|
|
Neuro-ophthalmology is a field that helps elucidate the causes of visual symptoms, such as double vision (diplopia), and signs including pupillary dysfunction and eye movement abnormalities. Because these symptoms and signs can reflect lesions at multiple points along a complex path from the eye to the brain, or even more generalized systemic illnesses, it is important to take a careful history. The presenting details narrow the differential and guide the choice of specialized tests during the neuro-ophthalmologic examination. This examination focuses on factors including visual acuity, visual fields, the optic disc, pupillary function, eye movements, and eyelid elevation. Sometimes, a combination of findings forms a pattern that reflects a well-recognized syndrome.
The ability to localize lesions and identify syndromes to generate a plan for investigation and management depends on understanding the structures that play a role in visual systems. It is also important to comprehend how they interact with the external environment. The more anterior parts of the visual system (i.e., the eyes and optic nerves) allow exploration of visual space. The posterior cerebral hemispheres are involved in the integration and perception of visual information through higher cortical function—as discussed in Chapter 11.
ANATOMY OF VISUAL PATHS, PUPILS, AND OCULOMOTOR NERVES
Light enters the cornea and stimulates the rods and cones in the retina, generating electrical signals that are transmitted through the optic nerve. Fibers from the nerve of each eye cross at the optic chiasm before forming the optic tracts. Ninety percent of retinal axons in these tracts terminate in the lateral geniculate nucleus of the thalamus, the principal subcortical structure that carries visual information to the cerebral cortex through the optic radiations. The primary visual cortex is visual area 1, corresponding to Brodmann area 17 (striate cortex), which receives information from the contralateral visual hemi-field. This information is then transferred to the associative visual cortex, including areas 18 and 19, and to higher order centers in the posterior parietal and inferior temporal cortices, where the perception of motion, depth, color, location, and form takes place. The visual pathway and associated visual field defects are shown in Figure 4-1.
Pupil size is determined by parasympathetic and sympathetic influences. The pupil controls light transmission through the initial part of the visual pathway, including the optic tract. The optic tract synapses with the Edinger–Westphal nucleus (EWN) in the rostral aspect of the third nerve nucleus. Efferent parasympathetic fibers from the EWN travel with the third cranial nerve (CN III). In the cavernous sinus, they run with the inferior division of CN III and synapse in the parasympathetic ciliary ganglion in the posterior orbit. Parasympathetic fibers ultimately innervate pupilloconstrictor muscles in the iris (Fig. 4-2).
Sympathetic fibers effect pupil dilation via a three-neuron route. First-order neurons project down from the hypothalamus to an initial synapse in the intermediolateral cell column from C8 to T2 spinal levels (the “ciliospinal center of Budge”). Second-order neurons then travel from the sympathetic trunk to the superior cervical ganglion located near the bifurcation of the common carotid artery. Third-order neurons then travel in the adventitia of the internal carotid artery to join the first division of the trigeminal nerve to innervate elements of the orbit and eye, including the pupillodilator muscles (Fig. 4-3).
FIGURE 4-1. Visual pathways (figure, left; Note: this diagram is as if from an MRI scan: right side of brain on the left and left side of the brain on the right.) and visual field defects associated with lesions along the visual pathway (table, right). Light entering from the nasal visual field stimulates photoreceptors of the temporal retina, and vice versa. Photoreceptors transmit signals to bipolar cells and then retinal ganglion cells. Ganglion cell axons form the nerve fiber layer of the retina and enter the optic nerve (1 and 2). Each optic nerve carries visual information from one eye. (Arteritic and nonarteritic anterior ischemic optic neuropathy may be associated with altitudinal deficits.) The two optic nerves form the optic chiasm (3). Fibers from nasal halves of the retina (carrying information from the temporal visual fields) decussate at the optic chiasm and join temporal fibers (carrying information from the nasal visual fields) from the contralateral eye to form the optic tracts (4). Each optic tract carries visual information from the contralateral visual field. Each optic tract synapses with neurons at the lateral geniculate nucleus (LGN) on the same side (some fibers involved in the light reflex do not reach the LGN but go instead to the pretectal nucleus as part of the pupillary light reflex). From the LGNs, signals are transmitted through the geniculocalcarine tract (5, 6, and 7). Fibers that carry visual information from the upper contralateral visual fields travel through Meyer’s loop in the temporal lobes; fibers carrying visual information from the lower contralateral visual field travel through the parietal lobes. Visual information from the contralateral visual field reaches the occipital (calcarine) cortex (8). Strokes affecting the PCA may be associated with macular sparing because the macula is also supplied by the MCA. AMD, age-related macular degeneration; CRAO, central retinal artery occlusion; CRVO, central retinal vein occlusion; MCA, middle cerebral artery; PCA, posterior cerebral artery. Reprinted with permission from Chowdhury SH, Chowdhury JH, Cozma AI. Essentials for the Canadian Medical Licensing Exam: Review and Prep for MCCQE. 2nd ed. Philadelphia, PA: Lippincott Williams & Wilkins; 2016. Figure 15-5.
FIGURE 4-2. Eye movement abnormalities related to brainstem lesions of the abducens nerve or nucleus or both, and of the medial longitudinal fasciculus (MLF), causing deficits of eye movements in the horizontal plane. (The diagram depicts a coronal view of the brainstem, with midbrain structures above and pontine structures below.) (1) Lesion of the abducens nerve root: Motor neurons in the abducens nucleus innervate the ipsilateral lateral rectus muscle, so a patient with a lesion of the abducens root external to the pons has a loss of voluntary lateral gaze in the eye on the side of the lesion, with paralysis of the lateral rectus muscle. Other movements in the affected eye, and all movements in the contralateral eye, are normal. The patient will have diplopia. When looking straight ahead, the eye on the lesioned side will deviate slightly toward the midline (given the unopposed action of the medial rectus in the same eye). The diplopia is made worse when attempting to look toward the lesioned side in a horizontal plane. (2) Caudal basilar pontine lesion: Because axons arising from abducens motor neurons pass through the basilar pons, they are located laterally adjacent to corticospinal fibers. A lesion in this portion of the pons may simultaneously damage the exiting abducens fibers and corticospinal axons. A patient with this lesion may have a crossed hemiplegia, paralysis of the lateral rectus muscle on the side of the lesion (with loss of voluntary lateral gaze to that side, and diplopia), and a paralysis of the arm and leg on the opposite side of the body. (3) Internuclear ophthalmoplegia (INO): In addition to abducens motor neurons that innervate the ipsilateral lateral rectus muscle, the abducens nucleus also contains interneurons. The axons of these interneurons cross the midline, enter the MLF, and ascend to terminate on motor neurons in the oculomotor nucleus that innervate the medial rectus muscle on that side. A lesion in the MLF interrupts these axons and results in a loss of medial gaze (medial rectus paralysis) in the eye on the side of the lesion during attempted conjugate eye movements. Other movements in the affected eye and all movements in the contralateral eye are normal. (Thus, a right INO specifies a lesion in the right MLF and paralysis of the right medial rectus muscle.) (4) A lesion of the abducens nucleus damages α motor neurons innervating the ipsilateral lateral rectus muscle and the interneurons that terminate on medial rectus α motor neurons in the contralateral oculomotor nucleus. A patient with this lesion has loss of horizontal gaze in both eyes during attempted voluntary eye movement toward the side of the lesion; horizontal gaze toward the contralateral side is normal. (This is basically an abducens root lesion plus an INO.) (5) The one-and-a-half syndrome is so named because a unilateral pontine lesion may result in a loss of medial and lateral voluntary eye movement on the side of the lesion (the “one”) and a loss of medial horizontal eye movement on the contralateral side (the “one-half”). This lesion involves the abducens nucleus on one side (deficits = lateral rectus paralysis on the side of the lesion, medial rectus paralysis on the contralateral side) and the immediately adjacent MLF conveying the axons of abducens interneurons originating in the opposite abducens nucleus (deficit = medial rectus paralysis on the side of the lesion). These lesions are usually large and involve portions of the paramedian pontine reticular formation, commonly called the horizontal gaze center. Reprinted with permission from Haines DE. Neuroanatomy: An Atlas of Structures, Sections, and Systems. 8th ed. Philadelphia, PA: Lippincott Williams & Wilkins; 2011.
FIGURE 4-3. Parasympathetic pathway mediating pupillary constriction to light. The axons of the retinal ganglion cells project to the pretectal area. The neurons in the pretectal area send projections to the preganglionic parasympathetic neurons of the ipsilateral and contralateral Edinger–Westphal nuclei (EWN). The axons of the neurons in each EWN exit through the ipsilateral oculomotor nerve and project to the corresponding ciliary ganglion. The postganglionic fibers of the ciliary ganglion innervate the ciliary muscle. CN, cranial nerve.
●Visual perception depends on the transmission of signals that travel through a multi-step pathway including the retina, optic nerve, optic chiasm, optic tracts, lateral geniculate nucleus, and optic radiations on their way to the visual cortex.
●The primary visual cortex receives information from the contralateral visual hemi-field.
●Parasympathetic fibers from the Edinger–Westphal nucleus play an important role in pupil constriction.
●Sympathetic fibers travel in a three-neuron path from the hypothalamus to the intermediolateral cell column/ciliospinal center and then to the superior cervical ganglion before joining the first division of the trigeminal nerve to innervate pupil dilators.
●Extra-ocular movements are governed by six muscles served by CNs III, IV, and IV.
HISTORY
As in all branches of medicine, the history is key in characterizing symptoms such as eye pain, eyelid droop, and visual disturbance. It is important to ask about antecedent trauma. If there is no identifiable trigger, inquiring about the timing of symptom onset can be helpful. For example, the sudden onset of visual symptoms makes vascular causes more likely. Diurnal variations in symptoms can also provide a clue: Patients with myasthenia gravis often experience worsening double vision and eyelid droop at the end of the day. Exploring associated symptoms is important: Does the patient have concomitant limb weakness that could point to a stroke? Review of systems can uncover diagnostic hints such as prior transient neurologic deficits, which could point to vascular cause or multiple sclerosis. Similarly, medical and surgical history can be informative (e.g., diabetes can predispose to sudden, painful dysfunction of CN III, or less frequently, CN IV or VI). Prior cataract surgery can change pupillary reactivity and be associated with an eyelid droop. A review of recently used medications is essential because oral antiseizure drugs, topical agents such as apraclonidine (used for glaucoma), transdermal scopolamine, inhaled ipratropium, and injected botulinum toxin can cause symptoms and signs on exam. Habits such as alcohol ingestion are important to ask about because intoxication and vitamin deficiencies may be relevant. Finally, a family history of neurologic symptoms (e.g., gait imbalance or incoordination) raises the possibility of genetic disorders (e.g., spinocerebellar ataxia) that can be accompanied by visual deficits.
CHARACTERIZING COMMON CHIEF CONCERNS
DIPLOPIA
Neurologists and ophthalmologists commonly evaluate patients who report blurred or frankly double vision. Double vision results from a misalignment of the eyes, either as a decompensation of a previous strabismus or more commonly as a symptom of one of many neurologic disorders. To help narrow the differential, a pertinent history is important. Four questions are particularly worthwhile: (1) Does the double vision improve with closure of one eye? The aim is to determine if the lesion is monocular (involves one eye) or binocular (involves both eyes). Monocular visual loss implies a problem in the eye, optic nerve, or chiasm. Binocular visual loss reflects a chiasmal or retrochiasmal lesion. (2) Does the double vision emerge when looking in a particular direction (e.g., up, down, left, or right)? Answers help determine which extra-ocular muscles could be weak, as does the next query. (3) Do the two images appear side by side, one directly above the other, or with a skew? Finally, (4) Does the double vision worsen when looking at objects up close or far away? Whereas intact medial rectus (CN III) function is needed for near gaze, CN VI is needed for far gaze.
POSITIVE AND NEGATIVE VISUAL PHENOMENA
Visual disturbances, as with other sensory symptoms, can be described as negative or positive phenomena. Negative visual phenomena can be described as blackness, grayness, dimness, or a shade that obscures vision (i.e., amaurosis fugax), as seen in patients with strokes or transient ischemic attacks. When decreased vision is unilateral and associated with eye pain that worsens with movement, optic neuritis is an important consideration. Positive visual phenomena include brightness, shimmering, sparkling, shining, flickering, or colors, often suggesting migraine or seizures. In the Charles Bonnet syndrome, simple and complex, nonstereotyped hallucinations (including of scenes and people) occur in the setting of acquired visual loss. A “release phenomenon,” it is most common with chronic disease, impaired visual acuity, and known binocular disease (e.g., glaucoma). Altered perception of the external environment can also manifest as the illusion of movement (oscillopsia).
KEY POINTS
●Determine if an eyelid abnormality is congenital or acquired using the history and photographs.
●If there is diurnal variation, determine if the application of ice decreases the degree of ptosis, as can be seen in myasthenia gravis.
●Assess for other historical and clinical signs to characterize the ptosis.
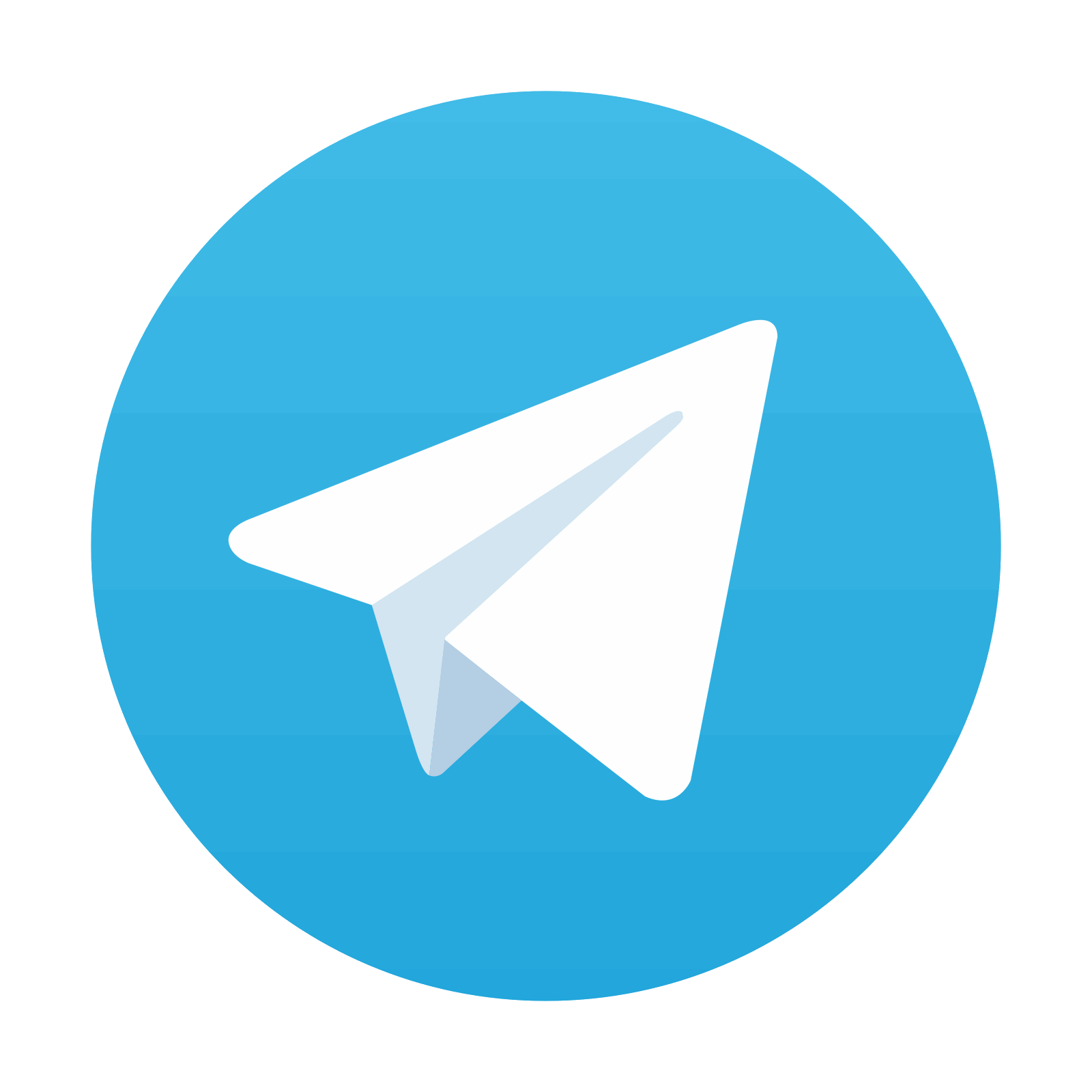
Stay updated, free articles. Join our Telegram channel

Full access? Get Clinical Tree
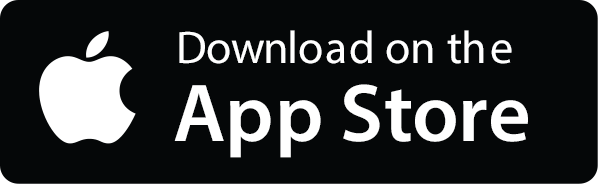
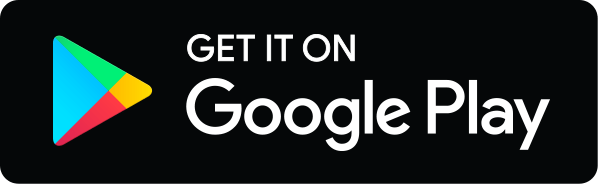