Model (animal, duration of hypoxia)
Dose; route; timing
Engraftment (days after transplantation)
Functional outcome
Cellular/molecular effects
References
P7 Wistar rats, 80 min
1 × 107 cells, IP; 24 h after HI
Large number of cells in the ischemic hemisphere (20 days)
Improved motor outcome (footprint analysis)
N/A
Meier et al. (2006)
P7 Wistar rats, 120 min
1 × 107 cells, IV; 24 h after HI
Few cells in the brain (24 h, 1 week and 3 weeks)
Spatial memory deficits persisted (Morris water maze)
Infarct size was not changed
de Paula et al. (2009)
P7 Sprague-Dawley rats, 150 min
1,5 × 104 cells, IV; 7 days after HI
Few cells in the ischemic hippocampus (14 days)
Improved motor outcome (rotarod and elevated body swing test)
Increased levels of GDNF, BDNF and NGF in the brain Increased hippocampal CA1 dendritic density
Yasuhara et al. (2010)
P7 Lister-Hooded rats, 90 min
2 × 106 cells, IP; 3 h after HI
Few cells in the ischemic cortex and striatum (2 days)
Prevented deficits in neonatal reflexes (cliff aversion and negative geotaxis)
Decreased neuronal death in the striatum Decreased microglial activation in the cortex
Pimentel-Coelho et al. (2010)
P7 Wistar rats, 80 min
1 × 107 cells, IP; 24 h after HI
Large number of cells in the ischemic hemisphere (48 days)
Decreased sensorimotor deficits (cylinder test)
Infarct size was not changed Reduced neural processing impairments in the primary somatosensory cortex
Geissler et al. (2011)
Biodistribution of the Transplanted Umbilical Cord Blood Mononuclear Cells
Meier et al. (2006) have found numerous donor UCBCs in the brain of hypoxic-ischemic rats, up to 41 days after the intraperitoneal transplantation (performed 24 h after the injury). The cells were localized only in the damaged brain regions, especially in areas with an increased expression of the chemokine stromal-derived factor-1 (SDF-1). Furthermore, they have demonstrated that SDF-1 expression is upregulated in reactive astrocytes after the injury and that the migration of UCBC to the ischemic brain could be blocked by the administration of SDF-1 neutralizing antibodies. Interestingly, there were no evidences of neuronal or glial differentiation of the transplanted cells (Meier et al. 2006; Rosenkranz et al. 2010; Geissler et al. 2011). In contrast, two other research groups have failed to find a large number of donor cells in the hypoxic-ischemic brain, when UCBC were intravenously transplanted 7 days after the injury or intraperitoneally injected 3 h after the injury, despite the functional benefits of the treatment (Yasuhara et al. 2010; Pimentel-Coelho et al. 2010). These apparently divergent observations could be attributed to the different timing of transplantation or to the different cell dose used in each study. Nevertheless, they indicate that the therapeutic effects of UCBC transplantation do not depend on the migration of the transplanted cells to the brain or on the differentiation of the donor cells into neural cells. Therefore, these studies suggest that neuronal replacement is not the mechanism of action of transplanted UCB mononuclear cells in HIE.
This is an important observation, given that some studies have reported that UCBC could differentiate into neurons under specific culture conditions. One of these studies has demonstrated that the UCB has a population of unrestricted somatic stem cells that grow as adherent cell colonies in the presence of dexamethasone. These cells can be induced to differentiate into neuron-like cells, although only a minority (11%) of the differentiated cells possesses functional voltage-gated sodium channels (Greschat et al. 2008). However, when UCB-derived unrestricted somatic stem cells were transplanted in an animal model of acute spinal cord trauma, there was no evidence of neural differentiation of the graft cells. Nevertheless, the cell therapy reduced the lesion size and promoted axonal regrowth in the injured spinal cord, which in turn resulted in an improved locomotor recovery of the treated animals. In this study, the authors have also identified hepatocyte growth factor as an important chemoattractant for UCB unrestricted somatic stem cells and they have demonstrated that the secreted factors present in the conditioned medium of these cells can stimulate neurite outgrowth in vitro (Schira et al. 2012). Taken together, these studies indicate that UCBC transplantation is not a cell replacement therapy. Multiple mechanisms seem to be involved in the therapeutic effects of UCBC, which are probably mediated by a paracrine effect.
Cellular and Molecular Mechanisms of Action of Umbilical Cord Blood Mononuclear Cells
The neuroprotective effects observed after UCB mononuclear cell transplantation in animal models of cerebral ischemia, such as in HIE and stroke (Pimentel-Coelho et al. 2010; Vendrame et al. 2006), were also replicated in several in vitro models of neuronal death. These studies are contributing to clarify the molecular and signaling pathways involved in UCBC-mediated neuroprotection and to identify which cell populations are involved in this effect.
For instance, it was observed that UCBC increase the survival of neuronal cells in an in vitro model of glutamate-induced toxicity, through the activation of the Akt pro-survival pathway (Dasari et al. 2008). Similarly, UCBC protect oligodendrocytes against oxygen/glucose deprivation in vitro, as well as in an animal model of stroke, through activation of Akt and upregulation of the antioxidant enzyme peroxiredoxin 4 (Rowe et al. 2012). However, it still needs to be investigated whether UCB would protect oligodendrocyte progenitors, which are highly susceptible to HIE.
Regarding the UCB cell types contributing to neuroprotection, it has been demonstrated that the CD133+ population, which encompass both HSPC and endothelial progenitor cells, decreased apoptosis and prevented the deleterious effects of hypoxia on axonal growth in organ co-cultures of the cerebral cortex and spinal cord from 3-day-old neonatal rats (Tanaka et al. 2010). However, one study has observed that UCB CD133+ cells and CD133− cells (the UCB mononuclear cell fraction depleted of CD133+ cells) provided a similar degree of neuroprotection in an in vitro model of neuronal hypoxia (Reich et al. 2008). Furthermore, UCB mononuclear cell transplantation provides an increased protection after stroke, than the transplantation of UCB CD34+ cells or than the mononuclear fraction depleted of CD34+ cells (CD34− cells), indicating that multiple cell types are involved in this effect. Both CD34+ and CD34− cells decreased the infarct volume and improved the motor function of the treated animals to a similar extent, although less efficiently than the complete mononuclear cell fraction. However, while the mononuclear cells and the CD34-enriched fraction protected hippocampal neurons in a model of oxygen and glucose deprivation, the CD34-depleted fraction had no effects on neuronal viability (Boltze et al. 2012). Taken together, these observations suggest that multiple cell types may contribute to the therapeutic effect of the UCB mononuclear cell fraction and indicate a role of CD34+ cells, which includes a population of HSPC and endothelial progenitor cells, in neuroprotection.
Interestingly, neuroprotection can be achieved by either direct or indirect co-cultivation of UCBC with post-hypoxic neuronal cells (Reich et al. 2008), suggesting that secreted soluble factors are largely responsible for the neuroprotective effects of UCBC. Accordingly, freshly isolated UCBC express higher levels of the mRNA of several neurotrophic factors, including BDNF, GDNF, NGF, neurotrophin-3 (NT-3) and NT-5, when compared to peripheral blood mononuclear cells (Fan et al. 2005). In addition, BDNF, vascular endothelial growth factor (VEGF), NT-4, NT-5 and several cytokines and chemokines can be found in the conditioned medium of UCBC cultures (Fan et al. 2005; Newman et al. 2006). These evidences, coupled to the absence of neural differentiation of the transplanted cells, suggest that UCBC might exert their therapeutic actions through a paracrine effect in the hypoxic-ischemic brain.
Besides neuroprotection, other mechanisms may underlie the functional benefits observed after UCBC transplantation in animal models of cerebral ischemia. For instance, Taguchi et al. (2004) have shown that UCBC stimulate angiogenesis and neurogenesis after stroke. Moreover, the effect of UCBC treatment on neurogenesis was abolished by the administration of endostatin, an antiangiogenic agent. Given that neurogenesis and angiogenesis are coupled and are regulated by the same growth factors (i.e., VEGF and angiopoietins), it is possible to speculate that some of the effects of UCBC might be related to the secretion of angiogenic factors.
Another line of evidence suggests that UCBC may have an anti-inflammatory effect after cerebral ischemia. UCBC treatment decreased the number of activated microglial cells in the cortex in a model of HIE (Pimentel-Coelho et al. 2010). In addition, intravenous UCBC transplantation reduced the infiltration of B lymphocytes and decreased the expression of pro-inflammatory cytokines in the brain of rats subjected to middle cerebral artery occlusion (MCAO; Vendrame et al. 2005). Although this anti-inflammatory effect is not yet completely understood, it has been suggested that UCBC can modulate the response of the spleen after stroke in rats, suggesting a systemic immunomodulatory role of UCBC treatment. In this regard, UCBC transplantation prevented the stroke-induced alterations in spleen size, cellular composition and function. This effect was accompanied by an increased expression of the mRNA of interferon-gamma and of the anti-inflammatory cytokine IL-10 in the spleen (Vendrame et al. 2006).
Therefore, UCBC exert their beneficial effects in the ischemic brain through a combination of multiple mechanisms. Future efforts should be made to understand which trophic/growth factors are involved in these mechanisms. Genetic manipulation of the cells before transplantation could increase the expression and the secretion of potentially therapeutic neurotrophic/growth factors by UCBC. In this regard, a recent study has reported a non-viral lipofection technique to obtain UCB CD34+ cells stably expressing the GDNF gene (Yu et al. 2010). It is also necessary to identify which cell populations contribute to the therapeutic effects and whether the depletion or the enrichment of one (or more) cell population(s) could further improve the benefits on neurological function.
Clinical Translation
Currently, two clinical trials are assessing the safety and the feasibility of autologous UCBC transplantation in HIE. In the first study, conducted at Duke University, newborns with HIE will be treated with up to four infusions of 5 × 107 cells/kg (depending on the number of available cells), within the first 14 postnatal days. Participants will be assessed by neurodevelopmental and neuroimaging examination for 1 year (http://clinicaltrials.gov; Identifier: NCT00593242). The number of cells transplanted in this clinical trial (even considering that the percentage of mononuclear cells will depend on the procedure used for reducing the volume of cord blood) is not so different from the dose used in the preclinical studies discussed above. In these studies, the cell dose ranged from 1.5 × 104 to 1 × 107 mononuclear UCBC (approximately 1.1 × 106–7.5 × 108 cells/kg of body weight). Nevertheless, future dose-response studies should address what is the optimal number of cells required for a long-term therapeutic effect in HIE.
The second trial has started recruiting participants in January 2012, in Mexico. In this study, autologous non-cryopreserved UCB CD34+ cells will be intravenously transplanted in term newborns with HIE, within the first 48 h after birth. Participants will be followed by clinical examination for 1 year (http://clinicaltrials.gov; Identifier: NCT01506258). In both studies, the results will be compared to historic controls.
In addition, there are two ongoing clinical trials evaluating the safety and effectiveness of autologous UCBC transplantation in children with cerebral palsy (CP), a group of non-progressive syndromes of motor and posture impairments caused by a wide range of acquired brain disorders during the antenatal or perinatal period. HIE is the cause of cerebral palsy in at least 14% of the cases, especially (but not exclusively) causing dyskinetic or spastic tetraplegic cerebral palsy (Graham et al. 2008). The trial at Georgia Health Sciences University, is recruiting children with a non-progressive motor disability, from 1 to 12 years, who were unable to sit independently by 12 months of age or unable to walk independently by 18 months of age, while the study at Duke University is treating children with spastic CP with diplegia, hemiplegia, or quadriplegia, ranging from 1 to 6 years of age. Both studies are randomized, crossover trials, in which both treatment groups (i.e., placebo first, or UCBC treatment first) will receive UCB mononuclear cells, but at different points in the study (http://clinicaltrials.gov; Identifiers NCT01072370 and NCT01147653, respectively).
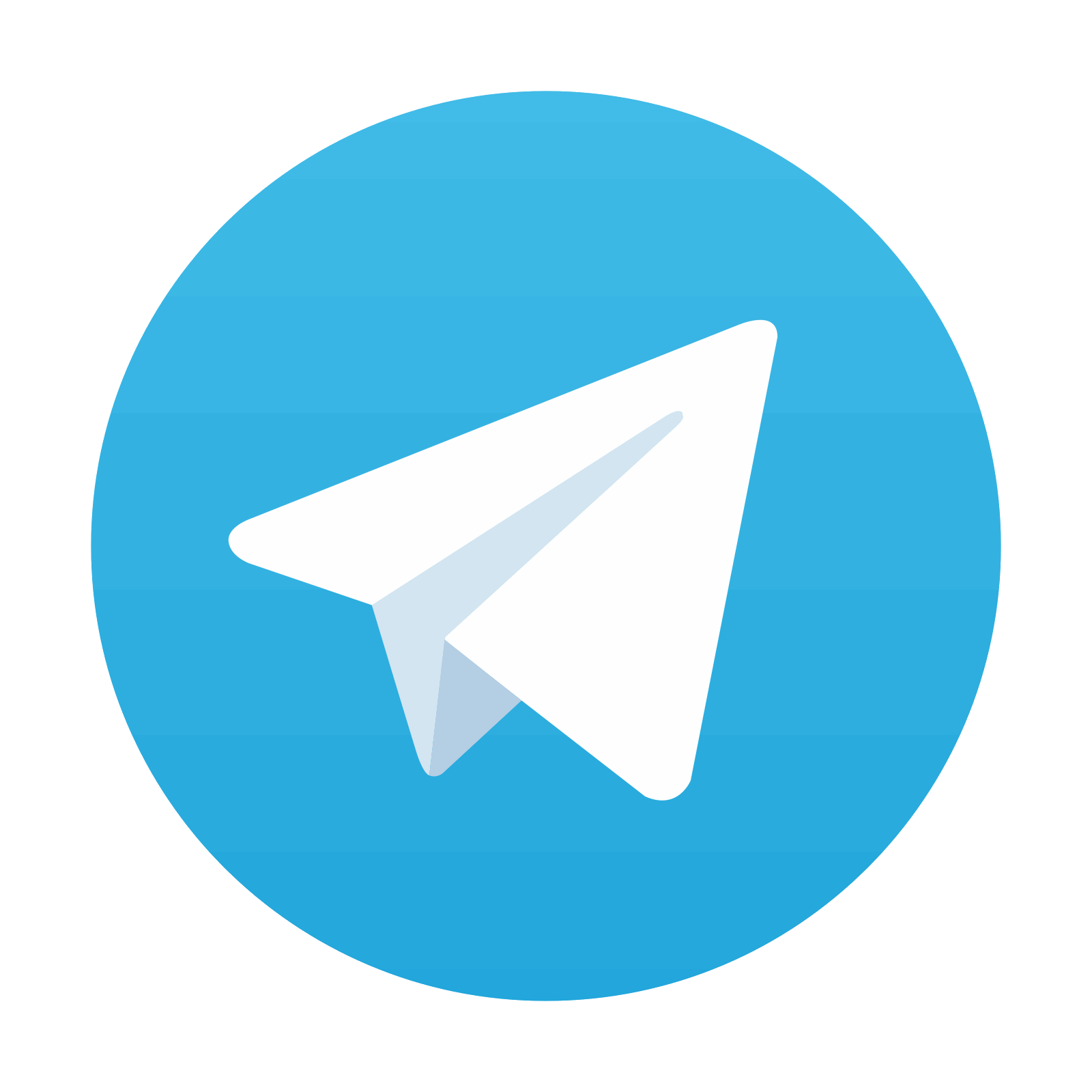
Stay updated, free articles. Join our Telegram channel

Full access? Get Clinical Tree
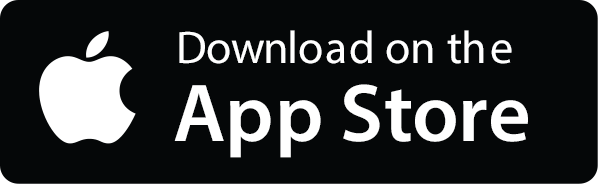
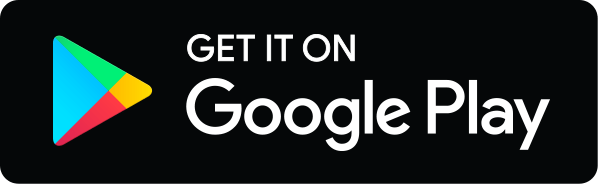