Fig. 1
Schematic representation of the Mecp2 gene and localization of the modifications made to engineer Mecp2-deficient mouse models. See text for details
Hundreds of MECP2 mutations are known in humans. Mutations were initially described in females with the typical RTT phenotype [5]. Soon after, males with MECP2 mutations were also described with an even more severe phenotype, in good agreement with the classical X-linked dominant inheritance pattern of MECP2 mutations. In the case of males, as for females, mutations are not inherited from the parents and occur sporadically. These mutations leading to RTT in females and neonatal encephalopathy in males constitute a first group (Group I mutations). A second group of mutations, never found in females with the classical RTT phenotype, cause X-linked intellectual disability in males and females (Group II mutations). The clinical consequences of group II mutations are generally milder (although still severe) than the classical RTT phenotype or male neonatal encephalopathy. Finally, deletions and duplications of the whole MECP2 gene have been described. Deletions cause a classical RTT phenotype in females and neonatal encephalopathy in affected males [8]. Intriguingly, duplications of MECP2 have been identified in male patients suffering from severe intellectual disabilities [9]. In this latter case, most female carriers are asymptomatic due to favorably skewed X-chromosome inactivation. Hence, it looks as if Group I mutations and deletions are behaving differently (with random X-chromosome inactivation and affected females) than MECP2 duplications (with asymptomatic carrier females). This large phenotypic spectrum suggests that (1) there are at least two categories of mutations causing different clinical phenotypes (Table 1); (2) the dose of MECP2 protein needs to be tightly controlled because both deletions and duplications cause a severe neurological phenotype; and (3) female cells have a different behavior when MECP2 is lacking or when it is overexpressed. These points will be important to consider to understand the significance of the results obtained using mouse models of Mecp2 dysfunction (see below). The Australian MECP2 mutation database lists more than 4,000 disease-causing mutations (http://mecp2.chw.edu.au/). Seventy-five percent of the mutations in MECP2 are predicted to disrupt the sequence of the protein and to cause either the absence of protein or the production of a putative truncated peptide.
Table 1
Phenotypes associated with MECP2 mutations in most patients
Female phenotype | Male phenotype | |
---|---|---|
Group I mutation | Rett syndrome | Neonatal encephalopathy |
Group II mutation | XLID, mild to moderate | XLID, moderate to severe |
Deletion | Rett syndrome | Neonatal encephalopathy |
Duplicationa | Unaffected carrier | XLID, severe |
2 Rett Syndrome Mouse Models
The mouse and human MECP2 genes are highly conserved and both located on the X chromosome. The MECP2 proteins display 94 % identities and 96 % similarities at the amino acid level. In both species, the gene is composed of four exons and is ubiquitously expressed; exon 2 is alternatively spliced and the isoform containing exons 1-3-4 is the predominant isoform in the brain (Mecp2-e1). These evolutionary conservation data suggest that modeling RTT in the mouse is relevant and feasible. However, because the mouse will not reproduce specific phenotypic traits such as language loss or hand stereotypies (two major clinical criteria that are necessary to make the diagnosis of RTT), we will talk of “models of Mecp2 deficiency” rather than “models of Rett syndrome” from now on in this chapter.
Given that most patients with RTT have a mutation disrupting the sequence of the MECP2 protein (although it is not always known if a modified protein is produced or not), the first mouse models that were engineered were knockouts. Several knock-in mouse models were subsequently engineered to reproduce mutations frequently found in RTT (Group I mutations), or in individuals presenting milder phenotypes (Group II mutations), or to model the overexpression of MECP2 (Table 2). These four classes of models will be discussed in the following sections: knockouts and conditional deletions in section A, knock-ins in section B, and models allowing the alteration of the Mecp2 protein dosage in section C.
Table 2
Major mouse models engineered to study the phenotypes associated with MECP2 dysfunction
Model name | Model class | References | |
---|---|---|---|
Group I mutation | Mecp2-308 | Knock-in | [36] |
R168X | Knock-in | [70] | |
R270X | Knock-in | [75] | |
T158A | Knock-in | [77] | |
R306C | Knock-in | [80] | |
Group II mutation | A140V | Knock-in | [68] |
G273X | Knock-in | [75] | |
S421A | Knock-in | [78] | |
T308A | Knock-in | [79] | |
Deletions | KO-B | Knock-out | [12] |
KO-J | Knock-out | [14] | |
TAM | Knock-out | [17] | |
Duplication | MECP2Tg1 | Transgenic | [62] |
Other | Tau-Mecp2 | Transgenic | [53] |
Mecp2 Lox-Stop | Knock-in | [32] | |
I-Mecp2 | Transgenic | [59] |
Frequent (>3 %) truncating group I mutations include R168X, R270X, R255X and R294X [10]. For group II mutations, the A140V model is relevant to human pathology because it reproduces a mutation that is frequently found in patients with mild to moderate X-linked intellectual disability [11]. To model dose-dependent phenotypes, the MECP2Tg1 mouse was created by the insertion of a PAC clone containing the human MECP2 locus into the mouse genome to increase the amount of MECP2 protein in the mouse cells. Hypomorphic alleles are also available and will be discussed in section B. Finally, conditional knockout Mecp2 floxed mice were produced and crossed with a series of Cre-expressing lines to delete Mecp2 from specific cell populations. These models are discussed in section A below, together with constitutional knockouts.
2.1 Constitutive and Conditional Knockout Models
2.1.1 Mecp2 KO-B
The first mouse model of Mecp2 deficiency has been generated by the laboratory of Adrian Bird [12]. This model has been created crossing mice carrying a floxed copy of MeCP2 with a mouse that expresses CRE recombinase on the X chromosome. The resulting mouse (Mecp2tm1.1Bird Mecp2Bird) is deleted for exons 3 and 4 of MeCP2, respectively, encoding the methyl-binding and the transcriptional repression domains. No MeCP2 protein is detected in the mice using antibodies targeting epitopes located in the N- or C-terminus [12]. This mouse model mimics more or less the development of the RTT phenotype. The development seems unaffected during early intrauterine and postnatal periods. The KO males for MeCP2 (MeCP2−/y) are most severely affected. The first signs are visible from the third week of postnatal life and worsen during the entire life of the animal to its death occurring at 7–8 weeks. Affected mice present first a growth deficit (reduced size) and abnormal positioning of the hind limbs (hind limb clasping). At the motor level, the mice exhibit a loss of coordination associated to the reduction in the number of spontaneous movements. Breathing instability is also observed mainly characterized by hyperventilation followed by apneas [13]. It is interesting to note an irregular tooth wear, possibly due to a defect of positioning between the maxillary and mandible bones. In contrast to males, no obvious symptoms are observed in heterozygous females (Mecp2+/−) during early postnatal life. Studies of Mecp2+/− mice have been limited because of potential phenotypic variability due to X chromosome inactivation effects. Even if the phenotypic variability is high in heterozygous female mice, this gender nonetheless represents the real RTT model and every effort should be made to confirm the results obtained in null males in heterozygous female mice. In the Mecp2 KO-B female mice, the first phenotypic signs appear around the 12th week of age consisting of abnormal positioning of the rear legs. Later, they tend to develop obesity and respiratory irregularities (around the 9th month) can be found in heterozygous females [12]. This last point is quite intriguing since most RTT patients exhibit an extremely low body weight. Several hypotheses could be proposed. A distinctive hormonal regulation in RTT girls and Mecp2 heterozygous female mice could explain the differences in weight gain. Alternatively, permanent access to food in mice could contribute to this phenomenon. Nevertheless, heterozygous female mice are definitively less affected than null males.
2.1.2 Mecp2 KO-J
Concomitantly to the Bird model, the Jaenisch model (or Mecp2tm1.1Jae or Mecp2Jae) [14] has been created with a deletion of exon 3 (encoding the DNA methyl-binding domain) and a portion of exon 4 of MeCP2. This model is generally considered as a model completely devoid of MeCP2 but nevertheless, it is worth noting that a small portion of MeCP2 is transcribed. Accordingly, if immunohistochemistry detects no MeCP2 protein, western blot can reveal small peptides in mutant brain mutant. Because the N-terminus is always present, including for example the NLS (Nuclear localization sequence) and a part of the TRD (transcriptional repression domain), it would be wise to consider the consequences of the expression of this mutant peptides [14, 15]. In vivo, KO-J mice appear normal until the 4–5th weeks of postnatal life. They display progressive behavioral disorders (impairment of motor skills, “hind limb clasping,” irregular breathing, tremors, epileptic seizures, weight loss), which also involve various neurological functions such as anxiety, social interactions, learning and memory. KO-J female mice have no phenotype before 4 months, even if one can observe a slightly lower weight when paired with wild type females at around 5 weeks of age. From the fourth month a decrease in locomotor activity appears. Weight gain has also been described and from the sixth month the females have trouble in positioning the hind limbs as well as slight respiratory irregularities [14–16].
2.1.3 Mecp2 TAM
Another knockout mouse (Mecp2tm1Tam) has been created carrying a deletion of the entire exon 3 and part of exon 4, replacing the coding sequence for the MBD with a floxed Pgk-Neo cassette [17]. In this model, the region encoding the TRD and the C-terminal part Mecp2 is not transcribed [17]. This construction leads to a complete lack of Mecp2 transcript and protein in these mice. The authors generated Mecp2tm1Tam line on a pure 129 or mixed 129 × C57BL/6 background, which could partially affect some of the behavioral tests. Mecp2tm1Tam male mice exhibit a phenotype close to that observed in Mecp2 KO-B and Mecp2 KO-J. For instance, the locomotion of the mice was assessed by their activity on freewheels and Mecp2tm1Tam male mice exhibit a significantly lower level of activity. Mutant male mice remain for a shorter time on the accelerated rotarod. In addition, Mecp2tm1Tam male mice present a reduction of fear and anxiety on the elevated plus maze apparatus. Finally, Mecp2tm1Tam male mice show impaired fear conditioning and contextual association. In addition to these classical test authors mentioned that mutant mice frequently present an abnormal gait, a folding of rear legs, tousled hair, abnormal breathing, tremors and seizures (however, no statistical study was reported for these parameters). From 5 weeks, the condition of male Mecp2 Tam begins to worsen. No difference in body weight was observed between wild-type females and heterozygote female mice. At 6 months females present front limb stereotypies and motor deficits [17, 18].
2.1.4 Mecp2 Hoxb1
In order to study the role of MeCP2 in the regulation of the autonomic functions, the group led by Jeffrey Neul in Houston [19] crossed the floxed MeCP2 mouse with Hoxb1-Cre mice [20] that expresses the Cre recombinase in the distal part of the hindbrain: the caudal pons (including the locus coeruleus), the medulla oblongata and the spinal cord. This area contains the respiratory centers such as the retrotrapezoid core and the pre-Bötzinger complex but also the noradrenergic cell groups A5, A1/C1, A2/C2 which represent strong respiratory modulators [21]. In addition to the brainstem, these mice exhibit also a lack of Mecp2 in many peripheral autonomic tissues. The analysis of the phenotype of Hoxb1-cKO mouse reveals a disruption of motor coordination with the conservation of behaviors depending of a neural substrate preferably located in the forebrain. The cortical activity recorded by EEG is normal, suggesting that the abnormal activity observed in MeCP2−/y mice is the consequence of a lack of MeCP2 in areas other than those expressing Hoxb1. It is important to note that these data were obtained using a 129S6 genetic background while most other studies with models of Mecp2 dysfunction were studied in a C57BL/6 background. Genetic background differences need to be taken into account to interpret the results of any study using mice [22]. When they grow older these mice tend to be obese, develop heart arrhythmia, and have abnormal baseline breathing. They have a reduced life span [19].
2.1.5 Mecp2 CaMKII
After the initial publication of the KO-J mouse model (see above), the same group inactivated Mecp2 in the postmitotic forebrain neurons expressing CaMKII to assess their contribution to the Mecp2-null phenotype. In this model the mutation was induced after the second postnatal day. The loss of Mecp2 preferentially in excitatory neurons of the forebrain causes a less severe phenotype than the constitutive knockout mice [14]. A more detailed analysis of the behavior of these mice at postnatal stages has shown that conditional inactivation of Mecp2 in the forebrain is sufficient to induce motor deficits (coordination), anxiety, and impaired social interactions [23].
2.1.6 Mecp2 Floxed TH-cKO, Pet1-cKO
To study the impact of the lack of MeCP2 in catecholaminergic or serotonergic neurons, the group of Huda Zoghbi invalidated Mecp2, respectively, in the neurons expressing tyrosine hydroxylase (Th), the rate-limiting enzyme in the catecholamine synthesis [24] or in neurons expressing PC12-ets factor 1 (Pet1) a factor involved in the acquisition of the neurochemical identity of serotonergic neurons [25] using the Cre-loxP system. In the Th-cKO mice, the deletion is occurring in Th-positive neurons including dopaminergic nuclei (substantia nigra pars compacta, ventral tegmental area) and noradrenergic (locus coeruleus, medulla) central and peripheral tissues such as the catecholaminergic medulla. At the neurochemical level, this conditional KO causes a large reduction of dopamine and norepinephrine levels. From a behavioral point of view, animals exhibit impaired motor function but retain normal memory and motor skills learning and show no signs of anxiety or impairment of social interactions. It is interesting to note that the respiratory phenotype does not seem to be exacerbated by the lack of MeCP2 in Th-expressing neurons. Pet1-cKO animals are more aggressive when exposed to conspecific partner mice. Surprisingly, grooming or stereotyped movements, which are known to be modulated by serotonin, are not modified. Similar to the Th-cKO mice, the deletion in the Pet1 neurons does not aggravate the respiratory phenotype. Overall, the two conditional knockout lines (cKO and Th-Pet1-cKO) survive up to 18 months of age while constitutional MeCP2−/y mice die around 69 days after birth. More recently, Lang and colleagues [26] created a new mouse model with a selective preservation of MeCP2 in catecholaminergic cells. This catecholaminergic rescue is sufficient to improve the behavioral phenotype of male and female Mecp2-deficient mice. Motor coordination is improved and epileptic manifestations are reduced, and the lifespan of the animals is significantly increased.
2.1.7 Mecp2 Sim1
To better understand the role of the hypothalamus in RTT, the group of Huda Zoghbi selectively inactivated Mecp2 in single minded-1 (Sim1)-positive hypothalamic neurons [27]. These Mecp2-Sim1 mice present no obvious deficits in motor coordination, tremors, learning, or memory. However, they have an enhanced physiologic response to stress associated to an increase of corticosterone secretion compared to the wild-type mice. This enhanced physiologic response to stress has been previously found in RTT patients who present an increase of urinary cortisol [28]. In the open field, Mecp2 Sim1 male mice explored the center significantly less than wild type mice, a finding consistent with enhanced anxiety-like behavior. However, light/dark box assay, one of the most used tests for studying anxiety-like behavior, did not reveal differences. The authors also found a role of Mecp2 in the regulation of social and feeding behaviors that largely rely on the hypothalamus. These mice are more aggressive and they engage in significantly more tail rattles and aggressive attacks to a new intruder than their control littermates. Finally, Mecp2 Sim1 male mice present a normal activity and normal basal metabolic rates but are hyperphagic and frequently obese possibly due to the increase in leptin and neuropeptide Y levels [27]. This last point is quite intriguing since in KO models B or J only heterozygous female mice become obese while adult male are usually skinny. Perhaps, the lack of Mecp2 in the whole body or in the hypothalamus alone will dramatically change the feeding behavior. Another possibility could be that the pure FVB background used in this study could affect the feeding behavior differently compared to B or J knockouts maintained on pure C57BL/6 background.
2.1.8 Mecp2 Viaat
RTT is characterized by motor abnormalities, seizures, autistic features, and stereotyped behaviors. Most of these functions are regulated by GABA signaling, the most important inhibitory neurotransmitter in the brain. Chao and colleagues [29] produced a conditional Mecp2 knockout in neurons expressing the vesicular GABA transporter (vesicular amino acid transport, Viaat). Viaat-Mecp2−/y mice are indistinguishable from controls until 5 weeks of age, when they exhibit hind limb clasping, forelimb stereotypies, and a significant increase of grooming behavior associated to self-injury. At 9 weeks, these mice present impaired motor coordination, learning, memory, and respiratory irregularities. In addition, EEG recordings reveal hyper excitability without LTP modifications. Social interactions were increased whereas no signs of anxiety were detected. This Mecp2-Viaat model seems to recapitulate most of the deficits observed in Mecp2 null mice.
2.1.9 Mecp2 Adult (AKO)
Patients affected by RTT are normal at birth and progressively display clinical features during the first years of life. The progressive appearance of Mecp2-deficiency symptoms suggests that the function of Mecp2 in the maturing nervous system is critical for establishing normal adult neurological function. Two different groups developed an adult onset model of RTT by crossing mice harboring a floxed Mecp2 allele and a tamoxifen-inducible CreER allele to delete Mecp2 when animals are fully mature [30, 31]. By 10 weeks, McGraw and colleagues found that AKO mice are less active, have an impaired motor coordination (accelerating rotarod) and abnormal gait, and develop hind limb clasping similar to 10–11-week-old KO mice. AKO mice also develop motor abnormalities and impaired nesting ability together with impaired learning and memory. Using accelerating rotarod, Cheval and colleagues found that the latency to fall is significantly shorter for AKO mice compared to controls. Moreover, performances across the 3 days of trials suggest that AKO mice are unable to learn the motor task, but it is unclear whether this is due to a learning deficit or altered motor coordination. Most interestingly, both studies show that a removal of MeCP2 in older mice rapidly leads to their death. These results indicate that Mecp2 has an important role in neuromaintenance and associated behaviors, even if the brain is fully mature.
3 Knock-In and Transgenic Models
3.1 Mecp2 Lox-Stop (“Rescue”)
To control the activation of Mecp2, Guy and colleagues [32] introduced a floxed Stop cassette into the coding region of Mecp2. This cassette can be removed on demand using tamoxifen injections to induce the expression of the Cre recombinase coupled with an estrogen receptor (Cre-ER) response element [33]. Animals re-expressing MeCP2 before 3 weeks of age do not develop signs of disease. If the rescue is performed later, when the mice are severely affected, there is a considerable reduction of cellular, physiological, and behavioral deficits associated to a large increase of the life span of the “rescued” animals [32]. It is important to note that half of tamoxifen treated mice died prematurely, probably due to a sudden and not fully controlled Mecp2 reactivation. Along the same line, it is also interesting to note that untreated Lox-Stop mice displayed significantly increased survival compared to the null mice, indicating a possible “leakage” of the construct and therefore a production of a low amount of Mecp2. Nevertheless, “reactivated” mice exhibit a reversion of the structural deficits in cortical neurons, of neuronal arborization and long-term potentiation [34, 35]. In vivo, the breathing pattern of tamoxifen treated mice is largely stabilized. In addition, motor coordination, muscle strength, and posture are also improved causing a global amelioration of the sensory-motor tasks [35]. Altogether, these results show that even if Mecp2 is reactivated when the phenotype is present, “reversibility” is still possible.
3.2 The Mecp2-308 Mouse Model
The first knock-in model that was created is the Mecp2-308 mouse produced by Shahbazian and colleagues [36]. It models an early truncation of Mecp2 at amino acid 308, after the methyl-binding and transcription repression domains of the protein. The Mecp2 putative nuclear localization signal is preserved and the mutant allele produces a 52 kDa protein (instead of 74 kDa for the wild-type Mecp2 protein), that localized in the nucleus, as expected [36]. This Mecp2-308 allele was described to be hypomorphic [37–39]. However, there is no formal demonstration that this allele is indeed hypomorphic; that is, it retains a partial function normally accomplished by the wild type Mecp2 protein as previously mentioned. The 308X mutation does not reproduce a mutation found in the RTT patients. The choice of a truncation at amino acid 308 was based on the hypothesis that the mutant allele would partially preserve the function of the Mecp2 protein and allow for better survival of the affected males, by comparison with the knockout models. Indeed, male knockout mice live approximately 60 days (see above) while Mecp2-308 males live at least for 1 year. In humans though, truncations or deletions of MECP2 may give rise to very similar phenotypes (i.e., classical RTT). It is thus difficult to figure out why modeling a truncation in the mouse should make the disease milder than what it is in the knockout animals. In this respect, modeling the R306C or another human missense mutation frequently found in RTT would have been an interesting alternative because a whole length (although functionally abnormal) Mecp2 protein would have been present in the cells of the affected animals.
Another specificity of the Mecp2-308 model is that most studies are performed with animals maintained on a mixed 129/SvEv × C57BL/6J or pure 129/SvEv genetic backgrounds while most knockouts mice were maintained on a pure C57BL/6J background. Given that there are behavioral differences between different genetic backgrounds [40, 41], the direct comparisons made between the knockout models and the Mecp2-308 model are to be considered with caution. This is especially important when the phenotype is mild, such as the phenotype of the Mecp2-308 mice on the 129/SvEv × C57BL/6J background.
The Mecp2-308 pups were reported to display an abnormal behavior as early as 3, 6, or 9 days of age in the C57BL/6J background [42]. However, nest building and utilization are abnormal and abnormal social interactions are observed for the Mecp2-308 males using a partition test [43]. Later on, when tested for motor skills and diurnal behavior on a pure 129/SvEv background, 9–10-week-old Mecp2-308 males have no detectable motor phenotype (other than forepaw stereotypies and body tremor) [43]. Males display a “subtle tremor” phenotype appearing between 6 and 16 weeks of age together with stereotypic forepaw movements. These stereotypic movements were not clearly observed in a C57BL/6J background [39]. The motor function of male Mecp2-308 animals is normal when measured on the accelerated version of the rotarod at 3 months of age and the apparatus needs to be modified (elimination of the grips from the rod surface) to detect a subtle impairment in 5-month animals [36]. These animals show impaired capabilities in the vertical pole and wire suspension tests. Male Mecp2-308 mice also have a reduced activity in the open field. The overall speed and rearing are similar between Mecp2-308 and wild-type mice animals but the time spent in the center of the arena remains unchanged in Mecp2-308 male mice while it increases for wild-type animals. The abnormal parameters measured in the open field were used in several studies demonstrate that the Mecp2-308 mice have “increased anxiety” [36, 38]. However, in the resident-intruder, conditioned fear and Morris water maze tests, no statistically significant difference for most parameters were found [36]. The “tube test” (used to evaluate social dominance) revealed that wild-type mice retreat when they are facing a Mecp2-308 mouse in an acrylic tube [36], while another study showed that the Mecp2-308 male mice on a pure 129/SvEv background behave abnormally in the Morris water maze and contextual fear conditioning test and have difficulties to learn and to remember [44].
At 4 months of age, Mecp2-308 males have increased anxiety using the open field assay, the elevated plus maze and the light/dark box [38]. This trait was likely due to the overexpression of corticotropin releasing hormone (Crh) in this model but Crh expression was found to be normal in the knockout model [38]. In RTT patients, plasma cortisol (corticosterone in rodents) levels are normal [45, 46].
After 8 months of age, a proportion of mutant animals have oily and disheveled fur. Spontaneous seizures were described in one study [36] but were absent from another [47], although the same model and the same genetic background were used.
These various findings lead Shahbazian and colleagues [36] to conclude that this new model recapitulates a classic Rett phenotype. This is a surprising conclusion given that the Mecp2-308 mice behave normally in several motor and behavioral tests (conditioned fear or Morris water maze for instance). In addition, the neuropathology is normal in the Mecp2-308 mouse as is its brain weight [36, 44]. In RTT patients, brain pathology reveals reduced dendritic arborization, reduced soma size and microcephaly [48]. Although the Bdnf gene was shown to be a target of Mecp2 and Bdnf levels are abnormal in mice with knockout alleles, Bdnf levels are not altered in the Mecp2-308 male mouse [44]. Hence, based on published literature, the Mecp2-308 does not completely recapitulate the behavioral and molecular phenotype of RTT.
Most studies were performed using the male mice to avoid the interference of X-chromosome inactivation (XCI). However, female mice should be the model used to study RTT. Female Mecp2-308 mice were studied in a pure 129/SvEv background, but no behavioral testing was reported for this gender [36]. Homozygous Mecp2-308 females were reported to die at a “very young age” [49]. This difference between sexes is surprising and suggests that males and females do not behave similarly when the Mecp2-308 allele is the only allele available during the development. Females are impaired at 35–39 weeks of age and a majority (69 %) display stereotypic forepaw movements. They present deficiency in the dowel and wire suspension tests. Female Mecp2-308 mice show reduced social preference and social interaction together with decreased locomotor activity [50]. The Mecp2-308 mutation in males increases sociability, and specifically affiliative social behavior but, unfortunately, female animals were not studied [39]. Young and colleagues [37] studied female Mecp2-308 mice on a pure 129/SvEv background at 24 or 48 weeks of age. Tremor and stereotypic paw movements were scored, together with the appearance of disheveled fur and periocular lesions (although these two latter traits have not been described in other models of Mecp2 deletion). They found that the penetrance of the studied traits in Mecp2-308 females varies between 44 and 68 % at 48 weeks and that most female mice favor the expression of the X chromosome carrying the wild-type allele [37]. XCI patterns are susceptible to alter disease severity depending on the percentage of cells expressing the mutated X chromosomes. In humans, most RTT patients present random XCI pattern [51] and it is reasonable to hypothesize that XCI plays a role in modulating disease severity. However, the location and type of the mutation and their consequences on the Mecp2 function likely plays an important role as well. Indeed, the phenotype of the heterozygous Mecp2-308 females is significantly different from the phenotype of the heterozygous knockout females (see above). Given that XCI patterns are similar in both models, it is reasonable to propose that disease severity is modulated by factors other than the percentage of mutated allele being expressed. Unfortunately, this latter aspect has currently not been addressed in Mecp2-deficient mouse models.
3.3 The Tau-Mecp2 Mouse Model
Early studies claimed that Mecp2 was expressed in neurons but not in glia [52]. Although we now know that this statement was incorrect (Mecp2 is expressed in glia, but at significantly lower levels), much attention was paid to post-mitotic neurons to explain the RTT phenotype. To specifically assess the consequences of Mecp2 dysfunction in neuronal cells, the Mecp2 gene was knocked into exon 1 of the Tau locus [53]. Crossing C57BL/6 Tau-Mecp2 mice with C57BL/6 Mecp2-J knockouts produced “rescued” animals heterozygous for the Tau-Mecp2 locus. These animals were tested for body, brain weight and nocturnal locomotor activity was grossly evaluated between 4 and 6.5 months of age using cages equipped with infrared beams. All these parameters reach wild-type levels in the “rescued” male mice [53]. No further analysis of these “rescued” animals was published.
The Tau-Mecp2 mouse was also used to study the consequences of Mecp2 overexpression. Homozygous mice (expressing Mecp2 from the wild-type X chromosome and from the Tau locus) have profound motor dysfunction, absence of mating behavior and severely impaired growth by weaning age, probably due to their inability to feed normally [53]. A gross evaluation detected no phenotype in the heterozygous Tau-Mecp2 animals [53]. However, a more detailed analysis showed that these animals display motor coordination deficits on the rotarod and increased anxiety in the light/dark box and elevated plus maze tests [54]. The male knock-in mice have increased freezing behavior in the fear-conditioning test. However, this is not an indication of increased associative learning since extinction training revealed that Tau-Mecp2 mice are not able to return to baseline freezing when trained for 9 days in the absence of cue [54]. The mice were also less interested in novel objects in the novel object recognition task. The conclusion of this follow up study using the Tau-Mecp2 male mice is that these animals have deficient learning and memory capabilities. These observations are correlated with impaired hippocampal long-term potentiation [54]. Similar to observations in the Mecp2-308 model, Bdnf levels are not altered in the Tau-Mecp2 male mouse [54].
The Tau-Mecp2 mouse was reported to model several aspects of the human MECP2 duplication syndrome. The mouse has indeed a number of phenotypic RTT characteristics but that knock-in model also has several drawbacks. First, the Mecp2-e2 isoform (the less abundant isoform in the brain) was used to build the knock-in allele because the Mecp2-e1 isoform was unknown at the time. Second, the Mecp2 protein produced by the Tau-Mecp2 is a fusion protein that includes the N-terminal sequence beginning with 31 amino acids belonging to Tau. Mecp2 fusion proteins might have a different behavior and it is known that mutations affecting the stop codon of Mecp2 cause a “naturally tagged” protein extended by 26 amino acids and typical RTT [10]. Third, the Tau locus produces several isoforms, also expressed outside of the central nervous system [55]. For instance Tau protein can be found in the peripheral nervous system and in smaller amounts in the kidneys, lungs, and testes [56]. Once considered strictly axonal, Tau is now also reported in the somatodendritic compartment of astrocytes and oligodendrocytes [57, 58]. Fourth, the level of expression of Tau is much higher than the wild-type level of Mecp2. Tau-Mecp2 fusion protein is increased two- to fourfold in the brain of the transgenic animals.
3.4 The I-Mecp2 Mice
This transgenic model carries and inducible Mecp2 transgene (I-Mecp2) placed under the control of a minimal CMV promoter activated by a tetracycline responsive element [59]. The characterized transgenic line (Tg15) globally expresses Mecp2 at wild-type levels, but the expression in individual neurons is different from the wild type situation with cells expressing Mecp2 at higher or lower levels. When crossed with tetracycline transactivator (tTA)-expressing mouse lines, the I-Mecp2 can be induced in cell populations where tTA will be expressed.
The rationale for building this model was that if specific clinical signs arose from dysfunction of particular neuronal populations, it should be possible to alleviate these signs by (re)expressing Mecp2 in the corresponding populations. To achieve this goal, two different tTA-expressing lines were used. In the first line, tTA was expressed under the control of rat enolase 2 promoter (Eno2-tTA). Eno2 is a neuron-specific enolase expressed in terminally differentiated neurons and neuroendocrine cells and the Eno2-tTA mouse used by Alvarez-Saavedra and colleagues was shown to express tTA in the striatum and the cerebellum [60]. The second line used the calcium/calmodulin-dependent protein kinase IIα promoter to express tTA (CamKIIα-tTA). CamKIIα is expressed in several regions of the brain but the line used by Alvarez-Saavedra and colleagues was shown to express tTA mainly in the forebrain [61].
I-Mecp2 mice were crossed with Eno2-tTA or CamKIIa-tTA to generate double-transgenic mice. The resulting animals were crossed with Mecp2-308 or Mecp2 KO-B animals. According to that sophisticated experimental design, it should be possible to activate Mecp2 in specific cell populations to try, (1) to prevent the appearance of clinical signs, or (2) to reverse specific phenotypic traits in these mutant animals once the clinical signs have appeared.
The Mecp2-308 model has an identical phenotype when crossed with the Eno-tTA I-Mecp2 line. When crossed with the CamKIIα tTA line, the performance of the Mecp2-308 is only improved in the wire suspension test at 20 weeks of age, a very subtle difference. The performance of the Mecp2 KO-B line is slightly improved in the open field at 8 weeks of age when crossed with the CamKIIα tTA line. All other parameters (including life span) remain unchanged. This is an interesting observation because deletion of Mecp2 in CamKIIα-expressing neurons causes a neurobehavioral phenotype [14].
Here again, a Mecp2-e2 isoform was used to produce de I-Mecp2 mice as mentioned before. Mecp2-e2 is not the major brain isoform and this might be the reason why Alvarez-Saavedra and colleagues were unable to rescue the phenotype of the Mecp2-308 or Mecp2 KO-B animals. Unfortunately, the phenotype of the I-Mecp2 lines (overexpressing Mecp2-e2 with no phenotype) was not studied in detail and cannot be compared to the phenotype of the Tau-Mecp2 mice (also overexpressing Mecp2-e2 but presenting a phenotype) [53].
3.5 The MECP2Tg1 Mouse
MECP2Tg1 is a mouse line that overexpresses the human MECP2 protein due to the insertion of a human PAC clone containing the MECP2 locus [62]. Five transgenic lines were generated. Among these, the MECP2Tg1 expressed the two isoforms of MECP2 at wild-type levels, has the expected tissue distribution (e.g., high expression in brain, lung, and spleen) and nuclear localization. When crossed with Mecp2 KO-B mice, the resulting Tg1/KO animals are similar to wild types at 33 weeks of age [62]. High-expressing mouse lines manifest severe abnormalities within days to week. Male MECP2Tg1 mice were characterized in detail [62]. They have a highly variable lifespan (death between 5 months and normal life span). When evaluated between 6 and 20 weeks of age, these mice had subtle phenotypic traits such as increased rearing in the open field and reduced movements on the dowel test. Performance on the rotarod was improved over time when male MECP2Tg1 mice were tested between 10 and 20 weeks of age suggesting an improvement of the motor function learning [62]. Hippocampal learning was improved in the MECP2Tg1 animals tested in a fear-conditioning paradigm; and synaptic plasticity was enhanced in the hippocampal area CA1 [62].
This approach and the results generated by Collins and colleagues [62] are intellectually appealing. However, there are a number of unresolved issues. First, the performances of the transgenic animals seem to be improved when they have a double dose of Mecp2 (more exactly a mixture of mouse Mecp2 and human MECP2). In humans, males with a duplication of MECP2 present motor impairments and cognitive deficits early in life [9]. The authors of the MECP2Tg1 mice question the Tau-Mecp2 model because the absence of Tau could explain part of its phenotype. However, the use of MECP2Tg1 model raises the same question because the insertion site of the human PAC was not characterized and there is a possibility that this insertion affects the expression of another gene and causes part of the observed “improvements.” One way to study the rescuing effect should have been to analyze other mouse lines with different insertion sites to demonstrate that the observed effects are solely due to the changing in expression of the dose of the human MECP2 protein in the mouse brain cells. Finally, it is not known if regulatory sequences are identical in the mouse and in humans. The fact that MECP2Tg1 male mice can, in some cases, have a normal life span pinpoints the difference existing between the two species.
3.6 The Mecp2Flox/y Mouse
The Mecp2Flox/y allele is hypomorphic and allows the expression of 58 % wild type level of Mecp2. It was engineered during the construction of the Mecp2 KO-B line [12]. It contains a 3′UTR insertion potentially preventing the expression of the longest isoform of Mecp2 mRNA. Mecp2Flox/y mice have been studied by two groups [63, 64]. In both studies, the expression of the Mecp2 protein was found in the nucleus but protein levels differed from 42 % [63] to 70–10 % of wild-type levels [64]. Mutant animals have a normal life span but they tend to gain weight on the 129S1/SvImJ × B6/CBA mixed background [64]. The Floxed allele behaves differently depending on the genetic background. On the 129S6/B6 background, the Mecp2Flox/y animals tend to fall earlier from an accelerating rotarod and to display altered performances on the dowel and suspended wire tests, while there is no difference in the three tests on the 129S6/FVB background. The dowel test also reveals subtle differences with mice taking more time to reach the platform on the mixed background used by Kerr and colleagues [64] while the suspended wire test was normal for these animals. In the tail flick and hot plate tests, the two genetic backgrounds demonstrate the ability to detect the painful stimuli in the periphery (tail flick test normal) but have a deficit in pain recognition (abnormal hot plate test results) [63, 64]. In a fear conditioning task, the hypomorphic allele leads to decrease freezing in the context- and in the cue-dependent versions of the test on the 129S6 × FVB background but not on a 129S6 × C57BL/6 background. Altered social behavior and deficits in nest-building abilities were also observed for the Mecp2Flox/y mice in the 129S6 × C57BL/6 background. In the open field, the Mecp2Flox/y mice on the 129S6/FVB background shows increased rearings and time spent in the center of the arena [63] but the same model on a mixed 129S1 × B6/CBA background shows performance identical to wild-type animals [64]. Kerr and colleagues were not able to detect differences in the elevated plus maze in the same animals but evidenced subtle social interaction abnormalities in the three-chamber test with the Mecp2Flox/y mice spending more time with unfamiliar partners than wild-type animals.
From their study of the Mecp2Flox/y mice, Samaco and colleague conclude that the expression of approximately a half dose of Mecp2 is sufficient to produce a neurological phenotype. Indeed, several neurobehavioral tests detect a difference between mutant and wild-type mice. However, there is also a difference between mutant animals when the genetic background is different (129S6 × C57BL/6 vs. 129S6 × FVB vs. 129S1 × B6/CBA). When phenotypes are subtle, like it is here for these two studies, background differences may exacerbate or level abnormal neurobehavioral phenotypes. In such a case, the sensitivity of the tests used needs to be carefully evaluated.
3.7 The A140V Mouse Model
The A140V mutation in the MECP2 gene was initially identified in male individuals with non-syndromic intellectual disability [65]. The same mutation was identified in several individuals with intellectual disability, motor deficits and dysmorphic features [11, 66]. It was also shown to cause an X-linked syndrome of psychosis, pyramidal signs, and macro-orchidism (PPM-X) [67]. The mutation is inherited in familial cases and females can be unaffected or have a milder phenotypic presentation. Carrier females have random X-chromosome inactivation in their lymphocytes. None of the A140V carriers have clinical RTT.
The A140V mutation was knocked-in in the mouse genome [68]. This allele reproduces a mutation found in human affected with a Mecp2 group II mutation (see Table 1). These mice are not a “mouse model of RTT” per se [68] but rather a model of a severe to moderate X-linked intellectual disability caused by a MECP2 mutation.
The A140V mutation is located in the methyl binding domain of Mecp2 both in humans and mice. It does not reduce the affinity of Mecp2 for methyl CpGs but it impairs its interaction with its partner protein ATRX, also involved in X-linked intellectual disability [69].
In the brain of the A140V male mice, the mutant Mecp2 protein has a normal distribution and its transcript is present in normal amounts [68]. The gross morphology and life span (after 14 months of study) of the A140V animals is normal although mutant males seem not to be able to breed. Cells are more densely packed in the cerebellum of the A140V mice studied at 5 months of age. Although cell packing density was shown to be increased Scholl analysis performed at 2–3 months of age in the somatosensory cortex layers II/III revealed decreased branching complexity. Unfortunately, brain size, cognitive capabilities, motor function, or behavior of the mutant mice have not been assessed. Further studies are needed to demonstrate that the A140V model is a valuable model. The reported parameters, as they currently stand, are not specific and were found in multiple models of intellectual disability.
3.8 The R168X Mouse Model
Lawson-Yuen et al. [70] reported a mouse strain carrying the R168X mutation that is frequently found in patients with RTT (group I, mutation Table 1). As expected for this group of mutations, hemizygous male mice are more severely affected than females with a life span of 12–16 weeks. Female mice display hind limb clasping and breathing irregularities by 6 months of age. These animals were maintained on the 129S6/SvEvTac genetic background but no neurobehavioral characterization was reported. A similar knock-in model was produced by Brendel and colleagues more recently [71] in order to target aminoglycosides and to identify candidates for read through therapy in RTT patients, but no neurobehavioral characterization was reported. This model represents probably one of the most interesting models of RTT reproducing a mutation found a large number of typical RTT patients.
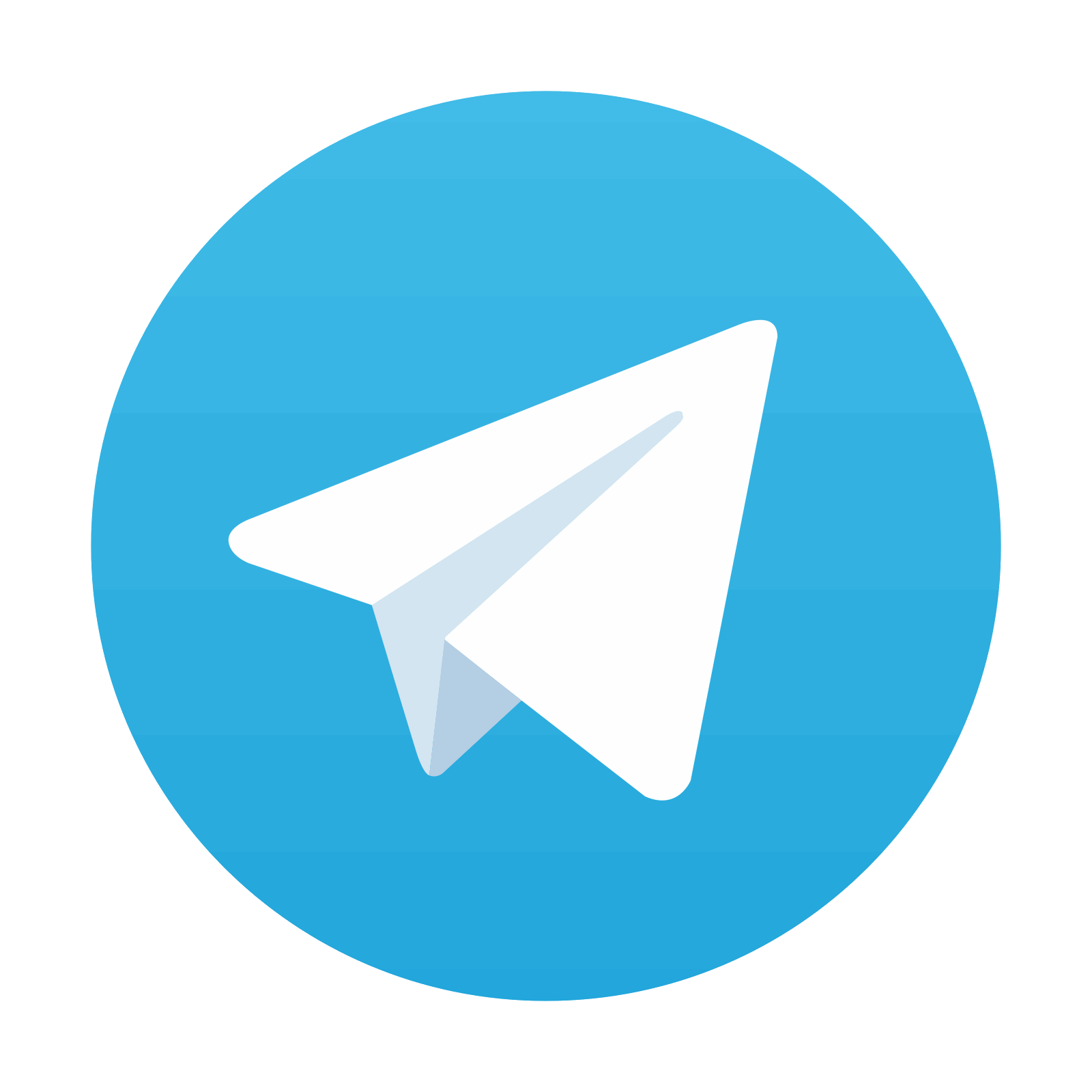
Stay updated, free articles. Join our Telegram channel

Full access? Get Clinical Tree
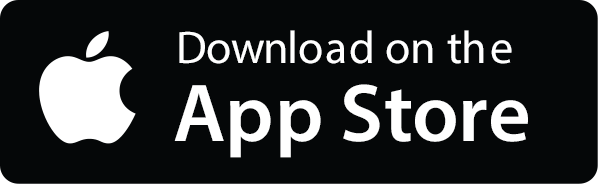
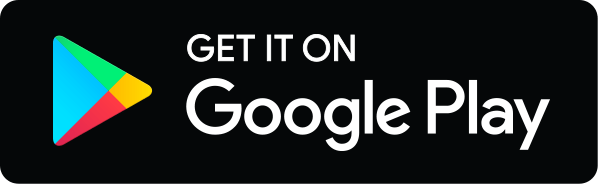