html xmlns=”http://www.w3.org/1999/xhtml” xmlns:mml=”http://www.w3.org/1998/Math/MathML” xmlns:epub=”http://www.idpf.org/2007/ops”>
Cognition is defined as the mental mechanisms through which thought, experience, and sensory organs operate to acquire knowledge and understanding; “cognition” as an umbrella term has extended to include active processes, such as judging and problem-solving, imagination, as well as planning. The foregoing processes are emergent properties of the brain, and the brain’s anatomy must contribute to how cognition works as well as how it can go awry. Cajal’s original images of how brain cells were organized suggested that the brain is structured in such a way that its function can be likened to a mighty parallel processor. Its sub division into structurally disparate areas – architectonics – was followed by functional localization through brain lesion and stimulation experiments. Similarly, as technology continues to advance, we are able to identify more in-depth brain function either at nodes, within distributed networks, and/or at chemically specific addresses.
The overarching theme of this book is that cognition is central to the pathophysiology of major depressive disorder (MDD). All domains of cognitive function have been described as abnormal in MDD, particularly when patients are acutely depressed (Chapter 3). Hot cognition is disturbed because emotional bias at an unconscious, but also most obviously at a conscious, level is clearly negative, and changes (for the positive) on recovery (Chapter 7). Cold cognition is increasingly being recognized in MDD; however, the effects of MDD on cognition and vice versa have yet to be elucidated. The pattern of deficits is similar in younger and older groups of individuals with MDD, although memory and learning are notably affected in those over 60 years of age (Thomas et al., 2009). The functional neuroanatomy of complex cold cognitive tasks is not highly localized, but the subservient anatomical networks involve nodes in the frontal cortex and hippocampus. Hot cognition (Chapter 6) is supported by pathways more often associated with the mesial frontal lobes, amygdala, thalamus, and basal ganglia. Taken together, the candidate brain anatomy is roughly outlined by the functional impairments commonly observed in patients with MDD, but it is not much constrained thereby.
Modern neuroscience continues, nevertheless, to be preoccupied by brain anatomy because it is so fundamentally an expression of brain function. The implication that can be derived from the preamble above is that the anatomy of the brain will also be central to explaining what MDD is. This is a surprising conclusion, and it would be a pointless one if we were not increasingly able to visualize the relevant anatomy in humans and start to build hypotheses upon it. Most of the existing studies have been conducted using non-invasive imaging: X-ray computed tomography originally, but now almost universally magnetic resonance imaging (MRI). Proton MRI measures the properties of water dipoles in the brain and can tell us something about the density of brain tissue, its structure as expressed in water diffusion, and regional blood flow reflected by oxygen desaturation in blood. Brain anatomy in MDD is studied primarily using the first of these applications. Thus, diffusion-tensor imaging (DTI) has contributed significantly to detecting white matter abnormality in MDD (Chapter 14) and blood oxygen level detection (BOLD) is widely used to study localization of brain function. The design of most studies is cross-sectional comparison of cases and controls. Where there are differences, it is reasonable to ask for validating clinical measures that correlate with the brain abnormality within the clinical group. Quantitative measures of cognition in particular could be very useful for this purpose and will be highlighted where they exist. However, because deficits of cold cognition have so often been ignored, it is quite unusual for the data to be available.
By contrast to the relatively large literature on imaging, classical neuropathological approaches to major depression, in common with all psychiatric disorders, have been limited and often little emphasized. Obviously they start from post-mortem tissue, and with a perhaps understandable pessimism about what is likely to be found in such a relatively subtle disorder. However, this approach still offers the only way we currently have for direct access to cellular abnormality in depressed brains. The limitations are widely understood and relate to the agonal state of the brain at death, the status of the psychiatric diagnosis (and comorbidity), the effects of treatment, and the impact of aging. However, some consistent findings on brain cellular anatomy are described and are of considerable interest. This is notwithstanding the fact that the primary interest of post-mortem studies has often been brain biochemistry, which is beyond the scope of this chapter.
Cellular anatomy of the brain in MDD
In reviewing the field over a decade ago, Harrison (2002: 1428) commented:
all findings remain preliminary due to a lack of unequivocal replication and the failure to control fully for other potential confounders and co‐morbid conditions. There are also basic questions to be answered concerning the clinical correlates, magnitude, progression and heterogeneity of the pathology. Nevertheless, it must now be considered likely that changes in brain structure, both macroscopic and microscopic, are a feature of primary mood disorder, a fact to be taken into account when interpreting functional imaging, neuropsychological and neurochemical data.
There remains cautious but still a reasonable consensus that MDD is associated with reduced post-mortem detection of glial cells (Rajkowska & Stockmeier, 2013). In contrast, counts of neurons suggest broad preservation of cell number. If correct, this simple finding must have profound implications for how we should think about the cellular underpinnings of cognition in MDD. There are three kinds of glial cells in the central nervous system: astrocytes (the most numerous and multifunctional), oligodendrocytes, and microglia. Astrocytes outnumber neurons several fold and come in two major subtypes: protoplasmic astrocytes in gray matter and fibrous astrocytes in white matter. Astrocytes are believed to be critical to the microenvironment of the brain by regulating glucose metabolism, neurotransmitter uptake (particularly glutamate), synaptic development and maturation, and the blood–brain barrier. Informative post-mortem studies have either counted cell density (using reliable methods) or localized cell types in brain tissue by antibody staining. The markers of astrocytes include glial fibrillary acidic protein (GFAP), gap junctions proteins such as connexin 40 and 43, the water channel aquaporin-4 (AQP4), a calcium-binding protein S100B, the excitatory amino acid transporters 1 and 2 (EAAT1, EAAT2), and glutamine synthetase. Human post-mortem studies have demonstrated changes in each of these astrocytic markers in MDD. In both gray (GM) and white matter (WM) there is a reduced density and function of astrocytes. This finding seems to be most striking in frontal/limbic cortex and associated subcortical areas but this may in part reflect reporting bias. The extent to which these findings are unique to depression (and not seen in other functional psychiatric disorders) remains debatable. Indeed, the finding of comparable non-specific global cognitive impairments in bipolar disorder and schizophrenia may suggest common findings are quite likely.
The implication of reduced numbers and density of astrocytes is likely to be highly significant for gross brain anatomy. In particular, this seems probable for changes that are essentially in brain density or isotropy as estimated using non-invasive brain imaging of all kinds. Indeed it is tempting to assume that the findings from human imaging can be extrapolated back to cellular brain structure, as “atrophy,” reduced brain density, WM disorganization, etc. The caution has to be that post-mortem studies are conducted on very limited sample numbers and on tissue subjected to all the complications of relatively prolonged tissue death and post-mortem delay. The necessary correlations between in vivo and post-mortem findings do not exist. Nevertheless glial cells will have a major capacity to influence signals from MRI. The excess of glia over neurons in GM has been noticed already. In the case of WM, axons are obviously present in massive numbers, but glial cell processes may cover a disproportionately large area, amounting to almost 50 percent of a voxel (oligodendrocytes are the most abundant glial cells and astrocytes are second most abundant in WM) (Walhovd, Johansen-Berg, & Karadottir, 2014).
The central interest of glial cells for depression highlights the absence of simple measures of their function in humans. Unlike neurons, glial cells do not communicate via electrical action potentials, but they may well contribute to functions requiring spatial and temporal integration (and typical of cognition). A recent editorial has emphasized how much neuroscience needs to shift attention toward glial cells to solve contemporary mysteries in cognition (Fields et al., 2014). The convergence between the cellular story in MDD and the parallel discovery of cognition as central to MDD is rather striking. Indeed, we should perhaps be thinking of MDD as a glial dysfunction before we look anywhere else. The fact that astrocytes can be produced from induced pluripotent stem cells means that the properties of disease- relevant cells from individual MDD patients may soon become available for investigation in vitro (Onorati et al., 2010).
Brain anatomy in MDD
In recent years a relatively large number of structural MRI studies have used voxel-based morphometry (VBM) to look at differences in GM structures. Unfortunately, there is a general problem with imaging studies in psychiatry that they have tended to be too small and so adapted to detect effect sizes that are larger than may actually be plausible (Kempton, Geddes, Ettinger, Williams, & Grasby, 2008). In addition, controls are often poorly selected: cases and controls should be recruited using very similar methods, which is always difficult in clinical case series. Together with the data-driven approaches to image analysis encouraged by available statistical packages and the usual publication biases, this has predisposed to the publication of positive findings that are not always exactly replicated. While a common problem in biological science, it means that for confidence, pooled analyses of data from as many comparable studies as possible are generally preferable to single studies, and the policy will be to describe findings from pooled analyses here.
In addition, MDD is a heterogeneous condition in which the uncertain cumulative effects of physical illness and poor lifestyle choices may produce acquired brain changes in mature patients. Moreover, current or previous drug treatment can be a major confound of imaging studies in MDD. While it is uncertain whether drug treatment per se would have an impact on brain structure, animal studies suggest that it might. Nevertheless, and these qualifications accepted, recent analysis of published data from 14 data sets (400 medication-free MDD patients and 424 healthy controls) has demonstrated consistency. It confirmed reduced GM in prefrontal and limbic cortex (including hippocampus bilaterally) (Zhao et al., 2014).
A pooled analysis from a selection of multimodal studies also supported reduced GM in frontal and limbic cortex (of the peak coordinates for MR structural data); specifically there was reduced GM in amygdala, dorsal fronto-median cortex, and right para cingulate cortex. In the same studies, positron emission tomography demonstrated increases in glucose metabolism in right subgenual and pregenual anterior cingulate (Sacher et al., 2012). The connectivity of the subgenual region had previously been shown to suggest projections to nucleus accumbens, amygdala, hypothalamus, and orbitofrontal cortex (Johansen-Berg et al., 2008).
To give some idea of the effect size for a familiar structure, a meta-analysis of volumes measured for the hippocampus gave a standardized mean difference (SMD) of −0.41, 95% confidence interval: [−0.78; −0.03], z = −2.14, p = 0.0321 for left and −0.53[−0.98; −0.09], z = −2.38, p = 0.0173) for right hippocampi. The average volume reduction relative to healthy controls was −4.0 percent in the left and −4.5 percent in the right hippocampus. This is a moderately large effect in studies selectively of first-episode cases. However, the patients were not very young (mean approximately 37 years) and illness duration was rather long (mean 14 months) (Cole, Costafreda, McGuffin, & Fu, 2011).
Other relatively well-powered and controlled studies have not confirmed brain volume changes in first-episode MDD and instead suggest that reductions in hippocampal volume (and so other GM changes) relate to illness duration (Cheng et al., 2010).
Adequate studies of at-risk subjects have not been published. Currently, therefore, it seems a reasonable hypothesis that brain structural changes in GM in MDD are acquired as a function of illness duration, intensity, or recurrence. It is a further hypothesis that hypercortisolemia or other aspects of the biology of MDD contribute to this change in the brain. The underlying functional neuropathology may in turn be reduced astrocyte numbers in frontal areas.
There are few studies that adequately combine measures of structure and cognition to make the further link between brain structure and cognition.
Brain anatomy and drug treatment in MDD
Building on the relatively robust and simple finding of reduced hippocampal volume in MDD, it has been suggested that small volumes are associated with depression severity, age at onset, non-responsiveness to treatment, untreated days of illness, illness burden, history of childhood abuse, level of anxiety, and certain genetic polymorphisms. Cross-sectional correlations can only suggest not prove causation, of course. For example, in chronic treatment-resistant depressed patients, we described reduced GM density in the left temporal cortex, including the hippocampus, which correlated with measures of verbal memory (and supported the functional significance of the observed MRI changes) (Shah, Ebmeier, Glabus, & Goodwin, 1998).
A few small prospective cross-sectional studies have examined whether hippocampus volume is prospectively associated with response to treatment in patients with depression, and generally, the larger the hippocampal volume, the greater the likelihood of response. Hippocampal volume therefore has some theoretical potential as a biomarker to predict response (MacQueen, 2009).
Whether response to treatment involves change in brain size, for example in frontal cortex, has been suggested in small, less than conclusive studies. This raises further issues of contemporary interest, such as does any change in brain size map onto cognitive improvement, rather than reduction in non-cognitive depressive symptoms? To answer this question would require large systematic treatment studies with parallel measures of brain size/function and cognition. Large size would be required to model direct and indirect pathways of treatment effect, and imaging reliably across many sites would bring its own challenges.
White matter integrity in MDD
Reports of WM abnormalities in mood disorder patients date from the early days of CT and MR imaging. So-called WM hyperintensities are frequently attributed to vascular pathology (Brown, Lewine, Hudgins, & Risch, 1992) and are most noticeable in older patients (Dupont et al., 1995) (Chapter 14). They are not believed to be a highly specific accompaniment of MDD (Sexton et al., 2012a), but are correlated with vascular disease.
Difffusion-weighted MR imaging (DTI) has been used in a limited (but now increasing) way to determine the integrity of WM in MDD. By resolving the diffusibility of water in different directions it gives a measure called fractional anisotropy (FA). Very constrained diffusion, as for example along the axons of a WM tract would have a high value for FA. Unconstrained diffusion as in a simple solution of saline or cerebrospinal fluid would have zero FA. Thus, reduced FA in cases compared with controls may imply WM disorganization either as a developmental or an acquired property of the MDD brain.
As in the case of studies of GM, consistency has been lacking across DTI studies. Variation in findings across studies is potentially due to the confounding factors already described for GM studies above. In an early systematic review, 21 of 27 studies described reduced FA in subjects with affective disorders compared with controls. Tracts in frontal and temporal lobes (and especially superior frontal gyrus) were most implicated but formal heterogeneity and evidence of publication bias were also significant (Sexton, Mackay, & Ebmeier, 2009). Subsequent reviews have reached the same conclusion and shown rather widespread WM changes, not just confined to frontal areas (Liao et al., 2013).
White matter integrity and neurodevelopment
In MDD, a small first-episode study suggested patients with MDD showed decreased FA values in three WM tracts: the left anterior limb of the internal capsule, the right parahippocampal gyrus, and the left posterior cingulate cortex (Zhu et al., 2011). No regions showed higher FA in MDD patients than in controls. Rather similar findings in young people with hypomanic experience showed diffuse reduction in FA (Yip, Chandler, Rogers, Mackay, & Goodwin, 2013a). Such findings in young people may suggest decreased fiber coherence or glial distribution (Song et al., 2002) as a risk factor in affective disorder. The abnormality appears likely to be neurodevelopmental rather than acquired as a result of medication or illness course. FA increases during healthy adolescence at different rates across different brain structures; for example, the splenium stabilizes by age 15 years whereas the uncinate fasciculus is probably still changing at age 30 (Lebel, Walker, Leemans, Phillips, & Beaulieu, 2008). This implies that development would be complete in the splenium in 20-year-olds, and so any difference in FA at that age would be enduring. This finding complements and may partly explain the findings of reduced FA in mature patients. Data from healthy twins strongly suggest that FA is under genetic control (Brouwer et al., 2012).
Accordingly, diffuse FA abnormalities in WM may be a neurodevelopmental marker of vulnerability to affective disorder. A small study of at-risk adolescents with a family history of mood disorder showed the predicted effect (Huang, Fan, Williamson, & Rao, 2011) and the clinical spectrum may include generalized anxiety disorder (Korgaonkor et al., 2011; Hettema et al., 2012). Other preliminary studies in MDD suggest that treatment resistance may be associated with lower FA (Zhou et al., 2011) and resilience to MDD may be associated with higher FA measures (Frodl et al., 2012). Future studies will be required to determine whether FA is a useful biomarker for stratification in clinical trials.
Alterations in WM microstructure have been implicated in a range of other disorders including bipolar disorder, attention-deficit/hyperactivity disorder (van Ewijk, Heslenfeld, Zwiers, Buitelaar, & Oosterlaan, 2012), schizophrenia (Ellison-Wright & Bullmore, 2009), and impulse control disorders (Yip et al., 2013b). Thus, alterations in WM microstructure may be a rather general marker of developmental abnormality with the potential to form a substrate for behavioral pathology. WM investigation at higher field and using more advanced MR methods may yet yield the higher resolution studies required to clarify any specificity by diagnosis that may exist.
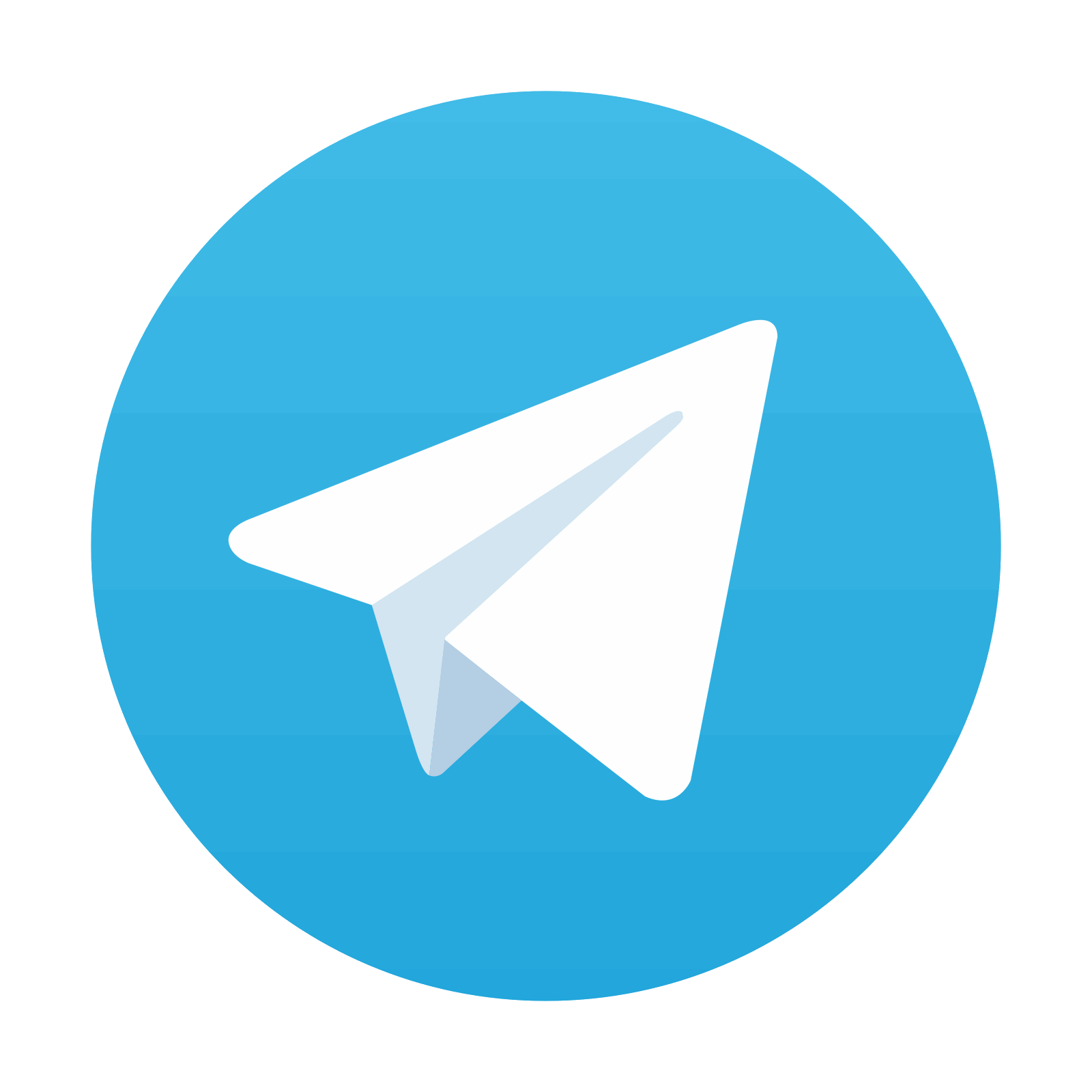
Stay updated, free articles. Join our Telegram channel

Full access? Get Clinical Tree
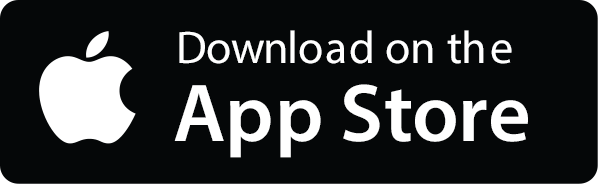
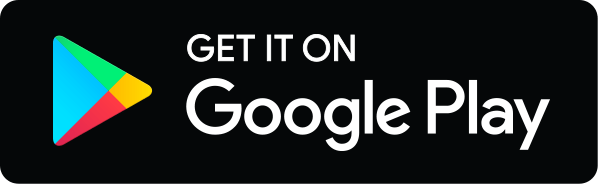