Fig. 1
Laser penetration profiles: (a) NILT penetration as a function of skull thickness in four species using a power density of 700 mW/cm2. NILT penetration decreased through the skulls of all four species utilized in this study as skull thickness increases. Presented as penetration, calculated based on the area of illumination and the amount of power utilized, (b) NILT penetration and scatter through the rabbit skull. Left, the red laser spotting beam penetrating the skull. Right, blue scatter through the skull. (c) NILT penetration as a function of power density: rabbit skull analysis
The photograph of the rabbit skull (Fig. 1, panel b, right) shows extensive scatter of laser light once the light has passed through the skull. Numerically, as shown in the line graph (Fig. 1a, b), there is >88 % attenuation of laser light passing from the surface to the interior measured using a Thorlabs power meter. As shown in (c), when using a power density of 200–700 mW/cm2 on the skull surface, it is estimated that the ventral surface of the skull has only achieved a power density of 26–85 mW/cm2, or approximately 11 % of the applied power. This level of attenuation must be considered when attempting to advance NITL therapy from animals to humans, especially because NILT has never been optimized in any animal species, nor has it been optimized for use in humans.
Using human calvaria, we completed similar measures of actual NILT penetration profiles to determine to what extent penetration was attenuated by the human skull. A sampling of data is presented in Fig. 2. As described above for the rabbit skull, there was extensive attenuation (>95 %) of laser light penetration through human calvaria, which was also measured using a Thorlabs power meter.
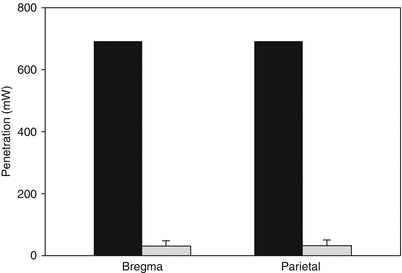
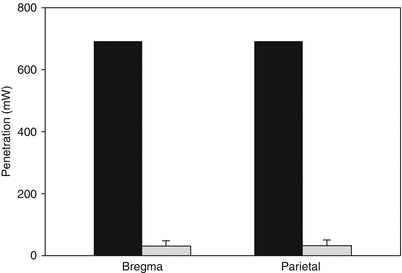
Fig. 2
Human calvaria laser penetration: NILT penetration through the dehydrated human calvaria measured at the bregma and the parietal skull. In both cases, approximately 4.5 % of laser light penetrated the skull bone
Using 18 human calvaria, we were able to accurately measure skull thickness and density by a new CT method described by Voie et al. [36]. We also conducted penetration analysis of every skull using a power density of 700 mW/cm2. With both data sets, we determined whether there was a correlation between laser light penetration and either skull thickness or density using Pearson correlation analysis. As shown in Fig. 3a, there was a positive correlation between decreased laser penetration and increased calvaria thickness (p = 0.0127), but there was no correlation between laser penetration and calvaria density (p = 0.9166).
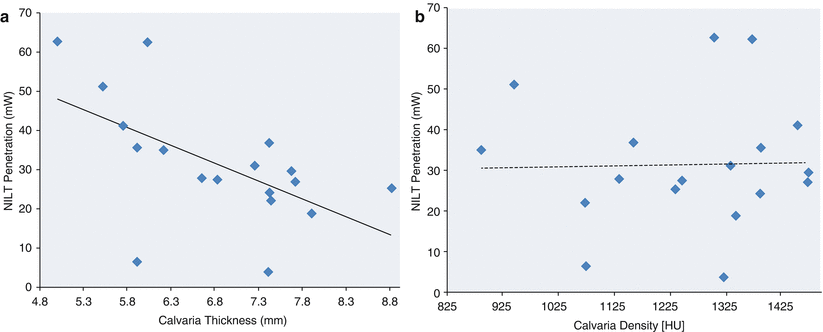
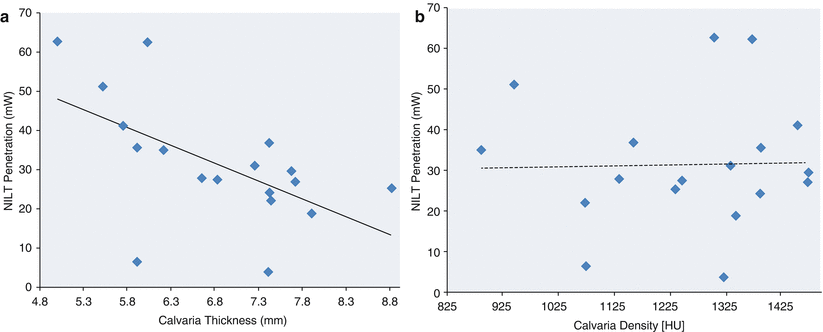
Fig. 3
Correlation analysis of NILT penetration through the dehydrated human calvaria: effect of thickness and density. (a) Plots of NILT penetration (mW) vs. thickness (mm) or (b) density (HU). Absolute penetration (mW) vs. thickness (mm) or density (HU). Correlation analysis was used to calculate R 2 values for each measurement point. In Panel a the R 2 values and Pearson correlations were 0.33433 (−0.57409, p = 0.0127) for thickness at the bregma. In Panel b, the R 2 values and Pearson correlations were 0.00071 (0.02658, p = 0.9166) for density at the bregma. There was a significant correlation between NILT penetration and skull thickness but not density
There is some literature on possible depth of penetration across the human skull and limited information on the rabbit skull. Using the RSCEM, NILT penetration of the rabbit skull and brain is achieved to a depth of 25–30 mm, almost the complete thickness, and the NILT beam would encompass the majority of the brain if placed on the skin surface posterior to the bregma at the midline [22]. In human studies, the investigators also indicate that NILT would penetrate the brain to approximately 20 mm depth using a power density of 10 mW/cm2 (1.2 J/cm2 on the cortical surface) [39]. Mathematical estimates indicate an estimated Power density drop from 10 mW/cm2 (1.2 J/cm2) to 7.5 μW/cm2 (0.9 mJ/cm2) at 18 mm depth from the cortical surface [2]. The extensive power density drop has also been addressed previously [29, 37]. Wan et al. [37] calculated that a power density of 8 J/cm2 applied to the “head” will allow for penetration of 2–3 % of laser photons up to 1 cm from the scalp surface; furthermore, only 0.2–0.3 % of photons will reach 2 cm depth. Thus, the overall penetration of continuous wave (CW)-administered near-infrared photons to significant depths is almost negligible.
NeuroThera Effectiveness and Safety Trial (NEST) Efficacy, Failures, and Considerations
NEST-1 was a phase II, double-blind clinical study randomized (2:1) in favor of NILT. The study included 120 patients 40–85 years of age with an average National Institutes of Health Stroke Scale (NIHSS) entry score of 7–22 (mean NIHSS 10), and time to treatment was 16 h 20 min [12]. The primary endpoints were NIHSS and modified Ranking Scale (mRS). As described in detail elsewhere [12], the laser probe was applied to 20 points on the skull for a 2-min duration at each spot, using a power density of 10 mW/cm2 delivering a cortical energy density of 1.2 J/cm2. The study found that NILT-treated patients showed greater significant improvement from baseline to 90 days (p = 0.021) than did the sham-treated group and NILT was safe with no differences in mortality rates (placebo 9.8 % vs. NILT 8.9 %) or serious adverse events (SAE: placebo 36.6 % vs. NILT 25.3 %) between the two groups.
NEST-2 was also a phase III, double-blind clinical study with equal randomization (1:1) that enrolled 660 stroke victims 19–95 years of age [39]. The trial was nearly identical to NEST-1, but used mRS as the primary endpoint with NIHSS for additional stratification analysis. In NEST-2 the time to treatment was 14 h 38 min. Unlike NEST-1, the trial was not positive (p = 0.094) for all enrolled patients, with an average NIHSS entry score of 13.1. Interestingly, additional post hoc analysis with severity stratification provided some evidence that stroke patients with NIHSS scores of 7–15 upon enrollment achieved better performance at 90 days (p = 0.044). Once again, there were no changes in mortality rate or SAEs, but the mortality rate in this trial was almost double that of NEST-1.
The NEST-3 clinical trial [9] was a phase III clinical trial delivering NILT within 24 h of a stroke. The study proposed to include a limited number of patients with an NIHSS baseline score of 7–17, which was based upon “efficacy” in the NEST-2 clinical trial [12, 39]. Moreover, as described in the NIH clinical trial design, only patients with cortical infarcts were enrolled, presumably because of the limited penetration of laser light across the scalp and skull. The trial was ended because of futility at interim analysis [9]. Clearly, with the current information from human skulls, NILT does not effectively cross barriers and must be optimized to promote neuroprotection in humans.
Conclusion
The principal aim of this chapter was to revisit the use of transcranial laser therapy to treat stroke and to discuss important information concerning the extent of NILT penetration through the skull of four species. Our results suggest that the use of small animal species with “thin” skulls, compared to humans, cannot be used to effectively model human diseases because of differential laser penetration and scatter patterns, in Lapchak et al [24]. Based on the scientific information presented here and the efficacy profile of NILT in multiple preclinical translational models, NILT should be further pursued as a possible important neuroprotective or neurorestorative treatment for stroke. It is evident from the literature that neuroprotection must be administered as soon as possible after a stroke [17, 31]. Extensive treatment delays have yet to be found efficacious in any animal model or clinical trial.
It is clear, however, because of significant variances of NILT penetration and skull thickness, that NILT must first be optimized in animals using novel approaches incorporating human skull characteristics. In addition, all future translational studies should incorporate standard STAIR criteria [32, 38], RIGOR guidelines [13, 18, 23], and, most importantly, studies in multiple species [15, 33, 34] for safety [7] and efficacy [15, 33, 34].
Conflict of Interest Statement
This study utilized a K-Laser model K-1200 device. K-Laser, Inc. did not have an editorial influence on the study design or scientific content of this article.
Disclosures and Acknowledgments
Dr. Lapchak serves as editor-in-chief of the Journal of Neurology & Neurophysiology and associate editor of Translational Stroke Research. The scientific content of this work was not directly supported by the National Institutes of Health or any other funding source external to Cedars-Sinai Medical Center. He was supported in part by a U01 translational research grant NS060685. Paul D. Boitano has no disclosures.
References
1.
Chung H, Dai T, Sharma SK, Huang YY, Carroll JD, Hamblin MR (2012) The nuts and bolts of low-level laser (light) therapy. Ann Biomed Eng 40:516–533PubMedCentralCrossRefPubMed
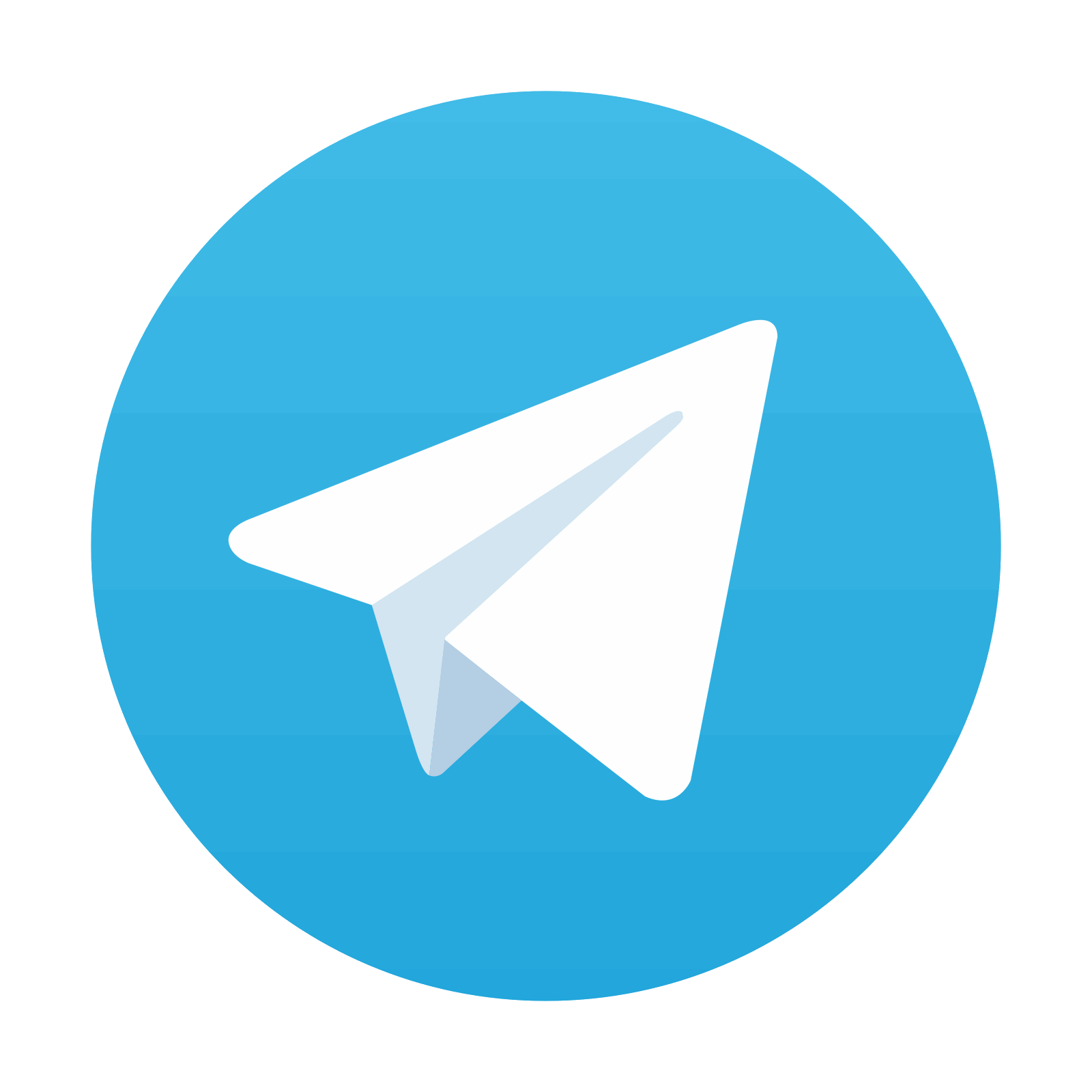
Stay updated, free articles. Join our Telegram channel

Full access? Get Clinical Tree
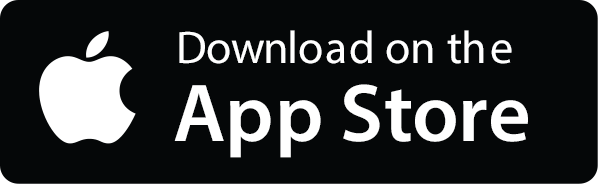
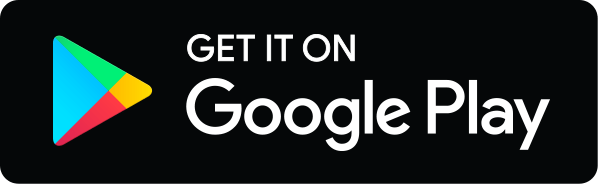