C5
C6
C7
C8
T1
Muscles groupsa
Elbow flexors
Wrist extensors
Elbow extensors
Finger flexors to the middle finger
Small finger abductors
Personal independenceb
Type, feed
Drink, wash, shave, dress upper body
Turn in bed, dress lower body
Bladder and bowel care
Wheelchair managementb
Manipulate brake, push on the flat
Remove armrests/footplates
Wheel over uneven ground
Negotiate curbs
Balance on rear wheels
Transfersb
From chair to bed or car
From chair to toilet or chair or bath
From chair to bath
From chair to floor
The time course of spontaneous motor recovery after a traumatic cervical sensorimotor-complete SCI is well known from various study populations. After 1 year, the average recovery amounts to 10–11 out of 50 bilateral upper extremity motor points [13, 160–162]. This is independent of cervical lesion level [162]. Cervical SCI patients with facet dislocation tended to present with a more severe degree of initial injury and displayed less potential for motor recovery at a 1-year follow-up [67]. Yet, it is important to note that a change in motor score cannot necessarily be taken as an indication of a gain in lesion level or functional independence. The distribution of gained motor points may be functionally more important than a given sum of improved motor points. Similarly, recovery of function is not exclusively dependent on recovery of motor score. Following traumatic SCI, there are no age-related differences in the recovery of motor score but significant age effects on the level of functioning [157, 163, 164]. The likelihood for a gain of one or two motor segments, which means a highly relevant improvement for a patient suffering from a complete cervical SCI, is 29–41 % and 29–33 %, respectively (Fig. 4.1), taking into account the data from two large cohort studies with more than 2600 patients together [162]. On the contrary, after 1 year, 60–70 % of subjects spontaneously recovered one motor level. Furthermore, these motor level changes were only moderately related to gains in motor score. However, a change of two motor levels in a cervical complete SCI was related to meaningful functional improvement as expressed by validated functional outcome scores [165], suggesting that careful tracking of cervical motor recovery outcomes and segmental changes may provide necessary sensitivity and accuracy to reliably detect significant and meaningful recovery of function or potentially as the equivalent of a significant treatment effect in this group of SCI patients [162]. Motor recovery in these patients showed a very characteristic time course with major improvement occurring within the first 12 weeks after SCI (Fig. 4.2). While these numbers are suggestive of characteristic recovery profiles, it has to be kept in mind that despite the rather large database these numbers are still too low to obtain recovery profiles in relation with lesion level.



Fig. 4.1
Proportion of individuals with an initial C5–C7 motor level spontaneously deteriorating, remaining stable, or gaining motor levels from baseline to different time points over the first year after cervical AIS-A SCI [162]. The percentage of individuals in each category of motor level change or stability at 1 year after SCI is shown from (a) Sygen; (b) EMSCI [162]

Fig. 4.2
Upper Extremity Motor Score (UEMS) during spontaneous recovery in the EMSCI and Sygen databases. (a–d), initial motor level of C4, C5, C6, and C7, respectively. (Error bars = 95 % confidence intervals) [162]
A motor level deterioration is much more rare (4.6 %), is more prevalent in lower cervical lesions (C6 or C7), and usually occurs within the first 4–8 weeks. Other than in the cervical complete SCI, thoracic lesions do not show a similar motor improvement, most likely because they are not accessible by the standardized neurological (ISNCSCI) and functional (Spinal Cord Independence Measure; SCIM) tests [159]. Segmental changes in the thoracic region will be expressed exclusively in sensation changes in the ISNCSCI exam, and while trunk stability, arm force, and respiratory function may be better, the lower the level of thoracic lesion, this is not truthfully reflected by common clinical or functional standardized scores.
4.4.2 Sensory Recovery
Sensory improvement in traumatic SCI is poor. Only small significant improvements were found with differences between tetraplegic and paraplegic subjects but not between complete and incomplete SCI [12]. In a population of 460 acute traumatic patients, the light touch sensation increased significantly in tetraplegic AIS A and C subjects, whereas light touch sensation on average of all tested segments remained unchanged in most paraplegic patients. Pinprick was even more stable and only increased moderately in tetraplegic AIS C patients. In this context, it must be kept in mind that the course scale of pinprick and light touch (LT) assessment according to ISNCSCI may not be sufficiently sensitive and responsive to detect subtle changes during the course of recovery or in a clinical trial. For that reason there was much interest in introducing a methodology which would allow sensory segmental testing on a more sensitive parametric scale. In order to overcome these shortcomings of LT testing, the electrical perception threshold (EPT) was introduced as a highly standardized and scalable measure [166] and validated in the healthy and a group of SCI patients [166–168]. According to these studies, it was possible to obtain reference values for each dermatome between cervical 3 (C3) and sacral 2 (S2) and age- and gender-specific nomograms. The EPT was shown to add sensitivity and resolution to the standard clinical testing as it was possible to show specifically the reliability of the EPT for each individual dermatome in healthy participants and the sensitivity of the EPT in detecting changes over time (i.e., responsiveness) in patients with SCI [168]. Normal values for each segment are thus available introducing objective and standardized sensory testing into SCI assessment with anatomical nomograms and demonstrating minimal or no segmental change over time in complete and incomplete SCI patients at and below the level of lesion. Thus, irrespective of the type of assessment, very little spontaneous recovery of sensory perception can be found caudal to the lesion in SCI. The reason for this difference between the motor and sensory adaptations seen after an acute SCI is unknown (Figs. 4.3 and 4.4).



Fig. 4.3
Course of normalized electrical perception threshold (EPT) within the first 6 months in sensory-incomplete tetraplegia: Average EPT values at 5, 2, and 1 levels above the neurological lesion; at level; and at 1, 2, and 5 levels below the neurological level of lesion. The averages are presented for 1, 3, and 6 months after initially sensory-complete paraplegia. Normalized EPT values above 1 indicate pathological EPT values. Abbreviations: N number of participants, EPT electrical perception threshold, normalized EPT is the recorded EPT value in spinal cord injury patients divided by the EPT value corresponding to the upper limit of the 95 % confidence interval of the recorded EPT value in healthy participants in the dermatome being tested. *P ≤ .05, **P ≤ .01 [168]

Fig 4.4
Course of normalized electrical perception threshold (EPT) within the first 6 months in sensory-complete paraplegia: Average EPT values at 5, 2, and 1 levels above the neurological lesion; at level; and at 1, 2, and 5 levels below the neurological level of lesion. The averages are presented for 1, 3, and 6 months after initially sensory-complete paraplegia. EPT norm values above 1 indicate pathological EPT values. Abbreviations as in Fig. 4.3. [168]
4.5 Outlook
As was discussed in this chapter, motor and functional recovery begin in parallel with cessation of spinal shock and increasing muscle tone following an acute SCI. Characteristic recovery curves for dedicated subpopulations have been obtained from large databases pooling information on SCI according to standardized protocols. For traumatic SCI these have informed health professionals about the typical time course of recovery of sensory and motor function below the level of lesion. Similar data is missing for nontraumatic etiologies, however. Recovery mechanisms as described in the previous paragraphs must thus be understood in the context of traumatic SCI only. Knowledge about the course of natural recovery may be useful to help design and evaluate incipient clinical trials to improve recovery in traumatic SCI. A key issue in this context is to distinguish treatment effects from natural recovery, in order to deal with the difficulty of determining potential efficacy of an intervention. In this context, knowledge of the disease course may not be sufficient, but additional assessment may be required, e.g., of underlying neurophysiological changes or surrogate markers such as MRI studies to fully characterize adaptations subsequent to a traumatic SCI in order to distinguish true treatment effects. This may help in the process of trial design and therapy development. Stratification and prediction strategies are crucial in this process as will be pointed out further in a later chapter.
References
1.
Hughes JT (1988) The Edwin Smith Surgical Papyrus: an analysis of the first case reports of spinal cord injuries. Paraplegia 26(2):71–82PubMed
2.
Breasted JH (1930) Edwin smith surgical papyrus in facsimile and hieroglyphic transliteration with translation and commentary. University of Chicago Oriental Institute Publications, Chicago
3.
Guttmann L (1976) Spinal cord injuries. Comprehensive management and research, 2nd edn. Blackwell Science Ltd, London/England, p 768
4.
Silver JR (2005) History of the treatment of spinal injuries. Postgrad Med J 81(952):108–114PubMedPubMedCentral
5.
McDermott W, Rogers DE (1982) Social ramifications of control of microbial disease. Johns Hopkins Med J 151(6):302–312PubMed
6.
Middleton JW et al (2012) Life expectancy after spinal cord injury: a 50-year study. Spinal Cord 50(11):803–811PubMed
7.
Shavelle RM et al (2015) Improvements in long-term survival after spinal cord injury? Arch Phys Med Rehabil 96(4):645–651PubMed
8.
Strauss DJ et al (2006) Trends in life expectancy after spinal cord injury. Arch Phys Med Rehabil 87(8):1079–1085PubMed
9.
Ghosh A et al (2009) Functional and anatomical reorganization of the sensory-motor cortex after incomplete spinal cord injury in adult rats. J Neurosci 29(39):12210–12219PubMed
10.
Raineteau O et al (2002) Reorganization of descending motor tracts in the rat spinal cord. Eur J Neurosci 16(9):1761–1771PubMed
11.
Bracken MB et al (1990) A randomized, controlled trial of methylprednisolone or naloxone in the treatment of acute spinal-cord injury. Results of the Second National Acute Spinal Cord Injury Study. N Engl J Med 322(20):1405–1411PubMed
12.
Curt A et al (2008) Recovery from a spinal cord injury: significance of compensation, neural plasticity, and repair. J Neurotrauma 25(6):677–685PubMed
13.
Geisler FH et al (2001) Measurements and recovery patterns in a multicenter study of acute spinal cord injury. Spine (Phila Pa 1976) 26(24 Suppl):S68–S86
14.
Marino RJ et al (1999) Neurologic recovery after traumatic spinal cord injury: data from the model spinal cord injury systems. Arch Phys Med Rehabil 80(11):1391–1396PubMed
15.
Kirshblum SC, O’Connor KC (2000) Levels of spinal cord injury and predictors of neurologic recovery. Phys Med Rehabil Clin N Am 11(1):1–27, viiPubMed
16.
Kirshblum S, Waring W 3rd (2014) Updates for the international standards for neurological classification of spinal cord injury. Phys Med Rehabil Clin N Am 25(3):505–517, viiPubMed
17.
Marino RJ et al (2003) International standards for neurological classification of spinal cord injury. J Spinal Cord Med 26(Suppl 1):S50–S56
18.
Frankel HL et al (1969) The value of postural reduction in the initial management of closed injuries of the spine with paraplegia and tetraplegia. I. Paraplegia 7(3):179–192PubMed
19.
Kirshblum S et al (2011) The impact of sacral sensory sparing in motor complete spinal cord injury. Arch Phys Med Rehabil 92(3):376–383PubMedPubMedCentral
20.
Waters RL, Adkins RH, Yakura JS (1991) Definition of complete spinal cord injury. Paraplegia 29(9):573–581PubMed
21.
Kirshblum S et al (2004) Late neurologic recovery after traumatic spinal cord injury. Arch Phys Med Rehabil 85(11):1811–1817PubMed
22.
Dietz V, Curt A (2006) Neurological aspects of spinal-cord repair: promises and challenges. Lancet Neurol 5(8):688–694PubMed
23.
McKinley W et al (2007) Incidence and outcomes of spinal cord injury clinical syndromes. J Spinal Cord Med 30(3):215–224PubMedPubMedCentral
24.
Kumral E et al (2011) Spinal ischaemic stroke: clinical and radiological findings and short-term outcome. Eur J Neurol 18(2):232–239PubMed
25.
Bosch A, Stauffer ES, Nickel VL (1971) Incomplete traumatic quadriplegia. A ten-year review. JAMA 216(3):473–478PubMed
26.
Nathan PW (1994) Effects on movement of surgical incisions into the human spinal cord. Brain 117(Pt 2):337–346PubMed
27.
Schneider RC (1955) The syndrome of acute anterior spinal cord injury. J Neurosurg 12(2):95–122PubMed
28.
Molliqaj G et al (2014) Acute traumatic central cord syndrome: a comprehensive review. Neurochirurgie 60(1–2):5–11PubMed
29.
Schneider RC, Cherry G, Pantek H (1954) The syndrome of acute central cervical spinal cord injury; with special reference to the mechanisms involved in hyperextension injuries of cervical spine. J Neurosurg 11(6):546–577PubMed
30.
Lemon RN et al (2004) Direct and indirect pathways for corticospinal control of upper limb motoneurons in the primate. Prog Brain Res 143:263–279PubMed
31.
Bernhard CG, Bohm E (1954) Monosynaptic corticospinal activation of fore limb motoneurones in monkeys (Macaca mulatta). Acta Physiol Scand 31(2–3):104–112PubMed
32.
Kuypers HG (1978) The motor system and the capacity to execute highly fractionated distal extremity movements. Electroencephalogr Clin Neurophysiol Suppl 34:429–431
33.
Wolpert DM, Ghahramani Z, Flanagan JR (2001) Perspectives and problems in motor learning. Trends Cogn Sci 5(11):487–494PubMed
34.
Brown-Sequard CE (1868) Lectures on the physiology and pathology of the central nervous system and the treatment of organic nervous affections. Lancet 2:593–595, 659–662,755–757,821–823
35.
Little JW, Halar E (1985) Temporal course of motor recovery after Brown-Sequard spinal cord injuries. Paraplegia 23(1):39–46PubMed
36.
Rosenzweig ES et al (2009) Extensive spinal decussation and bilateral termination of cervical corticospinal projections in rhesus monkeys. J Comp Neurol 513(2):151–163PubMedPubMedCentral
37.
Rosenzweig ES et al (2010) Extensive spontaneous plasticity of corticospinal projections after primate spinal cord injury. Nat Neurosci 13(12):1505–1510PubMedPubMedCentral
38.
Dietz V (2002) Proprioception and locomotor disorders. Nat Rev Neurosci 3(10):781–790PubMed
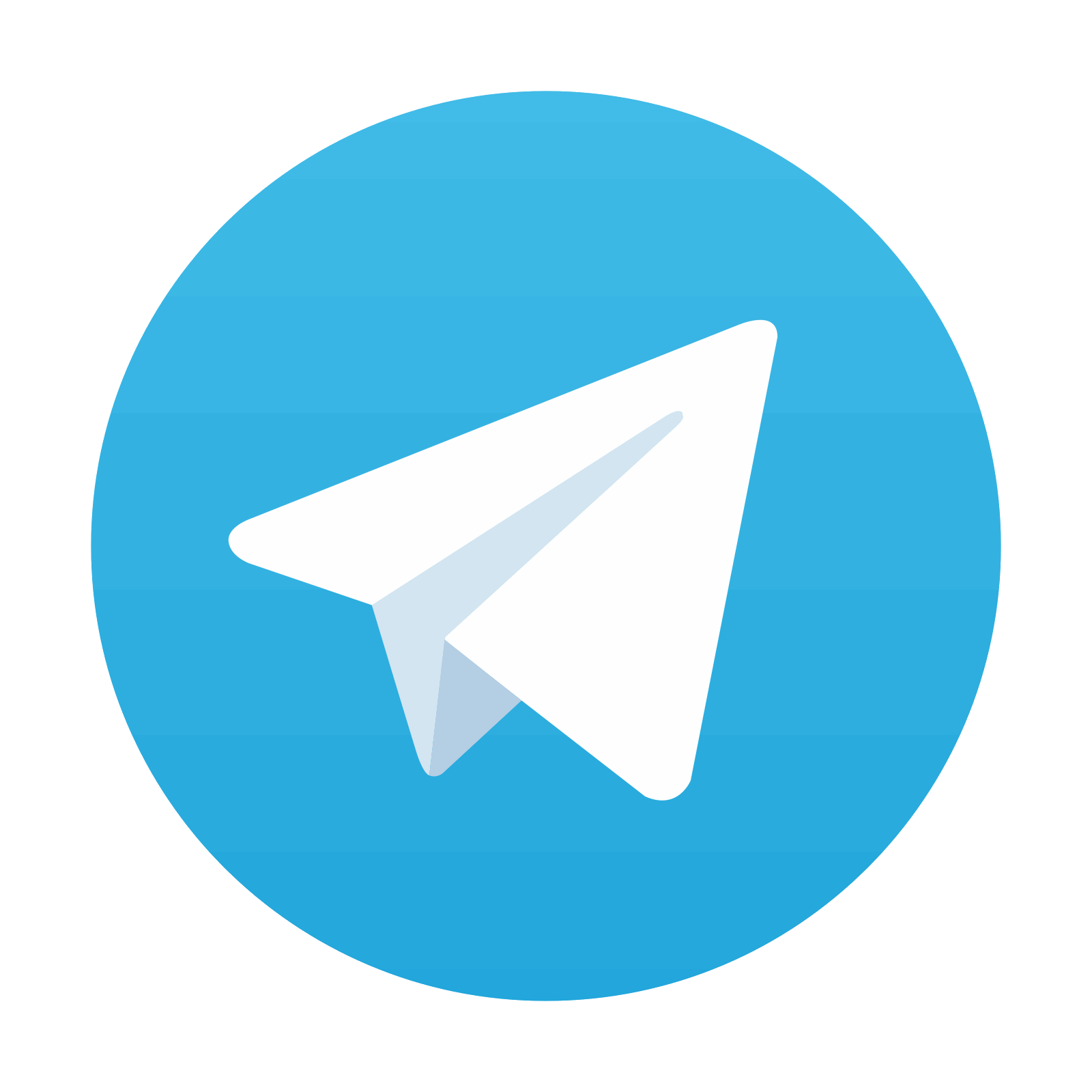
Stay updated, free articles. Join our Telegram channel

Full access? Get Clinical Tree
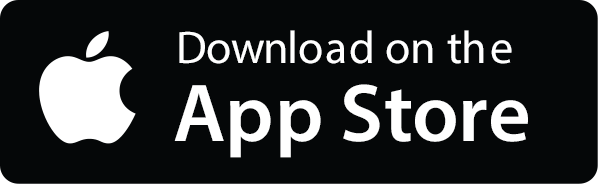
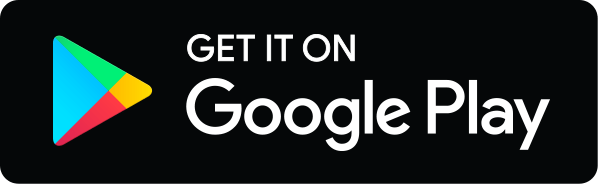