9 Natural History and Management Options of Traumatic Brain Injury
Stephen Honeybul
Abstract
There have been considerable advances in knowledge regarding the basic science of the complex cellular response to trauma, and this has challenged the traditional classification of primary and secondary brain injury. It is also becoming increasingly apparent that TBI is a very heterogeneous disease process with areas of ischemia coexisting with mass lesions, contusions, areas with blood-brain barrier disruption as well as areas where the brain parenchyma is relatively normal. The optimal management for each type of brain injury will not necessarily be the same, and there are significant limitations when attempting to apply blanket therapies across all types of TBI. What remain to be established are either new or improved therapeutic strategies that convert the basic science and clinical information gained into clinical benefit and this is the challenge in the years ahead.
Keywords: traumatic brain injury hypothermia barbiturate coma decompressive craniectomy outcome prediction
9.1 Introduction
Traumatic brain injury (TBI) is a global health care problem that consumes significant clinical and research resources, and the medical and surgical management strategies continue to evolve. From a clinical perspective, there have been significant improvements in data gathering and statistical analysis that have enabled researchers to develop sophisticated web-based prediction models that can be used to stratify patients according to injury severity and provide clinically useful information regarding prognosis.1, 2 In addition, the continued development of sophisticated intracranial monitoring techniques has provided a considerable amount of data regarding the developing secondary brain injury.3, 4 Finally, the results of several recent prospective multicenter randomized controlled trials have provided important information to guide clinical practice.5, 6, 7, 8, 9, 10, 11
In addition to these clinical developments, there have been considerable advances in knowledge regarding the basic science of the complex cellular response to trauma and this has challenged the traditional classification of primary brain injury, which occurs at the initial trauma, and the secondary injury, which follows in the hours and days thereafter. It is now realized that there is considerable overlap between these two processes and a substantial amount of cell death is due to a series of deleterious neurochemical cascades that are initiated at the time of injury and are amplified by secondary insults such as hypoxia or hypotension.12, 13 It is also becoming increasingly apparent that TBI is a very heterogeneous disease process with areas of ischemia coexisting with mass lesions, contusions, areas with blood–brain barrier disruption, as well as areas where the brain parenchyma is relatively normal. The optimal management for each type of brain injury will not necessarily be the same, and there are significant limitations when attempting to apply blanket therapies across all types of TBI.
What remains to be established are either new or improved therapeutic strategies that convert the basic science and clinical information gained into clinical benefit, and this is the challenge in the years ahead.
9.2 Selected Papers on the Natural History of Traumatic Brain Injury
●Mollayeva T, Kendzerska T, Mollayeva S, Shapiro CM, Colantonio A, Cassidy JD. A systematic review of fatigue in patients with traumatic brain injury: the course, predictors and consequences. Neurosci Biobehav Rev 2014;47:684–716
●Hicks AJ, Gould KR, Hopwood M, Kenardy J, Krivonos I, Ponsford JL. Behaviours of concern following moderate to severe traumatic brain injury in individuals living in the community. Brain Inj 2017;31(10):1312–1319
9.3 Natural History of Traumatic Brain Injury
The traditional classification of TBI severity is divided into mild, moderate, and severe, based on the initial Glasgow coma scale (GCS). Mild is classified as a GCS of 13 to 15, moderate as 9 to 12, and severe as less than 8. Notwithstanding some limitations of this classification, it does serve as a useful framework in which to consider the long-term outcome. There is, however, wide variation in the incidence of posttraumatic symptoms ranging from complete recovery in most patients to high rates of chronic problems.14, 15, 16 This variation has been reported across all levels of injury severity and whereas this may reflect a lack of standardization when considering timing of assessment, symptom description, and a universally accepted definition of outcome, it may equally reflect the heterogeneity of the disease process.
Headache is one of the most common persisting symptoms with reported incidence ranging from 30 to 90%.17 The mechanism of headache remains poorly understood; however, the presence of premorbid headaches appears significant. Indeed, the presence of a premorbid symptom is an important predictor of several posttraumatic symptoms, and the posttraumatic exacerbation of that particular symptom, such as headaches, may merely be a reflection of reduced tolerance brought on by the brain injury.
Fatigue is another common long-standing problem that can have a very negative effect on social, physical, and cognitive function after TBI; however, as with other posttraumatic symptoms, there is a wide range of reported prevalence from as low as 21% to as high as 73%.14 This wide prevalence is perhaps unsurprising given the difficulties inherent in defining “fatigue.” For example, “physiological fatigue” refers to a state of general tiredness due to physical or mental exertion, which can be ameliorated by rest, whereas “pathological fatigue” refers to a weariness that is unrelated to previous exertion and is not ameliorated by rest. These difficulties in symptom definition are further compounded when considering the numerous possible causes of fatigue, which may include any combination of neuroanatomical, functional, psychological, biochemical, or endocrine dysfunction. Notwithstanding several comprehensive reviews into this topic, there remains relatively limited knowledge regarding specific clinical and pathophysiological factors that predispose to posttraumatic fatigue, its natural history, and the overall health care burden of this symptom.
The same can be said of many other reported posttraumatic symptoms such as sleep dysfunction, depression, physical and cognitive impairment, and overall medical and neurological dysfunction, all of which can have a significant impact on an individual’s long-term recovery.16, 17
Finally, one of the most challenging aspects of recovery from moderate to severe TBI is the development of behavioral changes that can continue after the acute phase of recovery, becoming chronic and thereafter persistent.18 These so-called behaviors of concern (BoCs) cover a broad spectrum ranging from apathy and social withdrawal to more challenging behaviors such as disinhibition, sexually inappropriate, aggressive, and violent behavior patterns.
There are likely to be many factors that contribute to the BoCs, but it is becoming increasingly apparent that not only do these issues persist in many individuals but they can also worsen over time and may be exacerbated when individuals become frustrated as they attempt to reintegrate into family and community life. These individuals place a significant strain on community health care resources, and recent studies investigating this issue have highlighted the lack of resources available among community-based adults who have survived many years with these issues.18
Overall, the long-term natural history of TBI remains difficult to predict on an individual basis and further work is required in order to gain consensus regarding issues such as precise symptom definition, timelines for assessment, and validated measures of outcome. It would also be useful to make any early determination regarding which individuals are likely to develop long-term problems and it is in this regard that more sophisticated outcome prediction models may provide some benefit.
9.4 Predicting Outcomes Following Traumatic Brain Injury
Predicting long-term outcome following TBI is important for several reasons, not least of which is the need to counsel families regarding what to expect in terms of functional recovery and the likelihood or otherwise of return of their loved one back into family life and the working environment. In addition, prognosis must be important when considering the continuation or indeed withdrawal of intensive care and neurosurgical therapy. Finally, the wider implications of equitable and sustainable use of resources need to be considered, not only in the acute phase of intervention but also when considering allocation of scarce rehabilitation facilities. Notwithstanding some limitations, prognostically important information can be obtained from the severity of the initial brain injury and thereafter the severity of the developing secondary brain injury.
9.5 Assessment of the Primary Brain Injury
Traditionally, the GCS has been used as an indicator of the severity of the initial injury, as well as clinical variables such as age, pupillary reaction, presence of extracranial injuries, and certain radiological features on initial computed tomography scan. More recently, the availability of increasing amounts of accurate baseline data, combined with advances in statistical analysis, has enabled researchers to develop sophisticated web-based prediction models that provide a mathematical calculation of a prognostic risk.1, 2 The CRASH (Corticosteroid Randomization after Significant Head Injury) collaborators’ model was developed from the data collected on the 10,000 patients in the CRASH trial that investigated whether steroids were beneficial following TBI, and included mild, moderate, and severe TBI.19 The clinical predictive variables required for the CRASH model are age, postresuscitation GCS score, pupillary response, and presence of a major extracranial injury. The radiological predictive variables are the presence of petechial hemorrhage, subarachnoid blood, midline shift, nonevacuated hematoma, and obliteration of the basal cisterns.
The IMPACT (International Mission for Prognosis and Clinical Trial) model was developed from 8,509 patients and focused on patients with moderate and severe TBI.2 The baseline presentation data differ slightly from that of the CRASH model. Only the motor function of the GCS is required, hypoxia and hypotension are recorded separately, and epidural mass is recorded as a separate radiological finding. The model also uses the Marshall radiological classification and incorporates the prognostic significance of presentation blood glucose and hemoglobin levels.
Both models are Web based and they provide a percentage risk of unfavorable outcome at 6 months. Unfavorable outcome is defined by the Glasgow Outcome Score (GOS) of severely disabled, vegetative, or dead.20 Several studies have demonstrated how the prediction of an unfavorable outcome may be used as a surrogate index of injury severity with which patients can be stratified according to injury severity.21, 22, 23 The authors of both models emphasize that the information provided should only be used to support and not replace clinical judgment; however, the data do provide an objective assessment of injury severity and can perhaps form the basis for ethical discussions when considering lifesaving but nonrestorative surgical intervention.24, 25
9.6 Assessment of Secondary Brain Injury
The use of intracranial pressure (ICP) monitoring has become widespread over the recent years not only to guide therapy but also to provide important prognostic information.26, 27 There is a strong correlation between outcome and the number of hours the ICP remains above 20 mm Hg, and this has been used as a threshold at which many medical therapies are introduced in a stepwise protocol-driven manner.28 However, notwithstanding the prognostic significance, one of the fundamental challenges in neurotrauma has been the inability to demonstrate that the reduction in ICP brought about by many of these therapies is necessarily translated into an improvement in clinical outcome.
Indeed, it is becoming increasingly apparent that whereas the ICP is a useful marker of the developing injury severity, it is essentially a marker of end organ injury, and reducing the absolute number by either medical or surgical means will not reverse the effects of the pathology that precipitated the rise in ICP. What is really needed is a more acute marker of the developing secondary brain injury and this has led to the development of several multimodal monitoring techniques such as microdialysis, brain tissue oxygenation, bedside measures of autoregulation (such as the pressure reactivity index or the mean index), and continuous electroencephalography monitoring.3, 4
Unfortunately, despite significant developments in the availability and use of these techniques, the clinical benefit gained from many of the recorded events remains undetermined. What is becoming increasingly apparent is that information obtained from a single modality that is interpreted independent from other physiological and metabolic parameters is unlikely to provide clinical benefit. In the same way that the CRASH and IMPACT models have combined individual prognostic indicators, what may now be required are advances in real-time, user-friendly data analysis and presentation that can be used to guide appropriately targeted individualized therapeutic management strategies. In addition, whereas the increasing complexity of monitoring technology must be matched with the appropriate clinical skills required to interpret the information gained, this is unlikely to improve outcome unless accompanied by new or improved therapies.
9.7 Selected Papers on the Treatment Outcomes
●Cooper DJ, Rosenfeld JV, Murray L, et al; DECRA Trial Investigators; Australian and New Zealand Intensive Care Society Clinical Trials Group. Decompressive craniectomy in diffuse traumatic brain injury. N Engl J Med 2011;364(16):1493–1502
●Hutchinson PJ, Kolias AG, Timofeev IS, et al; RESCUEicp Trial Collaborators. Trial of decompressive craniectomy for traumatic intracranial hypertension. N Engl J Med 2016;375(12):1119–1130
●Adelson PD, Wisniewski SR, Beca J, et al; Paediatric Traumatic Brain Injury Consortium. Comparison of hypothermia and normothermia after severe traumatic brain injury in children (Cool Kids): a phase 3, randomised controlled trial. Lancet Neurol 2013;12(6):546–553
●Andrews PJ, Sinclair HL, Rodriguez A, et al; Eurotherm3235 Trial Collaborators. Hypothermia for intracranial hypertension after traumatic brain injury. N Engl J Med 2015;373(25):2403–2412
9.8 Treatment Options
In general terms, the management of patients with mild and moderate TBI is supportive with an emphasis placed on the prevention of secondary brain injury due to hypoxia or hypotension combined with clinical surveillance to detect any neurological deterioration. The management strategies for these patients have changed little over the recent years; however, the management of severe TBI has evolved considerably.
9.9 Medical Management of Severe Traumatic Brain Injury
Management of TBI in the neurointensive care setting is targeted at optimizing cerebral perfusion and oxygenation with a view to minimizing secondary insults. Endotracheal intubation, sedation, and ventilation allow full control of cerebral perfusion and oxygenation, prevent agitation due to pain and distress, and facilitate ICP monitoring. The aim of ICP monitoring is to identify those patients who develop either surgical lesions requiring evacuation or progressive cerebral swelling, which would preclude from early weaning. This ICP-targeted approach also aims to maintain the cerebral perfusion pressure (mean arterial blood pressure minus the ICP) below a certain threshold using several interventions that are introduced in a stepwise protocol-driven manner.28
Once the ICP starts to rise above 20 mm Hg, initial management strategies include head elevation, further sedation and paralysis, cerebrospinal fluid (CSF) drainage, and osmotherapy with either mannitol or more recently hypertonic saline. These have been part of protocolized management for many years and continue to do so; however, many other therapies that once formed the cornerstone of neurointensive care management have had to be reevaluated in the light of recent clinical evidence.
For many years, patients who developed intracranial hypertension were routinely hyperventilated, placed in a barbiturate coma, or more recently rendered hypothermic.29, 30, 31, 32 The rationale was that lowering the ICP would improve cerebral perfusion, reduce secondary brain injury, and improve clinical outcome. However, despite numerous clinical studies demonstrating the use of these therapies in the management of raised ICP, none have demonstrated a conclusive improvement in outcome. Indeed, the recent randomized controlled trials investigating the role of hypothermia have shown a tendency to increased mortality in those patients randomized to the treatment arms of the trial.6, 7, 8, 9, 10, 11 The Hutchison et al pediatric trial showed a higher death rate and incidence of poor neurological outcome in the hypothermia group (23 of 108 [21%] patients in the hypothermia group died vs. 14 [12%] in the normothermia group).8 The National Acute Brain Injury Study: Hypothermia II (NABISH II) trial investigated early cooling within 2 hours of injury, and the outcomes were worse in the hypothermia group, although this was not statistically significant.9 The “Cool Kids” trial was stopped on the grounds of futility because hypothermia initiated early, used globally for 48 to 72 hours, and with a slow rewarming did not improve mortality at 3 months and again there was a tendency to increased mortality in the hypothermia group (6 of 39 [15%] patients in the hypothermia group vs. 2 [5%] in the normothermia group).10 Finally, the most recent multicenter trial by Andrews et al failed to show not only that hypothermia provided clinical benefit but also that there was a tendency to cause harm such that the trial also had to be halted early.11 Sixty nine of the 192 patients (36.5%) in the control group achieved a favorable outcome compared with 49 patients (25.7%) in the hypothermia arm of the trial. In addition, there was, again, an increased mortality in the hypothermia group (68 [34.9%] patients died vs. 51 [26.6%] in the normothermia group).
On face value, these findings would appear counterintuitive; however, studies investigating the effect that these three therapies have on cerebral blood flow indicate a probable reason. There is little doubt that barbiturates and hypothermia have the potential to be neuroprotective due to their influence on many aspects of the cellular response to injury, including calcium-mediated toxicity, glutamate excitotoxicity, free radical peroxidation, and cellular apoptosis.33, 34, 35 However, the mechanism by which these three therapies produce the often-rapid fall in ICP that occurs after their application is predominantly a result of cerebral vasoconstriction, and the subsequent reduction in cerebral blood flow has been clearly demonstrated by a number of studies.36, 37, 38 This does not necessarily mean that use of these therapies should be abandoned but rather their use should be far more judicious and should be generally reserved for intractable intracranial hypertension that is unresponsive to the aforementioned medical therapies. Their use must be tempered with the realization that any reduction in ICP may come at a cost of an increase in treatment morbidity and in these circumstances, consideration is often given to the use of decompressive craniectomy.
9.10 Surgical Management of Severe Traumatic Brain Injury
In some respects, the surgical management of severe TBI has changed over the recent years. The importance of early diagnosis of a clinically significant mass lesion such as an acute extradural, subdural, or parenchymal hematoma is well recognized and the need for expedient surgical evacuation is unquestioned. However, the past three decades have seen considerable debate regarding the use of decompressive craniectomy. The procedure itself is technically straightforward and first became popular in the management of severe TBI in the early 1970s. However, its use was almost abandoned in the latter part of that decade due to a combination of poor clinical outcomes and experimental studies that seemed to suggest that decompression may worsen cerebral edema.39, 40 Despite these early setbacks, interest in the procedure returned throughout the 1980s and 1990s, and there was a progressive increase in the number of publications reporting surgical efficacy of the procedure.41, 42, 43 Overall, there appeared to be little doubt that surgical intervention could reduce mortality; however, there has always been a concern that surgical intervention would convert death into survival with severe disability and dependency.39 In an attempt to address this issue, the last decade has seen two prospective multicenter randomized controlled trials investigating this issue.
9.11 Decompressive Craniectomy Following Severe Traumatic—not Hemicraniectomy Brain Injury
The DECompressive CRAniectomy (DECRA) study investigated the role of early bifrontal decompressive craniectomy in the context of diffuse cerebral swelling, and it demonstrated that outcomes were worse in those patients in the surgical arm of the trial.5 The results of the study evoked considerable debate, and one of the key criticisms was that the ICP threshold at which patients were randomised (20 mm Hg for more than 15 minutes in the hour) was not representative of current clinical practice (which is to intervene at higher ICP thresholds). While in hindsight, this may be a valid observation, it fails to acknowledge the trial hypothesis, which was that early decompression would improve cerebral perfusion, reduce secondary insults, and improve clinical outcome.
Given the enrolment at a relatively low ICP threshold, it is unsurprising that the trial did not demonstrate a survival benefit for those patients randomized to the surgical arm of the trial. However, the trial did clearly show that at that ICP threshold (20 mm Hg for > 15 min/h) there was insufficient ongoing secondary brain injury and therefore any potential benefit obtained from improved cerebral perfusion was offset by the now well-recognized surgical morbidity of the initial decompressive procedure and subsequent reconstructive cranioplasty. While the patients in the trial may not have been representative of current clinical practice, if the trial had shown benefit, these patients would have come to represent the clinical practice of the future and this would have had significant impact on neurosurgical resources.44
It is in this regard that the results of the recently published Randomized Evaluation of Surgery with Craniectomy for Uncontrollable Elevation of ICP (RESCUEicp) are particularly relevant as it was felt to be more reflective of current clinical practice.6 The trial compared last-tier secondary decompressive craniectomy with continued medical management in patients with a higher ICP threshold (25 mm Hg for 1–12 hours despite maximal medical treatment, except for barbiturates). It was conducted over a 10-year period between 2004 and 2014 and 409 patients were randomized among 2,008 eligible patients, at 52 centers in 20 countries. The trial demonstrated a clear survival benefit in those patients randomized to surgical decompression; however, this reduction in mortality came as an almost direct result of an increase in the number of survivors either in a vegetative state or with severe disability. At 12 months of follow-up, there was a small increase, albeit statistically insignificant in the number of patients with a favorable outcome from 34.6% in the medical arm of the trial to 42% (p = 0.12) in the surgical arm of the trial. However, this small increase was only possible by including patients with upper levels of severe disability within the favorable outcome category. Without this recategorization, the number of patients who survived with lower moderate disability or better (traditionally a favorable outcome) was very similar (32% favorable in the surgical arm of the trial and 28.5% favorable in the medical arm).
Overall, the results of these studies represent overwhelming evidence for efficacy of decompressive craniectomy as a lifesaving intervention; however, these recent studies clearly demonstrate that surgical intervention will not reverse the pathophysiology of the primary neurological insult and any reduction in mortality will come as a result of an increase in the number of survivors with significant disability.45
This raises several important ethical issues and the question remains as to the ongoing role of decompressive craniectomy and the direction of future research into clinical efficacy of the procedure. Given the significant reduction in mortality, it would appear unlikely that further randomized controlled trials comparing surgical decompression with standard medical therapy will be possible. Currently in most institutions, surgical intervention is usually performed when patients either have failed or are in the process of failing medical management, and randomizing patients in these circumstances is problematic as was clearly demonstrated in the RESCUEicp trial. However, there does appear to be a subset of patients who require surgical decompression, who survive and go on to make a good long-term recovery. In addition, some individuals seem to adapt to a level of disability and dependency that they might previously have thought to be unacceptable. The difficulty has always been how to accurately predict long-term outcome, and it is in this regard that observation cohort studies and the use of the aforementioned prediction models may be beneficial.
Over the recent years, several observational cohort studies in Western Australia have used the percentage prediction of unfavorable outcome provided by either the CRASH or IMPACT model as a surrogate index of injury severity with which to stratify patients according to injury severity.21, 22, 23, 46 Comparing the prediction of unfavorable outcome with the observed long-term outcome provides an objective assessment of the most likely outcome either for those patients who require surgical intervention to evacuate a mass lesion (primary decompression) or in those patients who develop intractable intracranial hypertension (secondary decompression; Fig. 9.1 and Fig. 9.2). Notwithstanding the limitations of applying population-based data to individual clinical cases, this type of objective assessment may act as a prompt to initiate discussions regarding realistic long-term outcome expectations.
Fig. 9.1 The CRASH (Corticosteroid Randomization after Significant Head Injury) collaborators’ prediction model. The prediction of an unfavorable outcome at 6 months (x axis) and the observed outcome at 18 months among the 319 patients for whom 18-month follow-up was available. Numbers within the bar chart represent absolute patient numbers. (Reproduced with permission of Elsevier from Honeybul S, Ho KM. Predicting long-term neurological outcomes after severe traumatic brain injury requiring decompressive craniectomy: a comparison of the CRASH and IMPACT prognostic models. Injury. 2016; 47(9):1886–1892.)
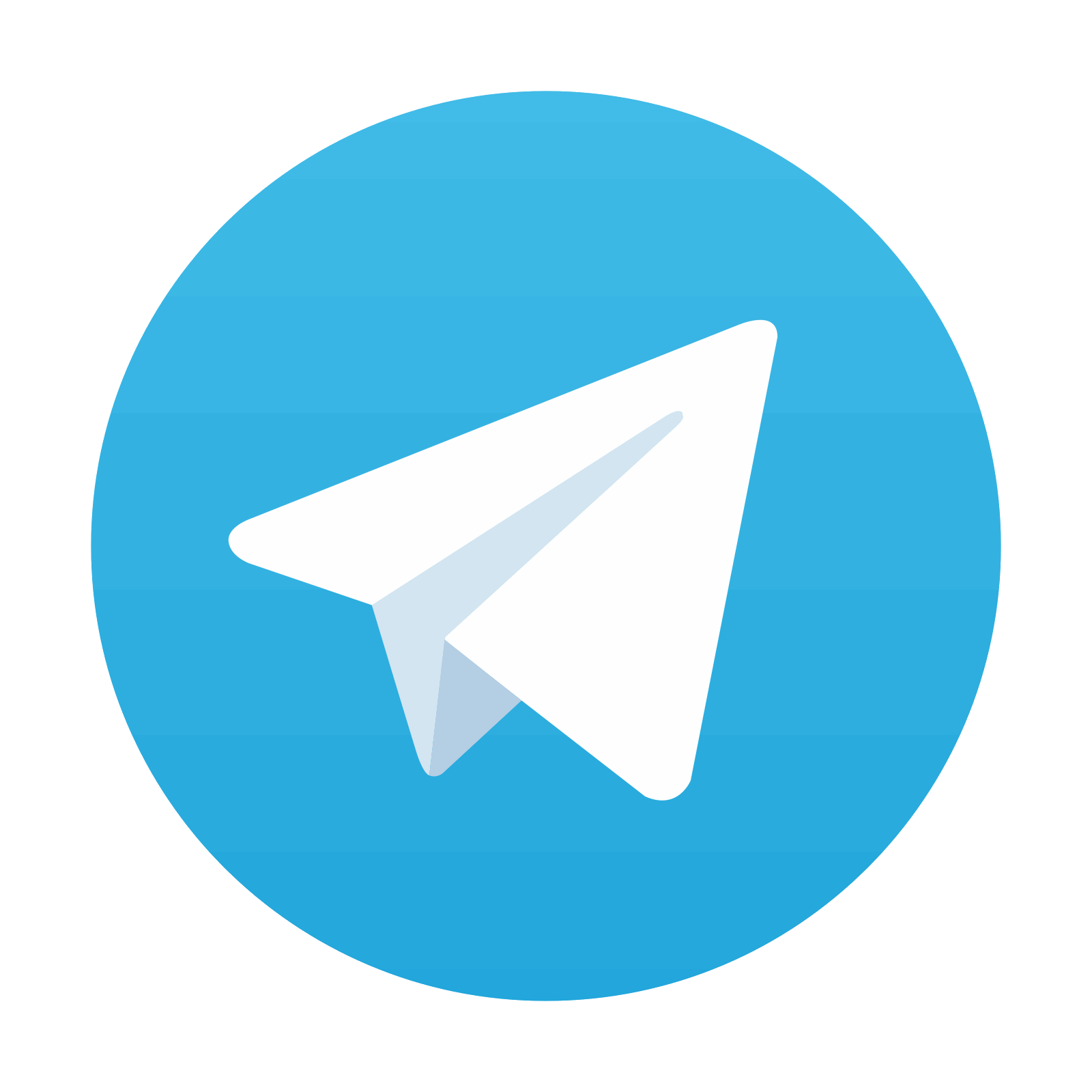
Stay updated, free articles. Join our Telegram channel

Full access? Get Clinical Tree
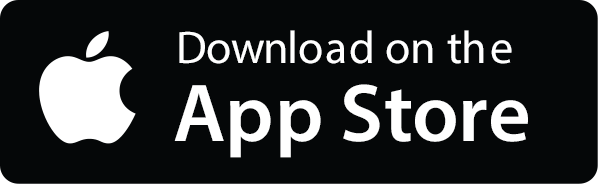
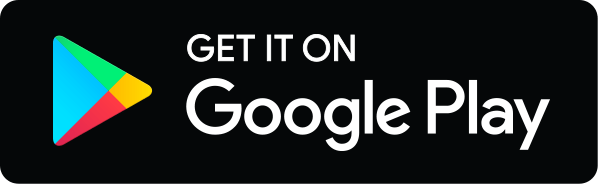