Figure 6.1 Activated areas on axial planes of the standard glass brain images (upper side of axial image shows left hemisphere). The figure on the left shows the activated areas averaged across eight HSS, while those on the right show those of LSS. Panels show the activated areas under the LST condition. See also color plate 1.
(p.103)

Figure 6.2 The mean time course of signal changes in the ACC and PFC during the LST condition. The left figure shows signal changes of HSS and the right those of LSS. Adapted with permission from Oasaka, M., Osaka, N., Kondo, H. et al. (2003). The neural basis of individual differences in working memory capacity: an fMRI study. NeuroImage, 18, 789–797, Elsevier Science.

Figure 6.3 ACC and DLPFC network differences between HSS and LSS groups. The solid line shows the network for HSS and the dotted line that for LSS. The dotted line shows weak network of LSS between DLPFC and ACC.
(p.104) Based on these findings, Osaka et al. (2003, 2004a) concluded that the ACC subserves attention control in working memory, allowing inhibition and monitoring in accordance with the DLPFC. While performing the span task such as LST and RST, the attention controller of the executive function was regulated by the DLPFC and ACC, which play roles in maintaining attention and inhibitory processes, respectively. As for capacity differences, it was found that activation differences between groups were also derived from differences in these two regions and high functional connectivity between ACC and DLPFC is constantly observed in HSS but not in LSS.
By applying structural equation modeling (SEM), we also identified the network system between DLPFC and ACC with empirical data using the OST (Kondo, Morishita, Osaka et al. 2004a). During OST, the subjects performed verification of arithmetic problems and retention of target words. Behavioral data showed that the performance was better in HSS than in LSS under OST, but there was no difference under two single-task conditions, that is, an arithmetic task and a word retention task.
Previous studies reported that the OST scores like RST scores are highly correlated with reading comprehension (Turner and Engle 1989). Engle et al. (1999) also suggested that predicted power of correlations between working memory tasks and cognitive tasks is derived from executive functioning using SEM. Then, we tested how activation of multiple brain areas is connected with working memory using SEM. We employed SEM to construct the best fitting neuronal network model that can account for time-series fMRI of ROIs.
The results showed that the ACC, left PFC and bilateral parietal cortex were activated for both groups. However, signal changes in the ACC were greater in the HSS than in the LSS under OST. SEM indicated that an estimate of effective connectivity from the ACC to the left PFC was positive (0.43) for the HSS while negative (−0.14) for the LSS, indicating that closer cooperation between the two brain areas was strongly related to working memory performance in HSS.
Using a spatial span task (SST), we also compared networks between ACC and DLPFC (Kondo, Osaka and Osaka. 2004b). In the SST, letter verification with mental rotation and retention of arrow orientations was performed. A significant positive correlation with signal change in the right DLPFC and right ACC (r = 0.49) was found under dual task. The rotation-arrow task is known to correlate with visuospatial processing (Shah and Miyake 1996). Right DLPFC activation was followed as the right brain plays a crucial role in visuo-spatial processing (Courtney et al. 1998; Jonides et al. 1993). We again confirmed that mean signal change was greater for the HSS than for the LSS under dual task, but not under either the rotation or arrow condition alone (single task). It was also found the effective connectivity between the right DLPFC and right ACC in the HSS but not in the LSS using SEM analysis.
These findings confirmed that executive function, i.e., attention controlling system, is more active in HSS than in LSS. These findings also suggest that there is a general neural basis for the central executive function in span tasks despite differences in modality-specific buffers that are employed by the phonological loop during RST, LST and OST or by visuospatial sketchpad during SST.
Focus and inhibitory processes
Little is known, however, regarding the neural bases of focusing attention during the span task. Focusing attention is extremely important for attentional control systems in executive function (Cowan 2001). The role of the central executive is related to the control and selection of the currently relevant portion of activated long-term memory representations, which was thought to be under the surveillance of the focus of attention (Cowan 1999, 2001). Cowan (2001) suggested (p.105) that the focus of attention represents a capacity-limited part of working memory and that the focus of attention holds a restricted sets of items, that is, about four independent units.
Focusing attention is also important for language comprehension during reading or listening (Blutner and Sommer 1988; Carpenter and Just 1977; Hornby 1974; Osaka, Nishizaki, Komori et al. 2002). In text reading, Birch and Garnsey (1995) investigated the effect of focus on memory for words in sentences and found that focusing on a word enhances memory. They proposed that focus in the sentence facilitates the process of integrating information in sentences and is critical for creating a coherent understanding. Carpenter and Just (1977) also suggested that subjects direct their attention initially to the focus of a sentence and then focused constituents are easily comprehended during reading.
Thus, while reading a sentence, readers usually focus attention on the most critical word to facilitate comprehension of the sentence. Once readers make the mental representation with the focus word, the contents of the sentence may have been easily accessed via the mental representation. Thus, it became easier for the subjects to read and comprehend the sentences by using these mental representations.
Other researchers suggest the importance of an inhibitory mechanism in reading. Gernsbacher (1990) suggested that a deficit in the inhibitory mechanism is implicated in comprehension difficulty In an experiment by Gernsbacher and Faust (1991), subjects were told to focus on either a picture or a word in the context display and to ignore the other. Then they were asked to indicate whether the probe word was related to the word in the context display. They found differences between skilled and less-skilled readers in terms of ignoring an irrelevant stimulus. The results suggested that the suppression mechanism of less-skilled readers is less efficient in suppressing the non-focused, ignored information than that of more skilled readers.
Similar suggestions have been made regarding deficits in inhibiting among those who perform poorly on the span task (Conway and Engle 1994; De Beni, Palladion, Pazzaglia et al. 1998; Engle, Conway, Tuholski et al. 1995; May, Hasher and Kane 1999). These studies showed that low performers in the span task have a deficit in inhibiting information irrelevant for the task. De Beni et al. (1998) presented random strings of words instead of sentences on the RST while requesting subjects to tap the hand when an animal noun appeared in the word strings. Then the subjects were required to recall the last word each set. The number of intrusion errors that subjects made when they recalled the target word were counted and compared between the two subject groups, identified as good comprehenders and poor comprehenders. The results showed that the intrusion errors when the subjects recalled the target words were larger for poor comprehenders than for good comprehenders. In addition, within the intrusion errors made by poor comprehenders, intrusions more frequently involved animal nouns than other non-target words. These results suggested that the low comprehenders had difficulty in inhibiting words that they had previously focused attention on.
Focused RST vs non focused RST
In order to confirm the importance of focusing attention in span task, Osaka et al. (2002) developed two kinds of RST, that is focused-RST (F-RST) and non-focused RST (NF-RST) and compared the performances between F-RST and NF-RST. In the F-RST, the target word to be maintained was a focus word of the sentence. In the NF-RST, however, the target word was not a focus word of the sentence.
The focus word was defined as the most critical word for comprehension of a sentence (Birch and Garnsey 1995; Halliday 1967), that is, the word with a core meaning necessary to integrate (p.106)
Table 6.1 Sample of ‘focus word’ of a sentence and the target words of the focused and non-focused RST
The child dropped food on his jacket and made stains. Focus word |
Focused RST |
The child dropped food on his jacket and made stains. Target word |
Non-focused RST |
The child dropped food on his jacket and made stains. Target word |
Table 6.1 shows sample sentences from both focused RST and non-focused RST. In the sentence ‘The child dropped food on his jacket and made stains’, the word ‘stains’ was chosen as the focus word by the preliminary investigation. Therefore, in the F-RST, ‘stains’ was selected as the target word, while in the NF-RST version, the non focus word ‘food ‘was selected as a target word. The non- focus word, that is ‘food ‘in NF-RST, was selected on 17 per cent of occasions as the most important word in the sentence.
As for the target position in the sentence of the Japanese version of RST, the target word occupied various positions in the sentence and was the underlined word in each sentence (Osaka and Osaka 1992, 1994). In studies of RST in English, the target word was the last word of each sentence (Daneman and Carpenter 1980). This difference of target position in our Japanese version was required because the last word of the sentence in Japanese is usually a verb. In addition, as a feature of Japanese syntactic mechanisms, the last word of a Japanese sentence is rarely a focus word (Kuno 1978), whereas in English, the last word of the sentence easily becomes the end focus word (Bolinger 1986).
With the difference of target position between Japanese-RST and English-RST, however, we found a significantly higher correlation between the Japanese-RST span scores and the English-RST span scores (r = 0.84, p ‹0.001) (Osaka and Osaka 1992). Moreover, as in the English RST version, a high correlation was found between the Japanese RST span scores and reading comprehension test scores (Osaka and Osaka 1994).
Focusing attention and inhibitory processes in NF-RST
While reading a sentence in RST, subjects likely focus attention on the focus word of the sentence to comprehend it. However, in RST, two different functions are concurrently executed: reading the sentences and memorizing the target words. Because the goal of the RST is to recall target words, subjects are not likely to maintain attention on the focus word of the sentence.
Thus, when the ‘target word’ to be maintained coincides with the focus of attention (‘focus word’) in the sentence, word maintenance is likely to be easier. However, when the target word does not coincide with the focus word of the sentence, subjects have to shift attention from the focus word to another target word.
Figure 6.4 shows a diagram of attention-control during F-RST (left-hand side) and NF- RST (right-hand side). In F-RST, the focus of attention corresponds between the focus word ‘stains’ and target word ‘stains’. However, in NF-RST (right-hand figure), the focus of attention does not correspond between the focus word ‘stains’ and target word ‘food’. Then subjects have to shift (p.107)

Figure 6.4 The diagram of attention control during both RST. The left diagram shows attention control under F-RST and the right that under NF-RST.
In Osaka et al. (2002), span scores were significantly higher for F-RST compared to NF-RST. The number of intrusion errors was significantly higher in NF-RST than F-RST. In the NF-RST, intrusion errors were divided into two types. One was a focus-intrusion error in which subjects recalled the focus word ‘stains’ instead of the target word ‘food’, and the other was a non-focus-intrusion in which subjects recalled a word (‘child’ for example) other than the focus word. It was interesting that most of the intrusion errors in NF-RST were focus intrusion errors. The predominance of focus-intrusion errors in NF-RST suggests that subjects had difficulty inhibiting the focus word on which the subjects had previously focused attention.
Individual differences in focusing and shifting attention
Osaka et al. (2002) also compared performance on both F-and NF-RST between HSS and LSS. The results demonstrated a significant effect of focusing and shifting attention for LSS but not for HSS. Rates of intrusion errors were higher for LSS than for HSS. These findings indicated that LSS have deficits in their ability to shift attention and inhibit the irrelevant word. These results support previous studies that indicate LSS have a deficit in ability to inhibit information irrelevant for the task (May, Hasher and Kane 1999). It was also supported by other studies that indicate poor comprehenders make significantly more intrusion errors than good comprehenders (Gernsbacher 1990; Gernsbacher and Faust 1991).
However, the LSS in Osaka et al. (2002) made significantly more intrusion errors in the NF-RST condition than in F-RST. The theory by Gernsbacher (1990) that implicates a lack of inhibition of irrelevant stimuli for poor comprehenders would predict that intrusion errors on F-RST would be equal to that on NF-RST, because there were still irrelevant stimuli even in F-RST (child, food, jacket for example). It was interesting that among intrusion errors in NF-RST in LSS, the number of focus-intrusions was significantly larger than non-focus intrusion. In HSS, on the contrary, this difference was not found. These results suggest that LSS have more difficulty in inhibiting information, especially of the focus word. If LSS had difficulty in inhibiting any kind stimuli, they would have shown a worse performance on F-RST similar to that on NF-RST. Thus, it was not that LSS can not inhibit irrelevant information, but that they can not inhibit irrelevant information after having initially focused attention on it.
Following these findings, it could be possible that shifting attention does not function well for LSS after they have previously focused attention and built a mental focus.
Neural bases of focusing and shifting attention
In the following section, neural substrates for focusing and shifting attention in executive function will be discussed. In Osaka et al. (2004b, 2007), to examine the neural bases of focusing (p.108) attention during RST, fMRI activations were compared between two kinds of RST conditions: F-RST and NF-RST. In addition, possible neural bases underlying group differences between HSS and LSS were examined.
fMRI activations were measured while subjects performed three kinds of experimental tasks: F-RST, NF-RST and READ. In READ condition, single reading of five sentences was required. Under RST conditions, subjects were required to read the sentence while concurrently remembering the target word in each sentence.
Figure 6.5 shows the experimental time course of RST and READ conditions. The lower left figure shows one block of the RST and the right shows the READ condition. Both conditions in reading phase consisted of five sentences.
Under both RST conditions, after the end of the reading phase, five probe stimuli appeared every 5 s in the recognition phase. The probe stimuli appeared in the same order as the stimulus sentences. Each probe stimulus consisted of two words and an X character. One of the probe words was the target word and other word was another word from the sentence. In F-RST, the target word was the focus word ‘stains’ of the sentence and in NF-RST, the target word was not the focus word of the sentence but rather a filler word ‘food’ was the target word. When the subject distinguished the target word between these two words, the subject pushed the key corresponding to the selected probe stimulus.

Figure 6.5 Time course of RST and READ conditions. The upper figure shows the block-design paradigm in fMRI study. The lower left figure shows one block of the RST and the right shows the READ condition. The reading phase under RST condition consisted on five sentences, which were presented at 5 s intervals. At the end of the five sentences under the RST condition, recognition stimuli appeared every 5 s during the recognition phase. Adapted with permission from Osaka, M., Komori, M., Morishita, M., & Osaka, N. (2007). Neural basis of focusing attention in working memory. Cognitive, Affective & Behavioral Neuroscience, In Press, The Psychonomic Society.
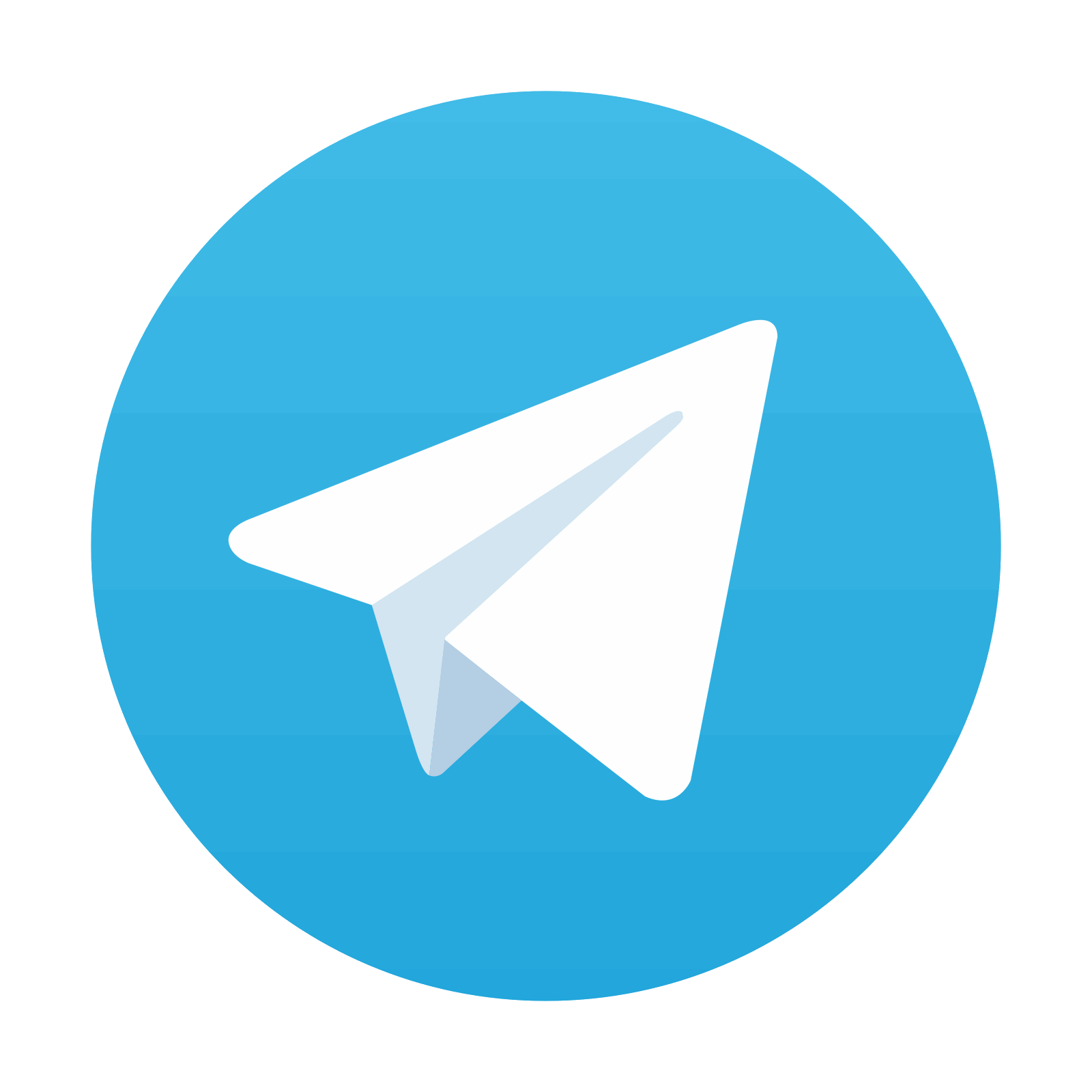
Stay updated, free articles. Join our Telegram channel

Full access? Get Clinical Tree
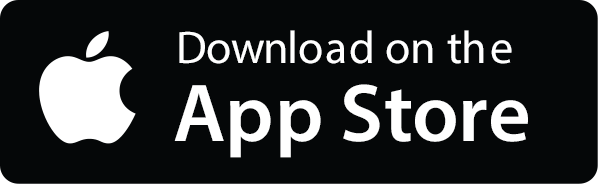
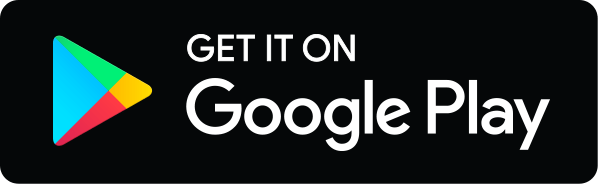
