(1)
Department of Behavioral Neurobiology, Institute of Experimental Medicine, Budapest, Hungary
Abstract
Any detail on the control of aggression is extremely valuable, but the grand aim is the understanding of the circuitry that subserves aggression and the identification of those alterations in the system that makes it dysfunctional. This major objective does not seem to be achieved at present stage. The main problem with the theories is their large number. While they operate with similar concepts overall, and cross-fertilization is also evident, approaches remain rather divergent. Our attempt of understanding aggression control will start with a brief presentation of the current concepts. Neither will be scrutinized at this stage. The following chapter will be devoted to an in-depth analysis of the nodal points of aggression control. The last chapter is a trial to put pieces together.
Any detail on the control of aggression is extremely valuable, but the grand aim is the understanding of the circuitry that subserves aggression and the identification of those alterations in the system that makes it dysfunctional. This major objective does not seem to be achieved at present stage. The main problem with the theories is their large number. While they operate with similar concepts overall, and cross-fertilization is also evident, approaches remain rather divergent. Our attempt of understanding aggression control will start with a brief presentation of the current concepts. Neither will be scrutinized at this stage. The following chapter will be devoted to an in-depth analysis of the nodal points of aggression control. The last chapter is a trial to put pieces together.
3.1 Animals
3.1.1 Cats
The most extensive mapping of the circuits that control aggressiveness was performed by the group of Siegel in cats; the studies available so far cover a period of 38 years (Siegel and Flynn 1968 through Bhatt and Siegel 2006). By using a complex set of advanced technologies, the group not only uncovered the brain areas that are involved in cat aggression and their connections but also the neurotransmitters used by the neurons of the circuit. A simplified summary of this exceptionally systematic work was summarized in Fig. 3.1.
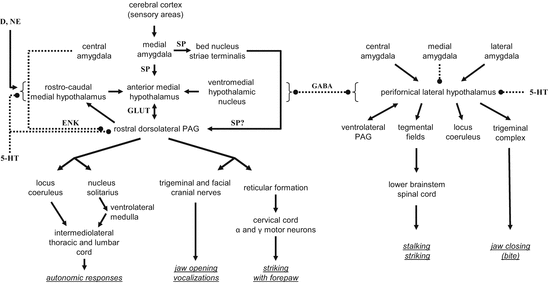
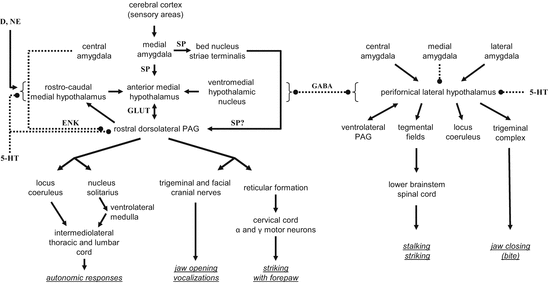
Fig. 3.1
The circuitry that controls affective aggression (left) and predatory aggression (right) in the cat (based on Siegel et al. 1999; Gregg and Siegel 2001; Siegel et al. 2007). Abbreviations: 5-HT serotonin; D dopamine; GABA gamma aminobutyric acid; GLUT glutamate; ENK enkephaline; NE norepinephrine; SP substance P; arrows, continuous line, stimulatory contacts; two-sided arrows, bidirectional stimulatory contacts; dotted lines with bullets, inhibitory contacts; dotted lines with bullets on both sides, bidirectional inhibitory contacts
The centerpiece of the circuitries identified by this group is the hypothalamus, the role of which was revealed by the seminal work of Hess (1928). In fact, the Siegel group asked two major questions: (1) which are the mechanisms that control the release of attacks from the hypothalamus, and (2) how does the hypothalamus control the sequence of movements that make up an attack? They identified two topographically distinct aggression-controlling mechanisms. One of these controls affective aggression and involves a downward stimulatory stream that includes the medial amygdala, medial hypothalamus, and dorsal periaqueductal gray, while the other controls predatory aggression and includes the central and lateral amygdala, lateral hypothalamus, and a series of downstream areas including the ventral periaqueductal gray. From upstream, both systems were assumed to be controlled by information received from the sensory cortex, while downstream, both were assumed to control neural mechanisms involved in autonomic and motor control. The two systems reciprocally inhibit each other at multiple levels, which implies that affective aggression inhibits predation, and similarly, affective aggression has low chances of expression during hunting. Stimulations within the circuitry were assumed to be realized by glutamatergic and substance P-ergic synapses, while inhibitions were assumed to be mediated by GABAergic and enkephalinergic neurotransmission (Fig. 3.1).
A blatant feature of the circuitry outlined by this group is the omission of the prefrontal cortex, which is considered a key region by practically all the other authors. The omission was not due to lack of interest or the incomplete survey of relevant subregions. Brutus et al. (1984) studied the involvement of this brain area by a combined technique. They electrically stimulated various prefrontal and other regions and identified the activated brain regions by [14C]2-deoxyglucose analysis.1 In the first series of studies, stimulation was performed in the dorsomedial, ventromedial, and infralimbic prefrontal cortices. Based on the figures provided, these areas seem analogous with the medial orbitofrontal, prelimbic and infralimbic cortices of rats. This detail appears important especially in comparison with rat findings. The stimulation activated a series of downstream areas, the largest activation being seen in the mediodorsal thalamus. No activation was observed in the hypothalamus. In the next step, the authors stimulated the mediodorsal thalamus, which activated the hypothalamus neither, but among other nuclei it activated the nucleus reuniens. When this was stimulated, finally the hypothalamus was activated; activation covered both the medial and lateral hypothalamus and a descending system, which could be followed to the level of the periaqueductal gray. This study suggested that information from the prefrontal cortex does reach the hypothalamus, but by a multi-synaptic pathway that goes through the mediodorsal thalamus and nucleus reuniens. The lack of the prefrontal cortex from all the schematics provided by this group is likely explained by the complexity of the synaptic links.
Another interesting feature of the mechanisms depicted in Fig. 3.1 is that affective aggression results in the opening of the jaws, while their closing results from the activation of the predatory mechanism. The likely reasons of this strange assumption are discussed below.
3.1.2 Rats
Hypothalamic mechanisms of aggression, including its neurochemical control, received considerable attention in rat studies as well (for a review see Kruk 1991), but the circuits controlling the hypothalamus and those translating hypothalamic activation into movement patterns were less systematically investigated. However, the circuits controlling rivalry aggression may be deduced from the findings of two studies that investigated the efferent and afferent connections of the mediobasal hypothalamus. Efferent connections were studied by Roeling et al. (1994), while afferent connections by Toth et al. (2010). Both studies found a surprisingly complex set of monosynaptic connections; the efferents were sent to and the afferents were received from over 50 brain areas, many of which overlapped. The large degree of overlapping demonstrates that most of the connections are reciprocal. Examples of these are shown in Fig. 3.2 (left-hand panel). There were a few notable exceptions from this reciprocity; it occurs that the connections are unilateral in the case of the prefrontal cortex-mediobasal hypothalamus and the mediobasal hypothalamus-periaqueductal gray connection; the medial amygdala-mediobasal hypothalamus connectivity appears to be highly asymmetrical. In all three cases, a downward stream of information can be assumed. This downward information flow may be complemented by a prefrontal cortex-medial amygdala connection, which was not studied by the aforementioned authors, but is evident from other sources (e.g., McDonald et al. (1999) described a direct connection between particular infralimbic neurons and the medial amygdala).
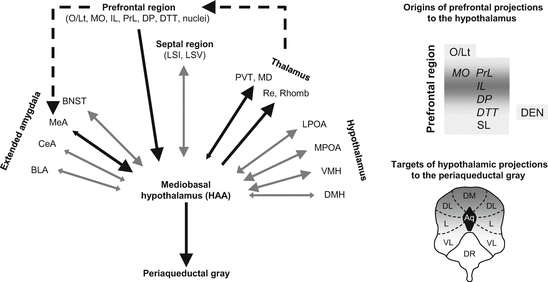
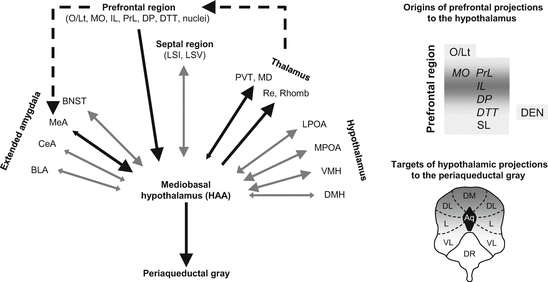
Fig. 3.2
Afferent and efferent connections of the mediobasal hypothalamus in the rat (based on Roeling et al. 1994 and Toth et al. 2010). Legend: Direction and size of arrowheads, represents the direction and stregth of the connection; Black arrows, connections that suggest directionality; gray arrows, reciprocal connections; dashed arrows, connections evidenced by other studies; grayness in right-hand panels: the strength of connections. Explanations for abbreviations were grouped according to major brain areas. Prefrontal regions: DEN dorsal endopiriform cortex; DP dorsal peduncular cortex; DTT dorsal tenia tecta; MO medial orbitofrontal cortex; O/Lt olfactory-limbic transition area; PrL prelimbic cortex; SL semilunar nucleus; Septal region: LSI lateral septal nucleus, intermediate part; LSV lateral septal nucleus, ventral part; Extended amygdala: BLA basolateral amygdala; BNST bed nucleus striae terminalis; CeA central amygdala; ; MeA medial amygdala; Thalamus: MD mediodorsal thalamic nucleus; PVT paraventricular nucleus of the thalamus; Re nucleus reuniens; Rho rhomboid nucleus; Hypothalamus: DMH dorsomedial hypothalamic nucleus; HAA hypothalamic attack area; LPOA lateral preoptic area; MPOA medial preoptic area; VMH ventromedial hypothalamic nucleus; Periaqueductal gray: DM dorsomedial nucleus; DL dorsolateral nucleus; L lateral nucleus; VL ventrolateral nucleus; DR dorsal raphe
Taken together, these findings suggest that the affective aggression mechanism evidenced in cats does work in rats and is composed of the same neural structures: the prefrontal cortex, medial amygdala, mediobasal hypothalamus, and periaqueductal gray. Other similarities between the two species are also suggestive of shared mechanisms. For example, the medial hypothalamus appears to be controlled by the same prefrontal subareas (the orbitofrontal, prelimbic, and infralimbic cortices) in cats and rats, even if the control seems indirect in the former and direct in the latter. Moreover, affective aggression is controlled by dorsal aspects of the periaqueductal gray in cats, while the hypothalamic attack area of rats preferentially projects to this region in rats (see the right-hand panels of Fig. 3.2).
In addition to this downward stream, asymmetric connections suggest the existence of an upward flow of information. The components of this putative stream are the mediobasal hypothalamus, various nuclei of the thalamus, and the prefrontal cortex (for evidence on thalamus-prefrontal cortex connections, see Groenewegen 1988). The assumption that the mediobasal hypothalamus sends information to the prefrontal cortex via the thalamus is supported by a study where the electrical stimulation of the hypothalamic attack area was followed by the activation of the prefrontal cortex despite the lack of a direct anatomical link; this was explained by the mediatory role of the mediobasal thalamus, which was also activated by stimulation (Halasz et al. 2002b).
Based on the above and sporadic data on predatory aggression in rats, we hypothesized that the mechanisms controlling rodent aggression are very similar to those operative in cats: rivalry aggression is governed by a circuit composed of the medial amygdala, mediobasal hypothalamus, and dorsal periaqueductal gray, while predatory aggression is controlled by a parallel mechanism composed of the central amygdala, lateral hypothalamus, and ventral periaqueductal gray (Haller 2013).
3.2 Humans
Information on the neural control of aggression in animals (especially in cats) was used to build simple mechanistic models of human aggression, the various forms of which were perceived as variants of affective and predatory aggressions that are seen in cats (Siegel et al. 2007, Siegel and Douard 2011). According to these views, the mechanisms operating in animals and humans are very much the same with the exception that there is some room for free will in the latter. However, psychological approaches that can be traced back to the nineteenth and early twentieth century operated with quite different notions, and their impact is strongly felt even today. Current theories in fact amalgamate the two approaches; human aggression is usually understood by an interaction between psychological and neural factors. The general assumption is that the substrate of the former is the prefrontal cortex, while the substrates of the latter are brain areas similar to those that control aggression in animals.
The role of the prefrontal cortex in human aggression is usually explained in terms of its role in emotional and cognitive functions. Theories suggest that deficits in prefrontal functions engender aggressiveness by the loss of control over emotions and impulsivity, the inability to recognize emotions in others and lack of empathy, the inability to evaluate the consequences of actions (including insensitivity to punishment), and deficits in emotional learning which result in faulty decision making (Anderson and Kiehl 2012; Blair 2001, 2007, 2010a, b; Brower and Price 2001; Davidson et al. 2000; Gao et al. 2009; Golden et al. 1996; Herpertz and Sass 2000; Hoaken et al. 1998; Lopez et al. 2004; Raine 2002; Yang and Raine 2009). The role of hypoarousal and sensation seeking was also emphasized by some authors (Gao et al. 2009; Herpertz and Sass 2000; Lopez et al. 2004; Raine 2002). These psychiatric mediators of prefrontal deficits appear in different combinations and are emphasized in different ways by the authors referred to above. Yet their conclusions are consonant overall.
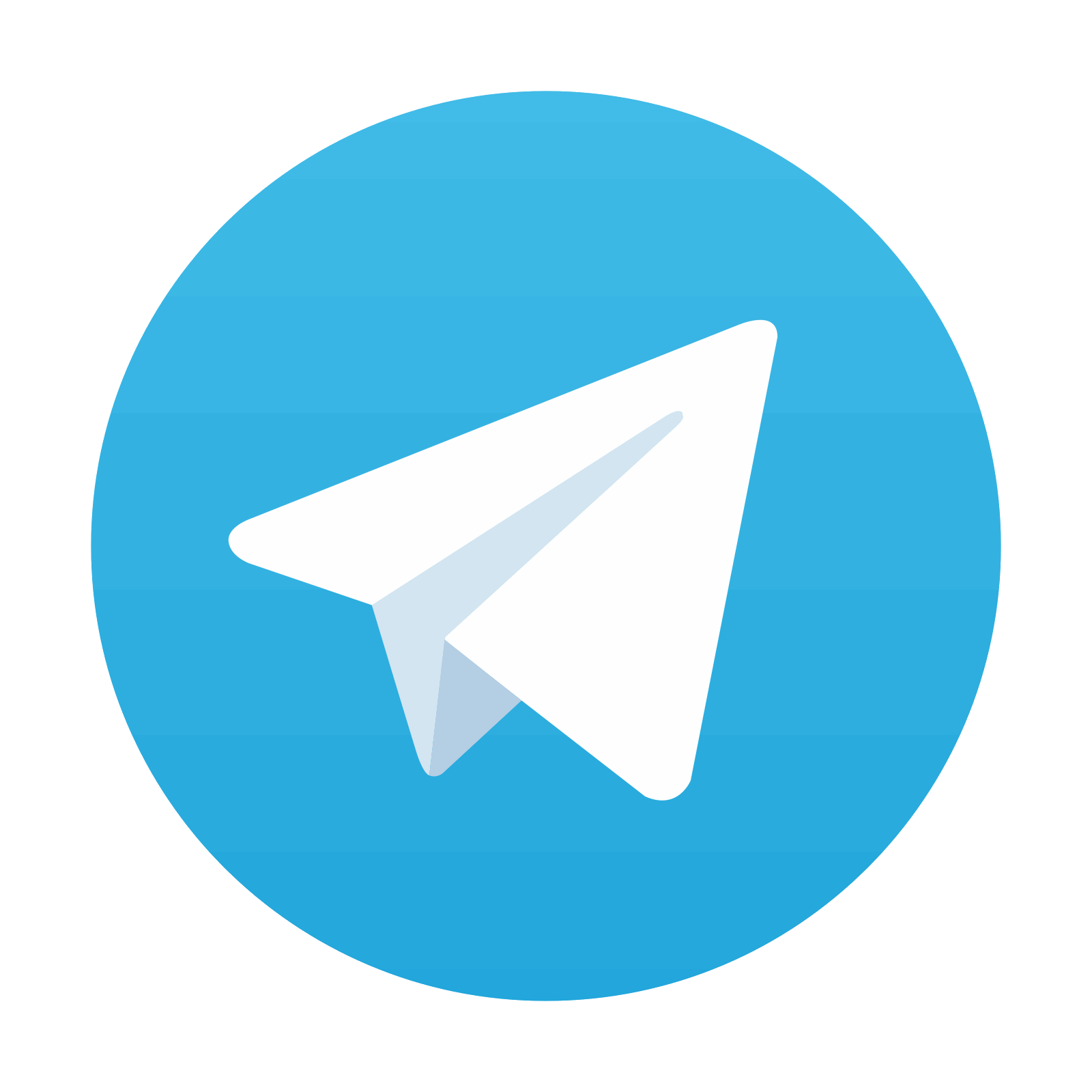
Stay updated, free articles. Join our Telegram channel

Full access? Get Clinical Tree
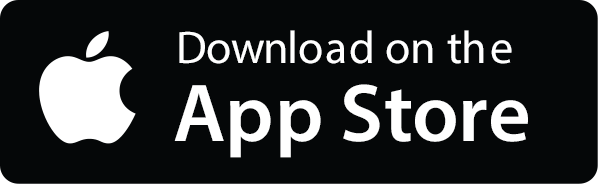
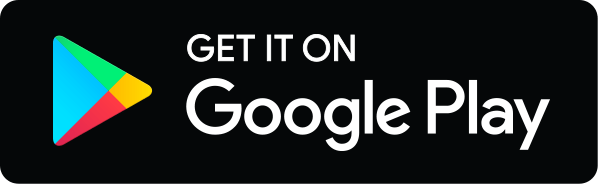