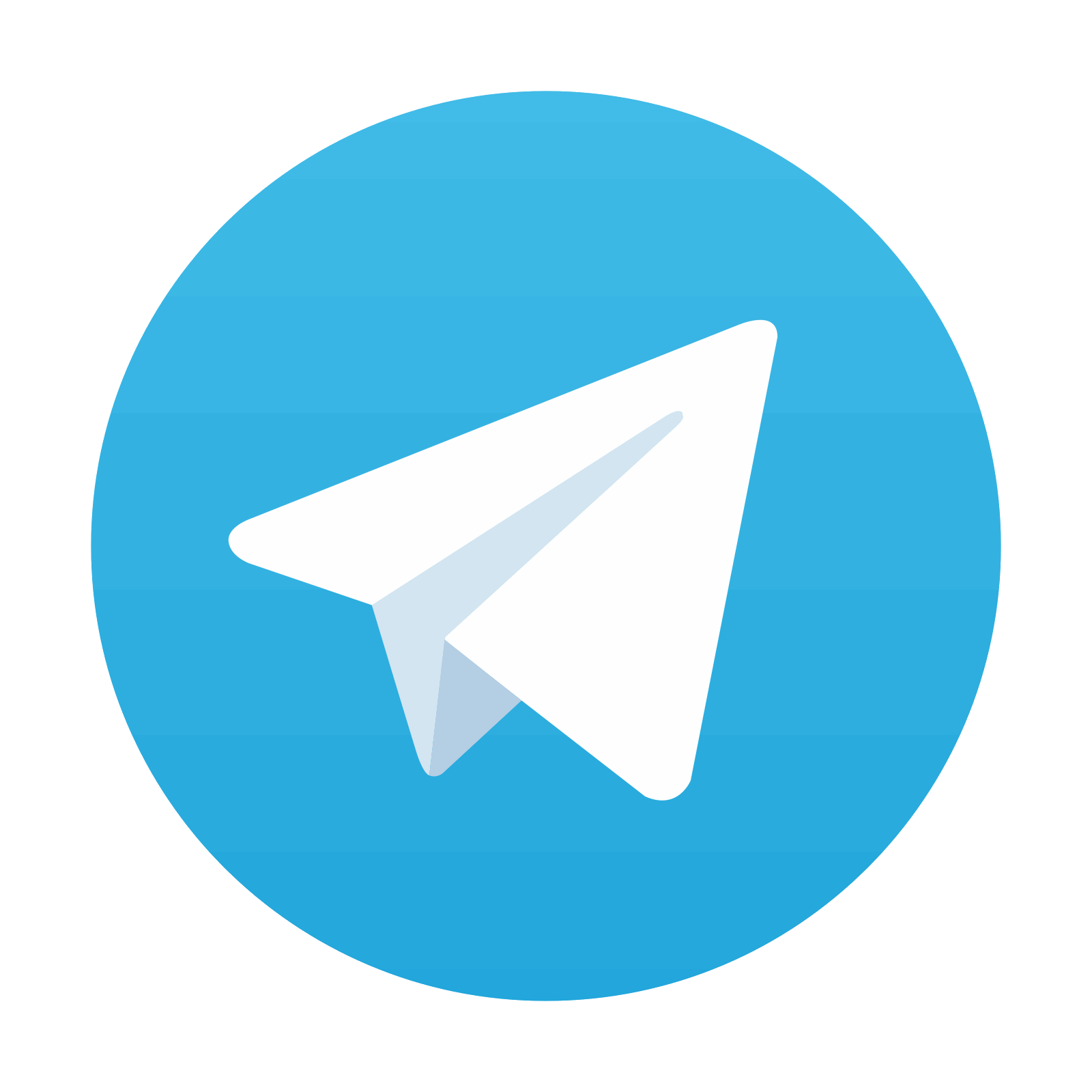
Stay updated, free articles. Join our Telegram channel

Full access? Get Clinical Tree
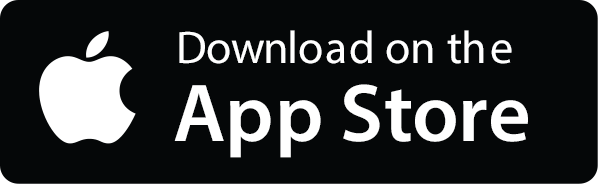
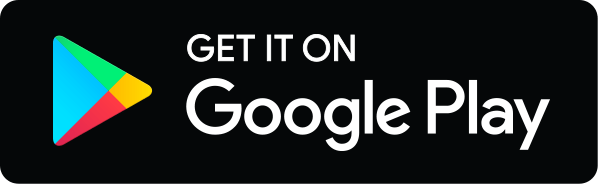