1 Note: Significant diseases are indicated in bold and syndromes in italics. 1. Important gyri, lobes, and Brodmann areas (Fig. 1–1) 2. Types of cortical neurons (Fig. 1–2) a. projection neurons: pyramidal and fusiform neurons b. interneurons: stellate and granule cells, horizontal cells of Cajal 3. Chemoanatomy a. afferents i. corticocortical fibers and thalamocortical fibers (glutamate, aspartate) ii. projections from the nucleus basalis of Meynert (acetylcholine), hypothalamus (histamine, orexin/hypocretin), and brainstem (norepinephrine, serotonin, dopamine) b. efferents i. all use glutamate and aspartate 4. Subtypes of cortex a. cytoarchitectonic subtypes i. isocortex: exhibits the six typical layers (1) homotypical isocortex: the six layers are proportionate (2) heterotypical isocortex: certain layers are enlarged, for example, the (a) primary motor cortex (Brodmann area 4) exhibits an enlarged layer 5 due to oversized pyramidal cells that project into the corticospinal tract {Betz cells} Figure 1–1 Important gyri, lobes, and Brodmann areas. (A) lateral surface; (B) medial surface. (From Duus P, Topical Diagnosis in Neurology. Stuttgart, Germany: Georg Thieme; 1998:276, Fig. 8.23. Reprinted by permission.) ii. mesocortex: exhibits five or six poorly defined layers, located at transition between isocortex and allocortex (e.g., parahippocampal gyrus) iii. allocortex: exhibits only three layers; includes the inferior and mesial temporal lobes (hippocampal formation) b. general functional subtypes i. primary motor and sensory areas ii. monomodal association areas (1) monomodal motor association areas: receive input from the primary and monomodal association sensory areas, and project to the primary motor area (2) monomodal sensory association areas: receive input from the primary sensory area, and project to the heteromodal association areas and primary motor area iii. heteromodal association areas: receive input from monomodal association areas; project to other heteromodal association and paralimbic areas, and the basal ganglia iv. limbic and paralimbic areas Figure 1–2 Types of cortical neurons. (From Duus P, Topical Diagnosis in Neurology. Stuttgart, Germany: Georg Thieme; 1998:262, Fig. 8.11. Reprinted by permission.) 1. Primary motor cortex (Brodmann area 4) a. somatotopic organization (Fig. 1–3) b. lesions strictly limited to Brodmann area 4 produce focal paralysis with hypotonia, mild hyperreflexia, and a partial Babinski sign (i.e., upgoing first toe only) i. extending the lesion into the premotor area (lateral surface of Brodmann area 6) produces the typical “clasp knife” spasticity of the antigravity muscles (upper extremity flexors and lower extremity extensors), pronounced hyperreflexia, and a complete Babinski sign (i.e., upgoing first toe with fanning of the other toes) c. stimulation causes simple flick-like focal movements involving a few agonist and antagonist muscles 2. Monomodal motor association areas: share the precentral sulcus with the primary motor cortex, and extend rostrally onto the superior, middle, and inferior frontal gyri a. premotor area (lateral surface of Brodmann area 6): prepares the body posture for subsequent complex limb movements; acts via the rubrospinal, tectospinal, and reticulospinal tracts i. shares the homunculus of the primary motor cortex: the frontal eye fields (Brodmann area 8) are located anterior to head representation b. supplementary motor area (medial surface of Brodmann area 6): prepares and initiates complex limb movements; likely exhibits its own homunculus independent of that of the premotor area and primary motor cortex i. lesions cause Figure 1–3 Sensory (A) and motor (B) homunculi. Note the proximity of the thumb to the forehead. (From Duus P, Topical Diagnosis in Neurology. Stuttgart, Germany: Georg Thieme; 1998:273, Fig. 8.20. Reprinted by permission.) (1) global akinesia with speech arrest that develops into hypokinesia (contralateral > ipsilateral), reduced speech, and reduced facial expression; chronically, patients will exhibit only poor hand coordination (2) ideomotor apraxia, which may be associated with a contralateral alien hand phenomenon characterized by unconscious reflexive movements ii. stimulation causes bilateral posturing movements, contralateral head and body rotation, and vocalizations {salutary seizures} 3. Subcortical projections a. corticobulbar tract: the ventral division travels with the corticospinal tract and synapses in the facial nucleus; the dorsal division travels closer to the medial lemniscus and synapses in other cranial nerve nuclei and the reticular formation b. corticospinal tract: composed of fibers not only from the primary motor cortex (25%) and monomodal motor association cortex (30%) but also the primary somatosensory cortex (30%) and superior parietal lobule (Brodmann areas 5 and 7; 15%) i. terminates in the cervical spinal cord (55%), thoracic spinal cord (20%), and lumbosacral spinal cord (25%) ii. the majority (80%) of the corticospinal tract decussates at cervicomedullary junction (upper extremity fibers decussate rostral to the lower extremity fibers) (1) the rest of the corticospinal tract does not decussate until it arrives at its terminal cervical and upper thoracic spinal cord levels (ventral corticospinal tract) 1. Primary sensory cortex (Brodmann areas 3-1-2) a. subdivided according to sensory modality i. Brodmann area 3a receives deep sensation from muscle spindles and Golgi tendon organs ii. Brodmann area 3b receives superficial sensation from exteroceptors and cutaneous receptors iii. Brodmann areas 1 and 2 receive deep and superficial sensation including subcutaneous tissues b. somatotopic organization: similar to the motor homunculus, but less well defined and may extend caudally into Brodmann areas 5 and 7 i. face and body are bilaterally represented; limbs are unilaterally represented c. lesions cause loss of proprioception (but not vibration sensation), poor localization of stimuli {topagnosia}, difficulty with two-point discrimination, reduced object recognition through touch {astereognosis}, tactile hallucinations, rapid fatigability of prolonged sensory stimulation, and paresthesias i. primary modality sensory loss is pronounced with postcentral gyrus lesions that involve the posterior insula and underlying white matter d. stimulation produces numbness and paresthesias, and often a sensation of movement 2. Secondary sensory cortex (supramarginal gyrus; Brodmann area 40): Posterior to the inferior part of the primary somatosensory cortex a. exhibits bilateral inputs with contralateral predominance, and is somato-topically organized into a separate homunculus that may be related to pain sensation 3. Unimodal somatosensory association area (Brodmann area 5): Involved in recognition of object shape by tactile sensation {stereognosis} 4. Subcortical afferents: anterolateral system/spinothalamic tract, dorsal columns, and trigeminal pathways terminate in the ventroposteromedial and ventroposterolateral thalamic nuclei that then project to the primary sensory cortex 1. Vision (see p. 37) and hearing (see p. 48) 2. Vestibular sensation (see p. 50) 3. Taste (Brodmann area 43): Adjacent to the primary somatosensory tongue representation and the insular cortex motor areas for salivation and gastrointestinal motility a. distorted taste perception {dysgeusia} is poorly localized and usually is caused by zinc or vitamin A deficiency 4. Olfaction: represented by bilateral projections from the olfactory nerves to the piriform cortex and amygdala via lateral olfactory stria, and to the orbitofrontal cortex overlying the septal nuclei via the medial olfactory stria; no olfactory bulb projections enter the anterior perforated substance in humans a. distorted odor perception {parosmia} is usually caused by injury to the olfactory nerves from head trauma or by psychiatric illness (e.g., depression) b. olfactory hallucinations can be caused either by injury to the olfactory nerves or by seizures in the mesial temporal lobe or uncus that involve the amygdala (Box 1.1) Other Types of Hallucinations Visual—Formed hallucinations from posterior temporal lobe or mesencephalon (i.e., peduncular hallucinosis) dysfunction or in reaction to vision loss {Bonnet’s syndrome}, which have hallucinations in the area of vision loss; unformed hallucinations with occipital lobe dysfunction Auditory—Formed hallucinations from superior temporal gyrus dysfunction; unformed hallucinations from pontine dysfunction, usually in the presence of hearing loss Gustatory—from frontoparietal cortex dysfunction 1. Frontal heteromodal association area/prefrontal cortex: involves all the cortex located anterior to the monomodal motor association areas a. unlike other heteromodal association areas, the prefrontal cortex i. projects to the amygdala and caudate ii. receives indirect projections from the hippocampus through the dorsomedial thalamus and direct projections from the cingulate gyrus and hypothalamus
Neuroanatomy
I. Cerebral Cortex
A. Motor Systems
B. Somatosensory Systems
C. Special Sensory Systems
Box 1.1
D. Heteromodal Association Areas
Prefrontal cortex region | Effects of unilateral damage | Effects of bilateral damage |
Dorsolateral | Dominant: ↓ verbal intellect and memory; perseveration Nondominant: ↓ visuospatial intellect and memory | Global intellectual impairment (dementia) |
Ventromedial | Dominant: labile emotions, sociopathy, environmental dependency (i.e., the presence of an object induces its use); speech apraxia, gait apraxia Nondominant: sociopathy, environmental dependency | Sociopathy, environmental dependency |
Anterior cingulate | Inability to perform tasks or make decisions {abulia} | Akinetic mutism |
Orbitofrontal | Disinhibited behavior |
|
b. lesions: in addition to the injured part of the prefrontal cortex, the depth of the lesion and the age at which the patient was injured will also affect the symptoms (Table 1–1)
i. Bruns’ frontal lobe ataxia/marché à petits pas of Dejerine—wide-based, slow, short-stepped gait with flexed posture and frequent freezes; can progress to inability to stand
(1) caused by lesions anywhere in frontal lobes that are often bilateral and multifocal
2. Parietal heteromodal association areas: Located in the superior and inferior parietal lobules
a. dominant-sided lesions produce (Box 1.2)
i. alexia
ii. Gerstmann’s syndrome—classically involves agraphia, finger agnosia, acalculia, and left-right confusion; lesions are located on the angular gyrus (Brodmann area 39)
iii. contralateral tactile neglect and spatial inattention
iv. somatotopic ideomotor apraxia
b. nondominant-sided lesions produce
i. contralateral tactile neglect and spatial inattention, which may be so severe that the patient does not recognize the deficit {anosognosia}
ii. constructional and dressing apraxias
iii. spatial disorientation
iv. impersistence; inattention; static confusional state/encephalopathy
E. Language Areas
1. Dominant hemisphere language areas
a. Broca’s area: located in the inferior frontal gyrus, and includes the pars opercularis (Brodmann area 44; a monomodal motor association area) (Box 1.3) and the pars triangularis (Brodmann area 45; a heteromodal association area)
Box 1.3
Bilateral damage of Brodmann area 44 that extends into the inferior parietal lobe causes loss of voluntary eye, face, oropharynx, and tongue movements (an apraxia).
i. lesions produce
(1) Broca’s aphasia—classically involves Broca’s area, the mouth representations of the primary motor and somatosensory cortices, and the underlying white matter
(a) symptoms include nonfluent speech that is slow and agrammatic as well as dysarthric, and impaired repetition; writing is minimal and agrammatic
b. Wernicke’s area
i. includes posterior superior and middle temporal gyri, planum temporale (Brodmann area 42; a monomodal auditory association area), and heteromodal association areas of the parietal lobe (angular and supra-marginal gyri; Brodmann areas 39 and 40)
ii. lesions cause Wernicke’s aphasia
(1) characterized by excessive speech of normal cadence and prosody associated with impaired naming, comprehension, and repetition, and the presence of paraphasias and neologisms; writing is similarly excessive with paraphasias and neologisms
(2) impairment of comprehension can be particularly pronounced with specific subjects, thereby giving the false appearance of a fluctuant deficit
c. secondary language areas (i.e., the cortex surrounding Broca’s and Wernicke’s areas): lesions produce the transcortical aphasias because they isolate the primary language areas without directly injuring them; all have preservation of repetition
i. subtypes of transcortical aphasias
(1) transcortical motor aphasia—generally caused by lesions anterior or superior to Broca’s area, or by lesions in the supplementary motor area
(2) transcortical sensory aphasia—can be caused by lesions surrounding Wernicke’s area, but otherwise is usually poorly localized
(3) mixed transcortical aphasia—caused by large areas of dominant hemisphere injury that spare the perisylvian regions (e.g., as in watershed infarction or dementias)
d. subcortical connections of Broca’s and Wernicke’s areas: lesions produce
i. conduction aphasia—caused by disconnection of Broca’s and Wernicke’s areas, most commonly the result of a subcortical white matter lesion that interrupts the arcuate fasciculus (in the superior longitudinal fasciculus; and/or the extreme capsule
(1) symptoms: isolated loss of repetition with occasional paraphasic errors
ii. anomia—generally has no localizing value when mild, but significant anomia may reflect lesions in the basal temporal lobe that interrupt hippocampal projections to Wernicke’s area
2. Nondominant hemisphere language areas
a. areas related to the variation of pitch, intonation, melody, rhythm, and loudness of speech {prosody}
i. affective prosody—involves the expression of mood and emotion in speech; caused by lesions in the nondominant hemisphere in areas that are anatomical and functional parallels to Broca’s and Wernicke’s areas of the dominant hemisphere
(1) affective prosody is most commonly observed in psychiatric diseases (e.g., motor and sensory aprosodies in schizophrenia, motor hyperprosody in mania)
ii. propositional prosody—defines a phrase as a question or a statement; can occur after lesions of either hemisphere
b. areas related to gesturing that accompanies speech {kinesia}: poorly localized
c. areas related to singing: an acquired inability to sing {amelodia} is caused by lesions of the posterior inferior frontal lobe or anterior parietal lobe
F. Paralimbic Cortex
1. Paralimbic cortex: collects inputs from heteromodal association areas, monomodal association areas, and limbic areas (hippocampal formation and amygdala); projects primarily to the hippocampal formation
2. Paralimbic cortex includes
a. anterior and posterior cingulate gyrus
b. parahippocampal gyrus (entorhinal cortex and piriform/periamygdaloid cortex)
c. insular and orbitofrontal cortex
d. temporal poles: lesions cause
i. Kluver-Bucy syndrome—occurs only after bilateral anterior temporal cortex destruction, usually from temporal lobectomy; does not have to involve the amygdala or hippocampus to produce all of its symptoms
(1) symptoms (Box 1.4)
Box 1.4
Placidity, hypersexuality, and hyperorality are the classic triad that occurs in animal experiments.
(a) placidity
(b) hypersexuality
(c) hyperorality and increased manual exploration, which likely reflects an inability to recognize objects by vision alone {visual agnosia}
(d) memory impairment, dementia
(e) hyperphagia
ii. rage—caused by bilateral anterior and inferior temporal lesions, usually due to trauma or herpes encephalitis
G. Limbic Areas
1. Hippocampal formation: includes the hippocampus, dentate gyrus, and subiculum
a. afferent and efferent connections: the circuit of Papez (Fig. 1–4)
b. intrinsic hippocampal connections (Fig. 1–5)
c. functions: memory formation, learning, and regulation of emotional behavior
d. pathophysiology—transient global amnesia
i. an unknown type of neurological dysfunction, likely involving bilateral mesial temporal lobes
ii. epidemiology: common only in patients > 50 years old; associated with a history of migraine
iii. symptoms: short-term anterograde and retrograde memory loss with preserved immediate recall and long-term memory; often develops acutely after exertion or excitement
(1) patient has a normal level of arousal and attention, and no of other neurological signs
iv. diagnostic testing: none are necessary to establish the diagnosis
Figure 1–4 The circuit of Papez. (From Duus P, Topical Diagnosis in Neurology. Stuttgart, Germany: Georg Thieme; 1998:206, Fig. 5.20. Reprinted by permission.)
Figure 1–5 Intrinsic hippocampal connections. (From Kahle W, Color Atlas/Text of Human Anatomy, Vol. 3: Nervous System and Sensory Organs. 4th ed. Stuttgart, Germany: Georg Thieme; 1993:221, Fig. A. Reprinted by permission.)
(1) electroencephalogram (EEG): can rule out nonconvulsive temporal lobe seizures
(2) magnetic resonance imaging (MRI) may exhibit increased diffusion-weighted imaging signal in the mesial temporal lobes
v. treatment: none specific
vi. prognosis: gradual return of memory over 12–24 hours; recurs in 20% of patients
2. Amygdala
a. subdivisions
i. corticomedial amygdala: interconnected with the olfactory bulb via the lateral olfactory tract, the hypothalamus, and the septum via the stria terminalis
ii. basolateral amygdala: interconnected with the hippocampal formation, ventral basal ganglia (limbic loop, see Table 1–2), and basal forebrain
iii. central amygdala: interconnected reciprocally with brainstem autonomic centers (e.g., solitary nucleus)
i. olfactory sensory processing and integration of olfactory-visceral reflexes
ii. coordination of emotional behavior and autonomic nervous system responses
iii. association of memories with their emotional content
c. lesions generally produce a loss of aggressive behavior; stimulation produces fear or defense reactions
II. Subcortical White Matter
1. Corona radiata/Centrum semiovale
a. pathophysiology
i. leukoaraiosis: can be part of, but is not identical to, vascular dementia or Binswanger’s disease
(1) lesion types include (Fig. 1–6)
Figure 1–6 Leukoaraiosis. (From Hosten N, Liebig T, CT of the Head and Spine. Stuttgart, Germany: Georg Thieme; 2002:31, Fig. 1.33b. Reprinted by permission.)
(a) irregular white matter abnormalities, typically scattered about randomly
(i) histology: resembles ischemia
(b) periventricular white matter caps and halos
(i) histology: resembles demyelination (not ischemia), with subependymal gliosis and breakdown of the underlying ependyma of the ventricles
b. internal capsule (Fig. 1–7)
i. pathophysiology: the striatocapsular syndrome, typically caused by infarction from occlusion of a lenticulostriate artery; symptoms include
(1) hemiparesis (95%), which is often the only symptom {pure motor syndrome} (Box 1.5)
Box 1.5
Differentiate striatocapsular syndrome from primary motor cortex lesions that may allow reflex movements of the apparently paralyzed limb (mediated by monomodal motor association areas).
(2) hemi-sensory loss (60%)
(3) aphasia or neglect (60%)
c. association fibers
i. short association fibers/“U” fibers: short-range cortical–cortical projections
ii. long association fibers (Fig. 1–8)
(1) cingulum: connects the temporal and prefrontal heteromodal cortices with the cingulate gyrus
(2) uncinate fasciculus: connects the temporal and prefrontal heteromodal cortices
Figure 1–7 Internal capsule. (From Greenberg MS, Handbook of Neurosurgery. 3rd ed. Greenberg Press; 1994:114, Fig. 23.12. Reprinted by permission.)
Figure 1–8 Long association fibers. (A) Lateral view; (B) transected hemisphere at the level of the insula. (Left, from Mumenthaler M, Neurological Differential Diagnosis. 2nd ed. Stuttgart, Germany: Georg Thieme; 1992:49, Fig. 18 and right, from Kahle W, Color Atlas/Text of Human Anatomy, Vol. 3: Nervous System and Sensory Organs. 4th ed. Stuttgart, Germany: Georg Thieme; 1993:247, Fig. D. Reprinted by permission.)
(3) superior longitudinal fasciculus: connects the parietal and temporal lobes with the frontal lobe; includes the arcuate fasciculus, lesions of which cause conduction aphasia
(4) inferior longitudinal fasciculus: connects the occipital cortex with the inferior temporal and fusiform gyri; important for the ventral occipitotemporal neural network, which is involved in face and object recognition (the “what” pathway)
(5) superior occipitofrontal fasciculus: interconnects all the lobes; important for the dorsal parietofrontal neural network, which is involved in spatial orientation (the “where” pathway)
(6) inferior occipitofrontal fasciculus: connects the inferior temporal, fusiform, and lingual gyri with the frontal lobe
d. commissures
i. corpus callosum: regions of the cortex project through the corpus callosum to symmetric regions in the contralateral hemisphere, and occasionally to other regions; fibers originate from cortex layer 3
(1) pathophysiology
(a) transection of the corpus callosum: symptoms are generally detectable only by specific testing, and include
(i) inability to name objects or read words presented in the visual hemifield of the nondominant hemisphere
(ii) inability to name objects felt by the nondominant hand
(iii) inability to write with the nondominant hand
(iv) impaired spatial construction skills with the dominant hand
(v) callosal alien hand syndrome—discoordination of simultaneous voluntary hand movements
ii. anterior commissure: connects the caudal orbitofrontal, anterior temporal, and parahippocampal cortices as well as the contralateral olfactory nerve to the piriform cortex for bilateral olfactory representation
iii. posterior commissure: carries fibers of the medial longitudinal fasciculus, posterior thalamus, pretectal nuclei, mesencephalic accessory ocular motor nuclei, and superior colliculi
iv. hippocampal commissure: minimal size and function in humans
Figure 1–9 The basal forebrain posterior to the nucleus accumbens demonstrating the olfactory cortex, septal nuclei, and ventral basal ganglia (inferior to the anterior commissure). (From Kahle W, Color Atlas/Text of Human Anatomy, Vol. 3: Nervous System and Sensory Organs. 4th ed. Stuttgart, Germany: Georg Thieme; 1993:201, Fig. B; 211. Reprinted by permission.)
III. Basal Forebrain (Fig. 1–9)
1. Septal region: Includes
a. septal nuclei: the lateral septal nucleus receives input from the medial olfactory stria, the hypothalamus, and the hippocampus via the fimbria-fornix, and relays that information to the medial septal nucleus; projections from the medial nucleus (acetylcholinergic as well as GABAergic) go to the hippocampus via the fornix and to the brainstem via the medial forebrain bundle
b. nucleus of the diagonal band of Broca, which provides acetylcholinergic innervation to the hippocampus as do the septal nuclei
c. nucleus accumbens: the equivalent of the caudate and putamen for a limbic basal ganglia loop (Table 1–2); receives dopaminergic afferents from the ventral tegmental area and is involved in positive reinforcement and craving behaviors
d. islets of Calleja, which have a poorly defined function
2. Nucleus basalis of Meynert: Similar to the septal nuclei but provides acetylcholinergic innervation to the cortex (particularly pronounced in limbic and paralimbic areas) and extrinsic cholinergic innervation to the striatum (although 80% of striatal acetylcholine is intrinsic) and not to the hippocampus; functions in memory formation and retrieval
IV. Basal Ganglia (Fig. 1–10)
1. Striatum: Includes the caudate, putamen, and nucleus accumbens; some also include the claustrum
a. neuron types: the motor striatum (caudate and putamen) and the limbic striatum (nucleus accumbens) are cytologically identical
i. spiny neurons: project from the striatum to the globus pallidus and substantia nigra
(1) nondopaminergic inputs to the spiny neurons terminate on the tips of their spines; dopaminergic inputs terminate on the spine bases, thereby modulating the efficiency of the nondopaminergic inputs
(a) type I spiny neurons: contain GABA and enkephalin, and express D2 receptors; act in the indirect pathway from the putamen
(b) type II spiny neurons: contain GABA, substance P, and dynorphin, and express D1 receptors; act in the direct pathway from the putamen
ii. aspiny neurons: the interneurons of the striatum
(1) subtypes
(a) type I aspiny neurons: contain GABA
(b) type II (large) aspiny neurons: contain acetylcholine
(c) type III aspiny neurons: contain somatostatin and neuropeptide Y
Figure 1–10 Superficial view of the diencephalic roof. (From Kahle W, Color Atlas/Text of Human Anatomy, Vol. 3: Nervous System and Sensory Organs. 4th ed. Stuttgart, Germany: Georg Thieme; 1993:207, Fig. B. Reprinted by permission.)
b. effects of lesions
i. caudate lesions cause contralateral choreoathetosis, abulia, and behavioral disinhibition
ii. putamen lesions cause
(1) contralateral hemidystonia (70%) > hemichorea or hemiparkinsonism
(2) hypophonic dysarthria with a transcortical motor aphasia-like language impairment due to bradykinesia
(3) impairment of short-term memory
(4) contralateral tilting and falling (in lesions that involve the globus pallidus)
2. Globus pallidus: contains large motor-type neurons and interneurons
a. unilateral lesions cause contralateral hemidystonia and/or hemiparkinsonism, abulia, and short-term memory loss; bilateral lesions may also cause akinetic mutism
3. Substantia nigra: lesions produce contralateral parkinsonism; subdivisions include
a. pars compacta (dopaminergic): has a predominantly afferent function in the basal ganglia loops; projects to the striatum via the nigrostriatal dopaminergic pathway
b. pars reticulata (GABAergic): has a predominantly efferent function in the basal ganglia loops; projects to the ventral anterior and ventral lateral nuclei of the thalamus
4. Ventral tegmental area: projects to the nucleus accumbens via the mesolimbic dopaminergic pathway; analogous to the substantia nigra for the limbic striatum
5. Subthalamic nucleus
a. lesions cause hypotonia and continuous flailing movements of the extremities sparing the face due to uncontrollable contractions of the proximal musculature {ballismus}
i. ballismus typically involves the contralateral half of the body {hemiballismus}, and may involve only a single extremity {monoballismus}
ii. ballismus is a violent type of chorea, and it may resolve over time into chorea
iii. ballismus may occur with lesions elsewhere in the basal ganglia, thalamus, or motor cortex, although these forms are generally milder than that caused by subthalamic nucleus lesions
Figure 1–11 Coronal section of the thalamus at the level of the mammillary bodies. (From Kahle W, Color Atlas/Text of Human Anatomy, Vol. 3: Nervous System and Sensory Organs. 4th ed. Stuttgart, Germany: Georg Thieme; 1993:163, Fig. B. Reprinted by permission.)
(1) transient dysfunction of the basal ganglia may underlie a rapidly reversible form of hemiballismus that occurs during hyperglycemia
iv. treatment: antipsychotic medications that have antagonist properties on D2 receptors (i.e., not clozapine); stereotactic lesioning or deep brain stimulation of the internal globus pallidus or ventral anterior–ventral lateral thalamus
6. General circuitry: See p. 12 (Table 1–2)
a. output projections (GABAergic) from the globus pallidus and substantia nigra reticulata to the (Box 1.6)
Box 1.6
Ansa lenticularis also carries ascending cerebellar projections to ventral lateral thalamic nucleus.
i. thalamus via ansa lenticularis and the lenticular and thalamic fascicles
ii. subthalamic nucleus
V. Thalamus (Fig. 1–11)
1. Functional divisions (Fig. 1–12)
a. cortical relay nuclei
i. anterior nuclei group (AG): functions in memory processing, learning, and attention
(1) subcortical afferents: hippocampus and mammillary bodies via mammillothalamic tract (part of the circuit of Papez)
(2) bilateral lesions cause
(a) anterograde amnesia, when they occur in conjunction with bilateral lesions of the dorsomedial thalamic nuclei
(b) antero- and retrograde amnesia {Korsakoff’s syndrome}, when they occur in conjunction with bilateral mammillary body and mammillothalamic tract lesions
ii. ventral nuclei group (Box 1.7) (Box 1.8)
Box 1.7
Subcortical Aphasias
Caused by dominant-side lesions of the anterior limb of internal capsule + head of caudate nucleus and/or putamen
Thalamic nuclei: dorsomedial nucleus or ventral nuclei group, which usually produces a mixed transcortical aphasia sparing reading and writing.
Box 1.8
Ventral anterior nuclei and ventral lateral nuclei are the motor nuclei of ventral nuclei group.
(1) ventral anterior nuclei
(2) ventral lateral nuclei: afferents involve not only globus pallidus and substantia nigra reticulata, but cerebellum as well
(a) lesions cause contralateral
Figure 1–12 Functional divisions of the thalamus. (From Duus P, Topical Diagnosis in Neurology. Stuttgart, Germany: Georg Thieme; 1998:275, Fig. 8.22. Reprinted by permission.)
(i) hemiataxia, usually with hemiparesis and/or sensory loss from involvement of nearby structures; develops acutely after injury
(ii) intention tremor: develops several weeks after injury
(iii) dystonia: develops several months or years after injury
(3) lateral geniculate (see p. 14) (Box 1.9)
Box 1.9
Lateral geniculate, medial geniculate, and posterior lateral nuclei: sensory nuclei of the ventral nuclei group
(4) medial geniculate
(5) ventral posterior nuclei
(a) ventral posterolateral nucleus has distinct areas for proprioception (i.e., the shell of the nucleus) and cutaneous (i.e., the core) inputs from body
(b) ventral posteromedial nucleus receives trigeminal inputs for facial sensation and inputs from the solitary tract nucleus via the solitary tract conveying taste
(c) lesions cause
(i) chiro-oral syndrome—symptoms include the contralateral loss of all sensory modalities and paresthesias of the mouth, tongue, and hand
1. face, trunk, and proximal extremities are bilaterally represented (with a contralateral preference) in the ventral posterior thalamus, and so their sensation is preserved in unilateral lesions
(iii) Dejerine-Roussy syndrome/anesthesia dolorosa (Box 1.10)
1. generally develops weeks or months after the initial lesion; occasionally develops acutely after injury
2. symptoms: burning pain that may be continuous or triggered by innocuous stimuli, and that is aggravated by emotional stress; sensory loss (proprioception > other modalities; deep sensation > cutaneous sensation)
a. threshold for pain is paradoxically increased in the affected regions
3. treatment: thalamotomy of the intralaminar thalamic nuclei
b. association nuclei
i. dorsomedial nucleus (Box 1.11)
Box 1.11
The dorsomedial nucleus is injured during Wernicke–Korsakoff syndrome even more than the mammillary bodies.
(1) specific afferents are from limbic structures (ventral basal ganglia, amygdala, hippocampal formation) and hypothalamus
(2) bilateral lesions cause
(a) hypersomnolence with reduced non-REM sleep
(b) akinetic mutism, coma
(c) anterograde amnesia, when they occur in conjunction with bilateral anterior thalamic lesions
(d) Klein-Levin syndrome—symptoms include recurrent episodes lasting several days or weeks of compulsive eating, sexual disinhibition, hypersomnia with normal sleep structure, and impaired memory
(i) likely involves some simultaneous dysfunction of the posterior hypothalamus
(ii) more common in adolescent boys, and spontaneously remits in adulthood
(iii) treatment: lithium, valproate
ii. pulvinar has poorly defined visual and language functions
c. intralaminar thalamic nuclei (ITN): connections involve
i. rostral nuclei: reticular formation → ITNr → entire telencephalon and diencephalon
ii. caudal nuclei: reticular formation → ITNc → motor telencephalon and diencephalon
d. reticular thalamic nucleus (RTN): forms a thin shell on the lateral surface of the thalamus
i. connections: reticular formation, collaterals of thalamocortical projections (particularly from intralaminar thalamus group), and cortex → RTN → all thalamic nuclei and reticular formation
2. Chemoanatomy: glutamate and aspartate are released from all thalamic nuclei except the reticular thalamic nucleus, which is GABAergic
3. Vascular anatomy (Fig. 1–13)
Figure 1–13 Vascular anatomy of the thalamus. (From Duus P, Topical Diagnosis in Neurology. Stuttgart, Germany: Georg Thieme; 1998:188, Fig. 5.8. Reprinted by permission.)
Figure 1–14 The hypothalamus in sagittal (A) and coronal (B) views. Panel A shows only the medial hypothalamus. Arrows in panel A correspond to the cross-sections in panel B. (From Duus P, Topical Diagnosis in Neurology. Stuttgart, Germany: Georg Thieme; 1998:194, Fig. 5.13; 195, Fig. 5.14. Reprinted by permission.)
VI. Hypothalamus
1. General functions
a. controls the autonomic nervous system and the endocrine system (Box 1.12)
Box 1.12
Autonomic Effects of Stimulation
Parasympathetic effects: Preoptic region (medial hypothalamus), supraoptic region (medial hypothalamus)
Sympathetic effects: Mammillary region (medial hypothalamus), lateral hypothalamus
b. maintains visceral activity, metabolic activity, and body temperature
c. participates in goal-directed behavior and motivation, affective behavior (particularly aggressive behaviors), and sleep–wake cycling
2. Subdivisions (Fig. 1–14)
a. lateral hypothalamus: a “reward” center that likely mediates pleasurable sensations; regulates thirst (by means of vasopressin secretion from the paraventricular and supraoptic nuclei of the supraoptic region) and hunger and food-motivated behaviors (Table 1–3)
b. medial hypothalamus: divided into
i. preoptic region
(1) median preoptic nucleus: a sexually dimorphic nucleus
(2) medial preoptic nucleus: regulates body temperature and febrile responses (Box 1.13)
Box 1.13
Responses involved in body temperature regulation include:
Heat loss (preoptic region): vasodilation; sweating, panting; decreased metabolism
Heat production (posterior nucleus): vasoconstriction; shivering, piloerection; increased metabolism
(3) lateral preoptic nucleus: promotes sleep onset, and inhibits brain regions that are necessary for maintaining arousal by GABAergic and galanin projections
ii. supraoptic region
(1) neurohormonal/magnocellular nuclei (paraventricular and supraoptic nuclei): project to the posterior pituitary where they directly release hormones
(2) suprachiasmatic nucleus: the pacemaker of circadian rhythms (e.g., sleep–wake cycle, body temperature, cortisol and growth hormone release)
(a) receives direct input from the retina; projects predominantly to the preoptic region and neurohormonal nuclei
(b) lesions cause irregular timing of sleep–wake behaviors; otherwise a normal amount of time is spent in each sleep phase
iii. tuberal region
(1) ventromedial nucleus: inhibits feeding behaviors together with the arcuate nucleus; opposed by the lateral hypothalamus (Box 1.14)
Box 1.14
Froehlich’s Syndrome
Symptoms—Obesity, failure of sexual development, and polyuria, occurring mostly in boys
Cause—Hypothalamic lesions producing multiple releasing hormone deficiencies
Differential diagnosis—Prader–Willi syndrome
(2) hormonal/parvocellular nuclei, which includes the ventromedial and arcuate nuclei: project to the median eminence where they release hormones into the portal system that then influence anterior pituitary hormone release (Table 1–4)
(3) dorsomedial nucleus, tuberal nucleus
iv. mammillary region
(1) mammillary bodies: receives projections from the hippocampus via the postcommissural fornix
(a) serves in the circuit of Papez for memory formation; one of the chief sites of atrophy in Wernicke–Korsakoff syndrome
(2) posterior nucleus: a “punishment” center that likely mediates negative affect; also involved in
(a) inducing and maintaining wakefulness by diffuse histamine projections
(b) initiating rage and fighting behaviors
(c) regulation of the sympathetic nervous system
3. Connections (Fig. 1–15)
4. Vascular supply
a. anterior hypothalamus: recurrent artery of Huebner
b. middle hypothalamus: thalamotuberal branches from posterior communicating artery
c. posterior hypothalamus: thalamoperfo-rating branches of posterior cerebral artery
VII. Cerebellum
1. Types of cerebellar neurons (Fig. 1–16)
a. molecular layer
i. Purkinje neurons: have a flat dendritic field that is organized perpendicular to the long axis of folia
(1) receive excitatory inputs from granular cells, which send parallel fibers that pass perpendicularly through the dendritic field of the Purkinje neurons
(2) send inhibitory GABAergic projections to the deep cerebellar nuclei and directly to the lateral vestibular nucleus
ii. interneurons (stellate cells, basket cells): all are GABAergic and inhibitory on the Purkinje neurons; all are excited by parallel fibers from the granular cells
iii. Bergman glia: form shells around the Purkinje cell bodies, exposing only synaptic sites; also serve to guide granule cell migration during development
b. granular layer
i. granular cells: each granular cell receives input from a single mossy fiber in a multisynaptic complex {glomerulus} that also involves inhibitory Golgi neuron inputs
ii. Golgi neurons: receive input from parallel fibers, but unlike Purkinje neurons they exhibit a three-dimensional dendritic field that extends into the molecular layer
(1) send inhibitory projections to the glomeruli
2. General afferent fibers
a. cerebellar relay nuclei
i. inferior olivary complex: sends glutamatergic projections to Purkinje neurons and Golgi cell dendrites {climbing fibers} (Box 1.15)
ii. pontine nuclei and arcuate nuclei (ectopic pontine nuclei), which in turn receive projections from the motor cortex {corticopontine tract}
Figure 1–15 Afferents (A) and efferents (B) of the hypothalamus. (Refer to Figure 1–14 for nuclei labels.) (From Duus P, Topical Diagnosis in Neurology. Stuttgart, Germany: Georg Thieme; 1998:196, Figs. 5.15, 5.16. Reprinted by permission.)
Box 1.15
Pontine nuclei arcuate nuclei, and all sensory inputs form mossy fiber inputs (glutamate) to the granular layer glomeruli
Figure 1–16 Types of cerebellar neurons and basic connections of the cerebellum. (From Duus P, Topical Diagnosis in Neurology. Stuttgart, Germany: Georg Thieme; 1998:166, Fig. 4.3. Reprinted by permission.)
i. spinal cord via spinocerebellar tracts
ii. medial and inferior vestibular nuclei
iii. vestibular division of CN VIII directly
iv. tactile, auditory, and visual inputs by poorly defined routes
c. modulatory inputs: pedunculopontine tegmental nucleus (acetylcholine); locus coeruleus (norepinephrine); ventral tegmental area (dopamine); medullary raphe nuclei (serotonin)
3. Efferents from the deep cerebellar nuclei: outputs are glutamatergic except for some of the projection to the inferior olivary nucleus, which is GABAergic
a. fastigial nucleus: contralateral > ipsilateral projections to the vestibular nuclei and pontomedullary reticular formation {uncinate fasciculus} via the inferior cerebellar peduncle (juxtarestiform body)
b. interposed nuclei (globose, emboliform): contralateral projections to the red nucleus via the superior cerebellar peduncle
c. dentate nucleus: contralateral projections via the superior cerebellar peduncle to the thalamus (ventro-lateral nuclei and a small part of the ventropostero-lateral nucleus), the ocular motor and accessory ocular motor nuclei, and the pontomedullary reticular formation
4.
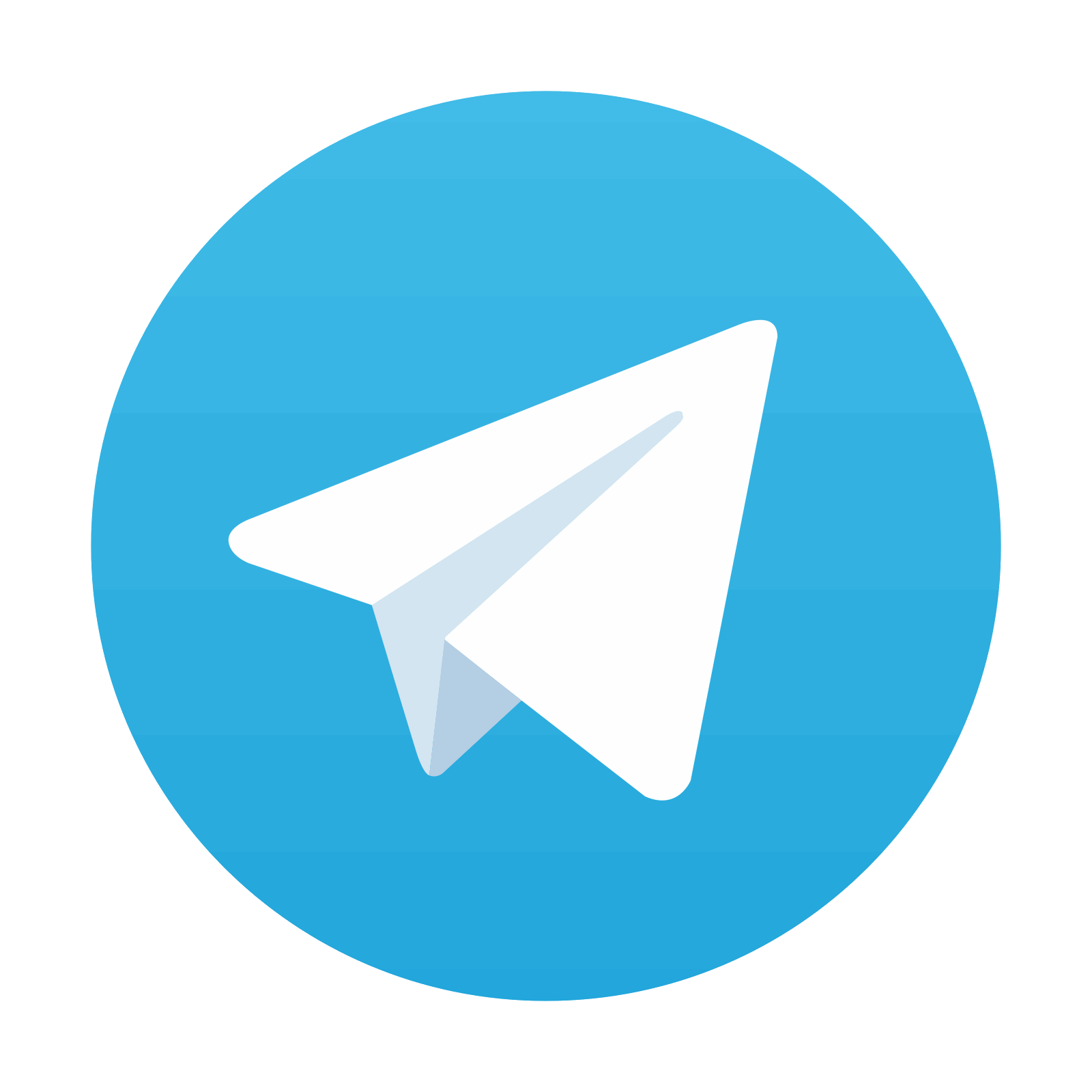
Stay updated, free articles. Join our Telegram channel

Full access? Get Clinical Tree
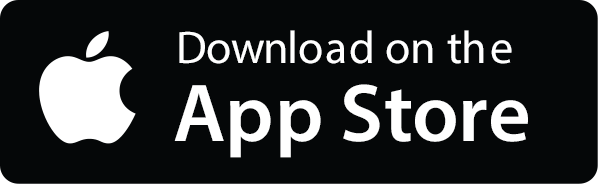
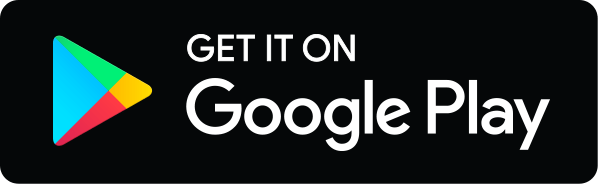