Neuroanatomical and Neurobiological Bases of Psychiatric Disorders
Hrvoje Hecimovic
Juan Santos
Frank Gilliam
Andres M. Kanner
Recent years have seen major advances in studying the neurobiological basis of psychiatric disorders. This is mostly due to the integration of basic science advances in neuroanatomy and neurochemistry of brain dysfunction and development of new clinical neuroimaging applications. The aim of this chapter is to review these findings and translate them into clinical setting.
Depression is the most prevalent psychiatric disorder that is complex in its neurobiology. Multiple studies tried to define its neurobiological mechanisms and genetic background and to understand the means by which environmental factors influence its onset, development, and severity of symptoms. Recent neuroimaging studies associated depression with specific cerebral structural and functional disturbances, suggesting that a dysfunction in neural networks underlie mood changes. Understanding neuroanatomy and interconnectivity of the structures, neurotransmitter pathways, and molecular mechanisms implicated in this dysfunction creates a basis to understand clinical expressions of the disease.
Anatomy of Frontolimbic Network
Current evidence suggests that hippocampal volume loss in humans is associated with depression (1), and functional imaging studies point to dysfunction in the frontolimbic network in patients with major depressive disorder (MDD). This is in line with earlier reports from animal, lesional, and human postmortem studies. Price (2,3) and others (4,5,6,7) provided evidence for the existence of a direct pathway from the hippocampus and subiculum to the specific structures in the prefrontal cortex. This pathway presents a model to study frontolimbic network dysfunction, which is associated with mood changes (8,9). Neuroanatomy of these structures is described in this text.
Hippocampus
Hippocampus is a structure with widespread connections that plays a central role in behavioral and other studies of mood, learning, and memory. This structure also has an
important function in stress response mediated through the hypothalamus-pituitary-adrenal (HPA) axis and in neuroplasticity.
important function in stress response mediated through the hypothalamus-pituitary-adrenal (HPA) axis and in neuroplasticity.
Hippocampal Afferent Projections
Hippocampal main afferents originate in the frontal lobe, Brodmann areas (BAs) 12, 13, and 25, occipital lobe (BA 19), and temporal lobe (BAs 20, 22, 35, 36, and 38). They provide inputs from the sensory systems by direct projections to the entorhinal cortex. This structure then projects further to the Ammon’s horn or the CA1, CA2, and CA3 layers. Neurons from the frontal lobe cortex (BAs 9 and 46) and parietal lobe (BAs 7 and 23) project directly to the Ammon’s horn. Information is then processed through a local hippocampal network from the dentate gyrus to the Ammon’s horn and to the subiculum. Further, visceral fibers from the basal and lateral nuclei of the amygdala project to the hippocampus through BA 28. Fibers from the anterior and midline thalamic nuclei pass through the cingulum to the entorhinal cortex, but one bundle also projects directly to the hippocampus. Projections from the supramammillary region of the hypothalamus pass through the fornix to the entorhinal area and the hippocampus proper (10,11) (Fig. 7.1).
![]() Figure 7.1 Hippocampal afferent pathways. Reproduced from Woolsey et al. (10) with permission. |
Hippocampal Efferent Projections
Most hippocampal efferents originate in the Ammon’s horn and the subiculum. The fibers from the subiculum then form the fornix, which is divided by the anterior commissure into the precommissural and postcommissural fornix. Axons in the precommissural
fornix synapse on the septal nuclei, nucleus accumbens, preoptic nucleus of the hypothalamus and the anterior olfactory nucleus and then project to the medial frontal cortex and the gyrus rectus including BAs 11, 12, 13, 25, and 32, respectively. Postcommissural fornix fibers project to the interstitial nucleus of the stria terminalis, anterior nucleus of the thalamus, and ventromedial and lateral mammillary nuclei of the hypothalamus. Additional fibers from the subiculum synapse directly in the basal and lateral nuclei of the amygdala, entorhinal cortex, and retrosplenial cortex, and some of them pass the cingulum and terminate in the cingulate cortex. Fibers from the Ammon’s horn terminate in the septal nuclei through the precommissural fornix (Fig. 7.2).
fornix synapse on the septal nuclei, nucleus accumbens, preoptic nucleus of the hypothalamus and the anterior olfactory nucleus and then project to the medial frontal cortex and the gyrus rectus including BAs 11, 12, 13, 25, and 32, respectively. Postcommissural fornix fibers project to the interstitial nucleus of the stria terminalis, anterior nucleus of the thalamus, and ventromedial and lateral mammillary nuclei of the hypothalamus. Additional fibers from the subiculum synapse directly in the basal and lateral nuclei of the amygdala, entorhinal cortex, and retrosplenial cortex, and some of them pass the cingulum and terminate in the cingulate cortex. Fibers from the Ammon’s horn terminate in the septal nuclei through the precommissural fornix (Fig. 7.2).
Amygdala
Current evidence suggests that the amygdala receives information from the sensory systems and the visceral afferents. This structure consists of five smaller nuclei organized in three groups: the central nucleus, the cortical and medial nuclei, and the basal and lateral nuclei, incorporating various functions.
![]() Figure 7.2 Hippocampal efferent pathways. Reproduced from Woolsey et al. (10) with permission. |
Amygdala and Afferent Projections
There are five main afferent pathways in the amygdala. Projections from the cerebral cortex and temporal lobe terminate in amygdalar nuclei: the insular fibers and the fibers from BAs 20, 21, 22, and 38 project to the central nucleus; and the insular fibers and the fibers from BAs 20, 21, 22, 35, 36, and 38 and some subiculum efferents project to the basal and lateral nuclei. Other subiculum projections terminate in the cortical and medial nuclei of the amygdala (10).
Further, axons from BAs 12, 13, 14, 23, 24, and 25 connect to the central nucleus, whereas those from BAs 11, 12, and 24 project to the basal and lateral nuclei. Axons from the interstitial nucleus of the
stria terminalis join the fibers from the ventromedial nucleus of the hypothalamus and lateral hypothalamic area and terminate in the central, medial, and cortical nuclei of the amygdala. Fibers from the olfactory bulb terminate in the medial and cortical amygdalar nuclei (Fig. 7.3).
stria terminalis join the fibers from the ventromedial nucleus of the hypothalamus and lateral hypothalamic area and terminate in the central, medial, and cortical nuclei of the amygdala. Fibers from the olfactory bulb terminate in the medial and cortical amygdalar nuclei (Fig. 7.3).
Amygdala and Efferent Projections
The five amygdalar nuclei project diffusely using four groups of axons. The central nucleus of the amygdala projects to the substantia innominata, nuclei of the diagonal gyrus, the lateral hypothalamic area, the interstitial nucleus of stria terminalis, and the septal nuclei (Fig. 7.4). Axons from the central nucleus also course through the medial forebrain bundle to reach their targets in the brainstem. In the midbrain, the fibers terminate in the parafascicular nucleus, ventral tegmental area, pars compacta of the substantia nigra, peripeduncular nucleus, periaqueductal gray matter, and the dorsal raphe nuclei. In the pons and medulla, the projections end in the superior central nucleus, lateral parabrachial nucleus, locus coeruleus (LC), nucleus subcoeruleus, raphe nuclei magnus, pallidus and obscurus, nucleus of the solitary tract, and the dorsal nucleus of the vagal nerve.
![]() Figure 7.3 Amygdalar afferent pathways MG, medical geniculate. Reproduced from Woolsey et al. (10) with permission. |
![]() Figure 7.4 Amygdalar efferent pathways. Reproduced from Woolsey et al. (10) with permission. |
Amygdalar efferents from the basal, lateral, medial, and cortical nuclei pass through the stria terminalis to innervate the interstitial nucleus of the stria terminalis and the paraventricular, anterior, ventromedial, and preoptic nuclei of the hypothalamus. Fibers from the basal and lateral nuclei that link with the stria terminalis also innervate the caudate nucleus and putamen, the nucleus accumbens, and the olfactory tubercle of the telencephalon. Further, some axons connect directly through the temporal lobe to the septal nuclei and the nucleus of the diagonal gyrus.
Prefrontal Cortex
Neuroanatomical, histological, and pharmacological studies suggest that the cortical afferents originating in the amygdala, hippocampus, and adjacent limbic structures are primarily directed to the specific structures of the orbital and medial prefrontal cortex, both in humans (3) and in monkeys (12,13,14). Various staining techniques and injections of specific tracers showed that there are more than 20 architectonic areas that can be differentiated within the orbital and medial cortex. Price et al. suggested that,
although each of these prefrontal regions has a distinct structure and connections, they can be grouped into two “networks” that interconnect and are defined by corticocortical connections within each network (15). The orbital network receives sensory inputs, including smell, taste, visceral afferents, somatic afferents, and vision, and appear to be particularly related to food and eating. In contrast, the medial network supplies the major cortical efferents to the visceromotor structures in the hypothalamus and periaqueductal gray. These networks have distinct connections with limbic structures, striatum, and mediodorsal thalamus. It also appears that rich interconnections between the two networks support information exchange from viscerosensory to visceromotor systems and vice versa.
although each of these prefrontal regions has a distinct structure and connections, they can be grouped into two “networks” that interconnect and are defined by corticocortical connections within each network (15). The orbital network receives sensory inputs, including smell, taste, visceral afferents, somatic afferents, and vision, and appear to be particularly related to food and eating. In contrast, the medial network supplies the major cortical efferents to the visceromotor structures in the hypothalamus and periaqueductal gray. These networks have distinct connections with limbic structures, striatum, and mediodorsal thalamus. It also appears that rich interconnections between the two networks support information exchange from viscerosensory to visceromotor systems and vice versa.
Neurotransmitters in Neurobiology of Affective Disorders
There is strong evidence that neurotransmitters play an important role in the modulation of brain dysfunction in mood disorders. However, it is unclear whether they present a primary modulating pathway or a final common pathway for the therapeutic effect of antidepressant drugs. Major neurotransmitters involved in this modulatory process are serotonin, norepinephrine (NE), dopamine (DA) and γ-aminobutyric acid (GABA).
Neurobiology of Serotonin
Approximately 30% to 80% of the neurons of the raphe nuclei synthesize serotonin. The secretion from the raphe nuclei is regulated with the 5-HT1A autoreceptors, located on the cell bodies. The raphe nuclei are localized along the midline of the brainstem and only the dorsal raphe nucleus lies in the ventral quadrant of the periaqueductal gray. The rostral group of nuclei includes the caudal linear, the median raphe, and the dorsal raphe nuclei with efferent projections directed rostrally. The caudal group, which includes the raphe magnus, the raphe obscurus, and the raphe pallidus nuclei and a cluster of neurons in the lateral reticular formation of the myelencephalon, project mainly to the caudal brainstem.
5-HT receptors are the most numerous and diverse of the monoamine transmitter system receptors. Projections of a single typical serotonergic neuron can reach distant brain areas that are in charge of various functions. This introduced a concept of the serotonergic system that exerts its action by modulating various other transmitter systems and by integrating and connecting other spatially distinct systems. The main role of the central 5-HT system is to regulate behavioral inhibitory system and it is implicated in the regulation of food intake, circadian rhythms, mood, anxiety, sleep, aggression, and impulsivity. Serotonergic dysfunction has been implicated in a number of neuropsychiatric disorders (Table 7.1).
At the cellular level, 5-HT receptors have been identified and classified on the basis of structural similarity and subdivided into seven families (16), as shown in Table 7.1. Each of them has a specific localization and distinct effector pathways. 5-HT1,2,4–7 receptor families are members of the G protein–coupled receptor superfamily. 5-HT3 receptor differs and belongs to the gated ionophore receptor superfamily. The largest family of the 5-HT receptors is the 5-HT1, with five 5-HT receptor subtypes. 5-HT1A receptors act as somatodendritic autoreceptors in the raphe nuclei and postsynaptically in the hippocampus, septum, and amygdala, where they initiate neuronal inhibition. They are also located on the astrocytes in the frontal and limbic cortex (19). A knockout transgenic mouse that lacks the 5-HT1A receptors has an increased sensitivity to anxiety that is caused by an increased serotonergic tone due to the failed negative feedback at the 5-HT1A autoreceptor (17).
Following its release, 5-HT that is present in the synaptic cleft is inactivated and transported back into the presynaptic terminal by the serotonin transporter (SERT). It is then either degraded by
monoamino-oxidase (MAO) to 5-hydroxy indoleacetic acid (5-HIAA), or repackaged into secretory vesicles through the SERT (18).
monoamino-oxidase (MAO) to 5-hydroxy indoleacetic acid (5-HIAA), or repackaged into secretory vesicles through the SERT (18).
TABLE 7.1 5-Hydroxytryptamine Receptors: Classification, Mechanisms of Action, and Pharmacotherapy | ||||||||||||||||||||||||||||||||||||||||||||||||
---|---|---|---|---|---|---|---|---|---|---|---|---|---|---|---|---|---|---|---|---|---|---|---|---|---|---|---|---|---|---|---|---|---|---|---|---|---|---|---|---|---|---|---|---|---|---|---|---|
|
Human SERT was recently cloned (20) and a number of biochemical and pharmacological studies have shown that this transporter exhibits voltage dependence and may alter excitability of the neurons (21). Decreased brain and platelet SERT binding is a frequent observation in depression (18), and some studies tried to examine if SERT dysregulation is part of the pathophysiology of depression. This is further suggested by recent findings that the tricyclic antidepressants (TCAs) and the newer selective serotonin reuptake inhibitors (SSRIs) also bind to the SERT. Therefore, it appears that most of the antidepressants used in clinical practice are potent SERT antagonists.
Neurobiology of Norepinephrine
NE is the primary sympathetic neurotransmitter in the periphery with abundant connections throughout the brain and the entire cortex. Cell bodies of NE reside predominantly in the LC of the dorsal pons. Many areas that are known to participate in the mediation of stress responses and autonomic functions, such as the paraventricular nucleus (PVN), the lateral hypothalamus, and the preoptic area, project their fibers toward the LC, adding further support for the theory that the NE system plays a role in stress and arousal; main LC efferents are cortical terminal fibers primarily ending in the layers I, IV, and V, suggesting that NE plays a role in modulating intracortical and thalamocortical transmission. These findings indicate that projections to the thalamus might have a role in coordinating the activity related to
levels of arousal, along with a role in sensory and motor gating. Projections to the thalamus are important in learning and memory, and hypothalamic fibers have a role in neuroendocrine effects related to the state of arousal (22).
levels of arousal, along with a role in sensory and motor gating. Projections to the thalamus are important in learning and memory, and hypothalamic fibers have a role in neuroendocrine effects related to the state of arousal (22).
Molecular studies showed that there are α and β classes of NE receptors. There are two subclasses of the α receptor, the α1 and α2, that are as distinct as the α and β classes. Several investigations using α2A– and α2C-adrenergic receptor knockout mice suggest that the α2A-adrenergic receptor is “stress-protective,” and the α2C-adrenergic receptor mediates stress susceptibility. All of the NE receptors belong to the G protein–linked receptor superfamily and therefore mediate the slower neuromodulatory postsynaptic responses.
NE system dysfunction includes changes in the NE metabolism in depressed patients (23,24). Studies showed that a prolonged exposure to stress decreases α2A-receptor density, mainly in the amygdala and the hippocampus. Further, LC, which is the main NE-containing nucleus in the brain, showed increased density of the α2-adrenergic receptors in suicide victims with a history of major depression. The results also showed increased activity of tyrosine hydroxylase in the LC in addition to decreased density of the NE transporter. Most of these effects are reproduced by NE depletion in the brain and to some extent reversed by antidepressant administration (25).
Neurobiology of Dopamine
DA is transported into storage vesicles in both DA- and NE-producing neurons through amine-specific transporters. NE-specific neurons, however, contain DA-β-hydroxylase within the vesicles that rapidly transforms DA to NE through hydroxylation of the β-carbon. After NE is released into the synaptic cleft following vesicle fusion, it is rapidly returned to the synaptic terminals through the NE transporter. These specific carriers are located on the outer membrane of the synaptic terminals. The NE transporter is inhibited by many classes of antidepressants that acutely increase synaptic NE concentration (22). L-Dopa has been shown to relieve mood symptoms in patients with Parkinson’s disease (26,27). DA reuptake inhibitors, such as bupropion, have been studied for their use as antidepressants (28). The potential role of DA in the neurobiology of depression is further supported by the regional specificity of dopaminergic projections from the ventral tegmental area to the prefrontal cortex, striatum, and the anterior cingulate. However, none of the studies clearly showed whether dopaminergic stimulation alone can alleviate depressive symptoms.
Neurobiology of γ-Aminobutyric Acid
It has been suggested that a GABA deficit in central neurons results in depressive symptoms, and low levels of GABA in the plasma and a decreased quantity of GABAB binding sites were found in the brain of patients, with and without depression, who committed suicide (29). Some investigators suggested that GABA has an inhibitory effect on the biogenic amine neurotransmitters and this inhibition may be implicated in the local network and interneurons. It has also been suggested that GABA deficit in mood disorders does not compete with, but complements, the hypothesis of alterations in NE and 5-HT function in mood disorders.
Models of Neurobiology of Depression
Monoaminergic Model of Depression
Early support for this hypothesis came from the observations that patients with a history of depression exposed to high doses of reserpine, which is a drug that depletes monoamine stores, had a recurrence of symptoms of depression (30). Dietary restrictions of tryptophan, which is a precursor of serotonin synthesis, cause a transient return of depression in patients who were
in remission (31). Following an exposure to α-methyl-paratyrosine (AMPT), an inhibitor of tyrosine hydroxylase, which is the enzyme responsible for the synthesis of catecholamines, an impairment in NE and DA synthesis was demonstrated with clinical recurrence of symptoms of depression (32). Therefore, most of this work focused on two monoaminergic, noradrenergic and serotonergic, neurotransmitter pathways that were described earlier. This also guided the development of most current antidepressants that act at the monoaminergic receptors.
in remission (31). Following an exposure to α-methyl-paratyrosine (AMPT), an inhibitor of tyrosine hydroxylase, which is the enzyme responsible for the synthesis of catecholamines, an impairment in NE and DA synthesis was demonstrated with clinical recurrence of symptoms of depression (32). Therefore, most of this work focused on two monoaminergic, noradrenergic and serotonergic, neurotransmitter pathways that were described earlier. This also guided the development of most current antidepressants that act at the monoaminergic receptors.
Cellular signaling pathways form complex networks to modulate signals generated by neurotransmitter and neuropeptide systems. One intracellular pathway that mediates effects of several classes of antidepressants is the cyclic adenosine monophosphate (cAMP) cascade, which is regulated by both 5-HT and NE (33). Receptor activation leads to the generation of the cAMP through the stimulation of adenylyl cyclase by the G protein subtype Gsα. The generation of the cAMP then results in activation of cAMP-dependent protein kinase (PKA) (34), and one of its substrates is the transcription factor cAMP response element binding protein (CREB). This protein, in its phosphorylated form, regulates transcriptional activity (35). Overexpression of CREB in the hippocampus results in an antidepressant effect and suggests that CREB may serve as a potential molecular target for therapeutic agents (Fig. 7.5).
Model of the Hypothalamic-Pituitary-Adrenal Axis Dysregulation
Activation of the HPA axis is important in understanding the neurobiology of depression. Several brain structures control the activity of the HPA axis, including the hippocampus (with an inhibitory effect on hypothalamic neurons) and the amygdala (with a direct excitatory influence) (33). Glucocorticoids from the circulation exert powerful feedback on the HPA axis, and under physiological conditions appear to enhance hippocampal inhibition of the HPA activity, and probably hippocampal function in general (37).
Neurons in the PVN of the hypothalamus secrete corticotrophin-releasing factor (CRF), which stimulates the synthesis and release of adrenocorticotropin (ACTH) from the anterior part of the pituitary gland. ACTH stimulates the synthesis and release of glucocorticoids from the adrenal cortex. Once glucocorticoids are in the circulation, they exert powerful feedback on the HPA axis, and under normal physiological conditions appear to enhance hippocampal inhibition of the HPA activity (37). However, prolonged increased concentration of glucocorticoids, for example during severe stress, is harmful and can damage hippocampal neurons, possibly by a reduction of dendritic branching and loss of dendritic spines that are included in glutamatergic synaptic inputs (38). Clinical studies show that excessive activation of the HPA axis is observed in almost half of the individuals with depression, and this effect is partially reversed by antidepressant treatment (39).
Recent reports (40,41) also suggested hypersecretion of the CRF in some depressed patients, which was reversed with antidepressant treatment. CRF is a major neuropeptide mediator of stress responses in the central nervous system (CNS) and is expressed in the PVN of the hypothalamus to coordinate the release of ACTH from the anterior pituitary (39). Levels of CRF are increased in the cerebrospinal fluid in suicide victims and in persons with depression. Two CRF receptors, CRF1 and CRF2, are abundant in the brain, and there is a hypothesis that both play a role in stress response (42,43).
Central administration of the CRF1 antisense oligodeoxynucleotides resulted in anxiolytic effects against both the CRF concentration and psychological stressors. There were also observations (18) that there is a downregulation in the CRF receptor number in the frontal cortex of depressed patients compared with controls. Further, chronic administration of CRF to normal volunteers resulted in HPA axis alterations
that were indistinguishable from those of the patients with major depression (40).
that were indistinguishable from those of the patients with major depression (40).
![]() Figure 7.5 Intracellular cAMP signaling pathway. 5-HT, 5-hydroxytryptamine; NE, norepinephrine; GDP, guanosine diphosphate; GTP, guanosine triphosphate; ATP, adenosine 5′-triphosphate; AMP, adenosine monophosphate; cAMP, cyclic adenosine monophosphate; PDE, phosphodiesterase; PKA, protein kinase; CREB, cAMP response element bindip; BDNF, brain-derived neurotrophic factor. Reproduced from Hecimovic et al. (36) with permission. |
Neurotrophic Model of Depression
The neurotrophic hypothesis of depression focuses on neurotrophic factors and their role in the neurobiology of depression and its treatment. Earlier studies described the role of neurotrophic factors in regulation of neuronal growth and differentiation during development. However, more recent studies have suggested their importance in plasticity and survival of adult neurons and glia (44). Brain-derived neurotrophic factor (BDNF) is a member of the nerve growth factor family, and it has been shown to increase synaptic strength, survival, and growth of mature neurons through activation of a transmembrane receptor that contains intrinsic tyrosine kinase B (TrkB) activity.
In animals, stress decreases levels of BDNF expression in the dentate gyrus and pyramidal cell layer of the hippocampus (45). This reduction appears to be mediated by glucocorticoid action and
by increases in serotonergic transmission. Chronic, but not an acute, administration of antidepressant drugs or chronic electroconvulsive treatment increased BDNF expression and prevented a stress-induced decrease in BDNF levels (46). Some groups (47) found in postmortem studies increased BDNF expression in dentate gyrus, hilus, and supragranular regions in subjects treated with antidepressant medications, compared with subjects not treated with antidepressants. Induction of the BDNF by antidepressants is at least partly mediated through the CREB.
by increases in serotonergic transmission. Chronic, but not an acute, administration of antidepressant drugs or chronic electroconvulsive treatment increased BDNF expression and prevented a stress-induced decrease in BDNF levels (46). Some groups (47) found in postmortem studies increased BDNF expression in dentate gyrus, hilus, and supragranular regions in subjects treated with antidepressant medications, compared with subjects not treated with antidepressants. Induction of the BDNF by antidepressants is at least partly mediated through the CREB.
It has been suggested that chronic antidepressant treatment upregulates the cAMP cascade in hippocampus and cerebral cortex, increases levels of cAMP-dependent PKA, and enhances expression of the CREB (34,35). These observations indicate that agents that activate this pathway could potentially be useful in treatment of depression. cAMP-specific phosphodiesterase (PDE4) is responsible for the breakdown of cAMP, and phosphodiesterase inhibitors were shown to prevent cAMP degradation (48). A proposal that phosphodiesterase inhibitors can theoretically reduce symptoms of depression generated another strategy for antidepressant drug development.
Neural Networks of Mood Dysfunction
Structural Magnetic Resonance Imaging Studies
Current evidence suggests structural brain abnormalities in patients with unipolar and bipolar depression (Table 7.2). Most of the studies in patients with MDD showed reduced hippocampal volume that is associated with early age of onset (49), number of previous episodes (50), or longer duration of untreated depression (51). However, there were also some studies that failed to determine any association. Reports of hippocampal volume change in bipolar disorder (BD) have also been contradictory. There have been reports of increases (52), decreases (53), or no change in the hippocampal volume (54). None of the studies showed any significant differences in the total intracranial volume between patients with depression and healthy subjects. Nevertheless, most authors use relative measurements for comparison of regions of interest (ROIs) or a total intracranial volume as a covariate in the statistical analysis.
Volume reductions in MDD were further reported in the prefrontal cortex, mainly in the anterior cingulate and the gyrus rectus. Inconsistent results were found in the amygdala volumes. Studies showed either a reduction in the left amygdala volume (71,81), asymmetry of the structure (69), an increase in bilateral amygdala volume after the first episode of MDD (83), or a reduction in the bilateral core nuclei (82). Some groups measured hippocampal-amygdalar complex as a single structure and found no change in its volume in comparison with healthy subjects (63,64). Volume examination of the basal ganglia found decreased left caudate (84) or putamen (85), or no change in the volume (86). Most of the studies that assessed white matter changes in people with MDD found an increase in the white matter hyperintensities either periventricularly or more frontally (94).
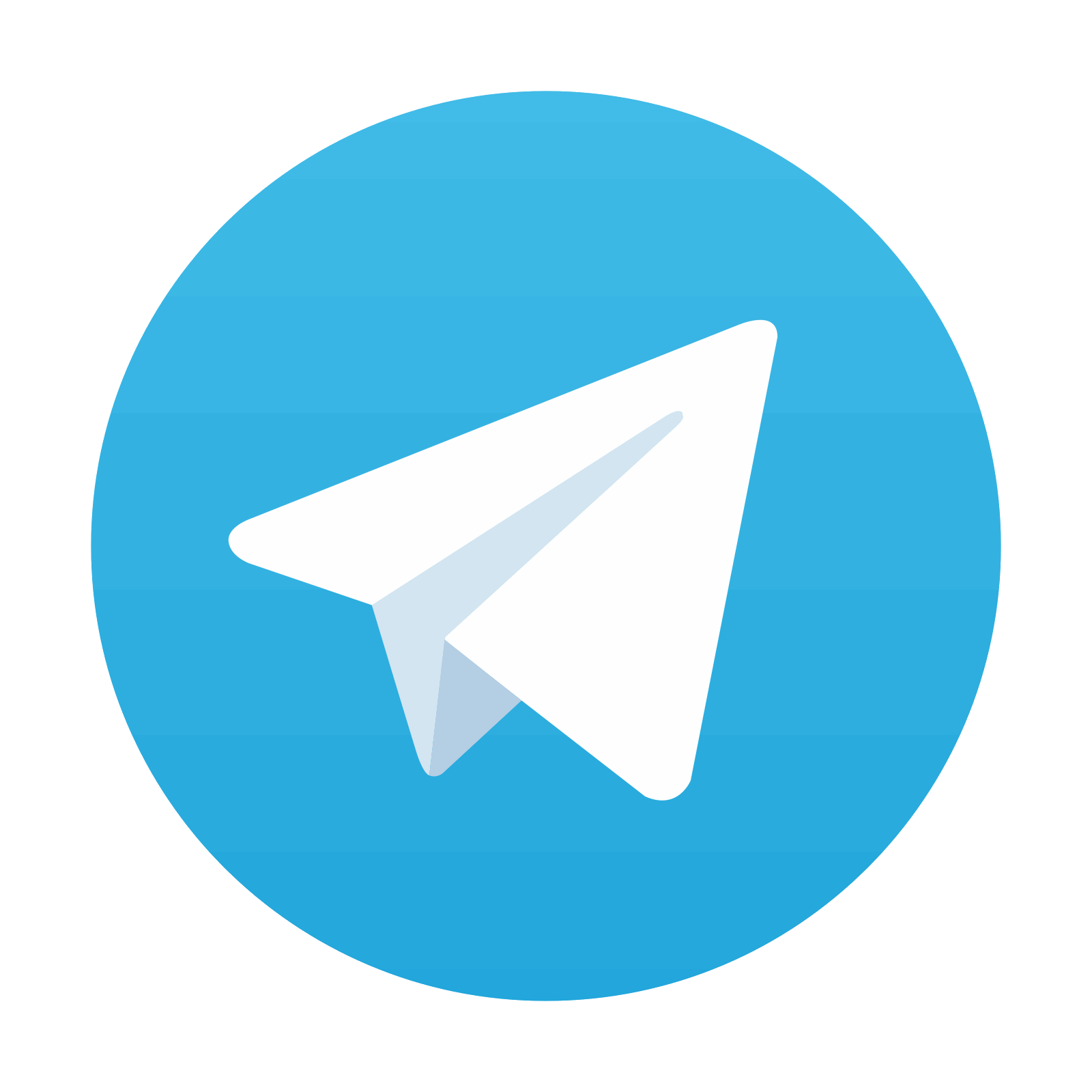
Stay updated, free articles. Join our Telegram channel

Full access? Get Clinical Tree
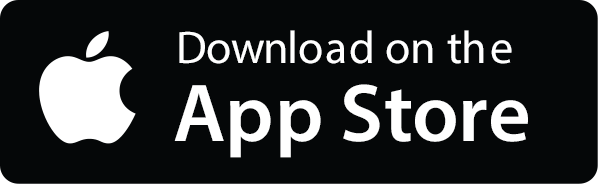
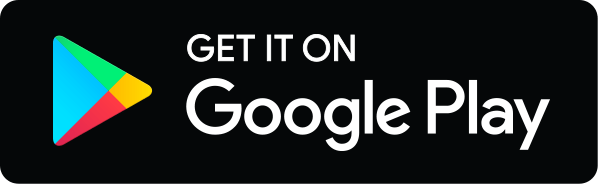