Neurochemistry, Pharmacodynamics, and Biological Psychiatry
George M. Anderson
Andrés Martin
Introduction
Continuing advances in basic neurobiology and psychopharmacology have led to a greatly expanded knowledge of brain functioning and hold the promise of better treatment and fuller understanding of childhood psychiatric disorders (1,2). The recent elucidation of the genetic bases of a number of single-gene childhood psychiatric disorders provides additional hope, and to some extent directions, for tackling the more complex molecular and biological influences in autism, attention deficit/hyperactivity disorder (ADHD), Tourette syndrome, anxiety, posttraumatic stress disorder (PTSD), depression, and suicide. Identification of causative factors in Parkinson’s disease, Huntington’s disease and Alzheimer’s disease further encourages the notion that the pharmacology of childhood psychiatric disorders can be made more rational and effective, and the biological bases of the relevant behaviors ascertained.
The three entwined areas of neurobiology, pharmacodynamics, and biological psychiatry can be introduced by first considering basic concepts in the separate realms of inquiry. Relevant neurobiology includes findings in the areas of neuronal circuitry, neural transmission and intracellular signaling. Phamacodynamics concerns the short- and long-term effects of drugs on neuronal function and structure. Insights and serendipitous findings from psychopharmacology reciprocally inform basic neuroscience and also have often been central to the hypotheses pursued in biological psychiatry. Recognition of the importance of genetics and complexity of psychiatric disorders is changing in fundamental ways how biological psychiatry is approached. Whether the field is termed molecular psychiatry, biological psychiatry, or clinical neuroscience, an increased focus on genetic influences and on behavioral components or endophenotypes has been prompted by a better appreciation of the scope and complexity of the endeavor.
General Considerations of CNS Functioning
Overview
Neuronal circuitry, synaptic neurotransmission, and intracellular information processing constitute three major levels of central nervous system (CNS) functioning critical to understanding mechanisms of psychotropic drug action and the biological basis of cognitive and behavioral processes.
Neuronal circuitry to a large extent defines and reflects the functional activity and organization of the CNS. Neuronal communication via neurotransmitter release is a fundamental mechanism of brain function. The release of neurotransmitters and neuromodulators, their mechanisms of action, and their effect on target neurons are complex and still not fully understood; however, despite the diversity of neurotransmitters and receptors in the human brain, all forms of neural communication have the common goal of modulating neuronal activity. This is achieved by changing either the electrical or biochemical properties of the cell. The balance of intracellular and extracellular ions characterizes the electrical properties of the neuron. At rest, there are more negatively charged ions inside than outside the cell, thereby creating a negative resting membrane potential. Decreasing the resting potential leads to excitation, increasing it, to inhibition. The more enduring properties of the neuron are determined by longer term processes regulating the expression of specific genes, the production of proteins, and the creation of a distinct metabolism.
Neuronal Circuitry
The adult human cerebral cortex has about 100 billion neurons; each neuron establishes about a thousand to ten thousand connections to other neurons. Neurons are arranged in distributed networks that play critical roles in the expression of human behavior (3). This involves the collection of sensory information through perceptual modules, the creation of a representation, and the production of a response. Here we focus on four major anatomic systems that are crucial for these three steps of information processing: the cortex, thalamus, basal ganglia, and medial temporal lobe (Figure 2.4.2.1).
The thalamus is the gateway to cortical processing of all incoming sensory information, here represented by the three major systems: somatosensory, auditory, and visual (Figure 2.4.2.1). Primary sensory cortices receive information from the appropriate input modules (sensory organ + thalamus).
The association cortex integrates information from primary cortices, subcortical structures, and brain areas affiliated with memory to create an internal representation of the sensory information.
The medial temporal lobe (including the hippocampus and amygdala) serves two major functions in the brain: to integrate multimodal sensory information for storage into and retrieval from memory, and to attach limbic valence to sensory information (e.g., pleasant or unpleasant, fight or flight).
The basal ganglia are primarily involved in the integration of input from cortical areas. The basal ganglia modulate cortical activity via a cortico-striato-pallido-thalamo-cortical (CSPTC) loop. The most prominent projections to the striatum arise from the motor cortex.
All major projections (solid lines) in this basic circuitry (Figure 2.4.2.1) are glutamatergic (using the amino acid neurotransmitter glutamate), except for the projections from the basal ganglia toward the thalamus, which are GABAergic (employing γ-aminobutyric acid). The glutamatergic neurons within each of the major components or regions of the circuit are under inhibitory control by GABAergic interneurons. In addition, four groups of densely packed neurons provide diffuse projections to all areas of the brain to modulate their functions: cholinergic neurons in the basal forebrain and brain stem, dopaminergic neurons in the substantia nigra and ventral tegmental area, noradrenergic neurons in the locus ceruleus, and serotonergic neurons in the raphe nuclei. The broken arrows in Figure 2.4.2.1 indicate the four neurotransmitter-specific projection systems. The relay of information from one neuron to another in these various circuits is usually effected by synaptic neurotransmission.
General Aspects of Synaptic Neurotransmission
Dendrites create a network of fibers providing the cell body of the neuron with input from other cells (Figure 2.4.2.2). The cell integrates these different inputs through modulation of the membrane potential, changes in second messenger systems and at the level of the nucleus (regulation of gene expression). The cell body is also the site of synthesis of nearly all cell-specific proteins, including transporters, receptors, and the enzymes needed for neurotransmitter production.
The axon is the output station of the neuron. The axon can be short (local circuit neuron) or long (projection neuron). If a deviation from the resting membrane potential is above a certain threshold, an action potential is created and travels downstream rapidly. The nerve terminal is the widened terminal part of the axon. It provides a small area of close contact with dendrites of communicating neurons: the synapse. Variations of this typical scheme include synapses between two terminals, two dendrites, and neurotransmitter release in medial parts of the axon.
As seen in Figure 2.4.2.2, the presynaptic neuron releases the vesicular stored neurotransmitter into the synapse and can express two types of proteins that affect synaptic communication: Presynaptic membrane-bound receptors can bind the intrinsic neurotransmitter (at autoreceptors) or transmitters of neighboring neurons (at heteroreceptors) and
affect the cell via intracellular messengers. One response, for example, is the modulation of neurotransmitter release (4). Presynaptic membrane-bound reuptake transporters pump the released neurotransmitter back into the cell (5). The released transmitter can undergo intracellular, extracellular metabolism (4B), or be repackaged in vesicules for rerelease.
affect the cell via intracellular messengers. One response, for example, is the modulation of neurotransmitter release (4). Presynaptic membrane-bound reuptake transporters pump the released neurotransmitter back into the cell (5). The released transmitter can undergo intracellular, extracellular metabolism (4B), or be repackaged in vesicules for rerelease.
The neuron receiving the input (postsynaptic cell) can be modulated via two different types of receptors (Figure 2.4.2.2
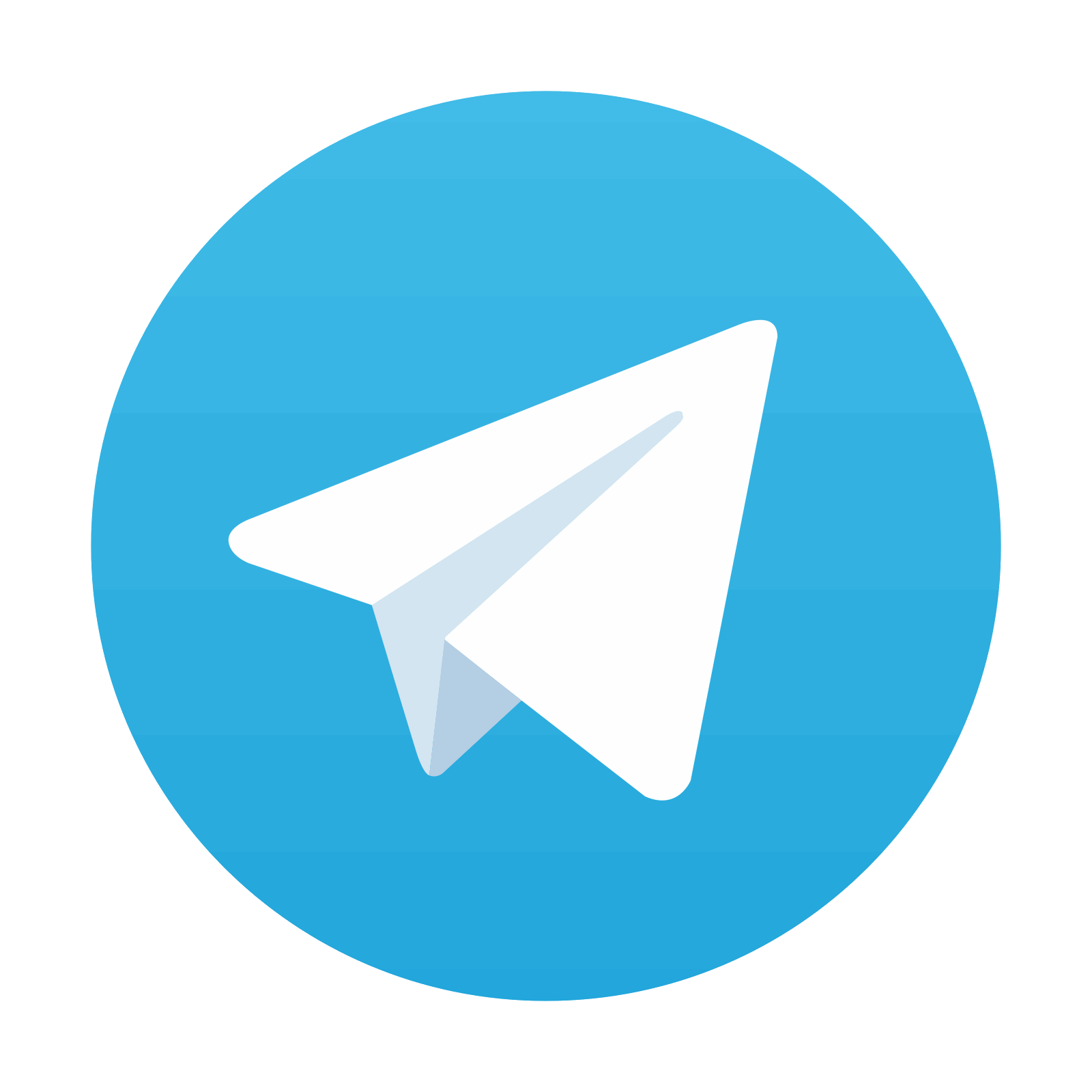
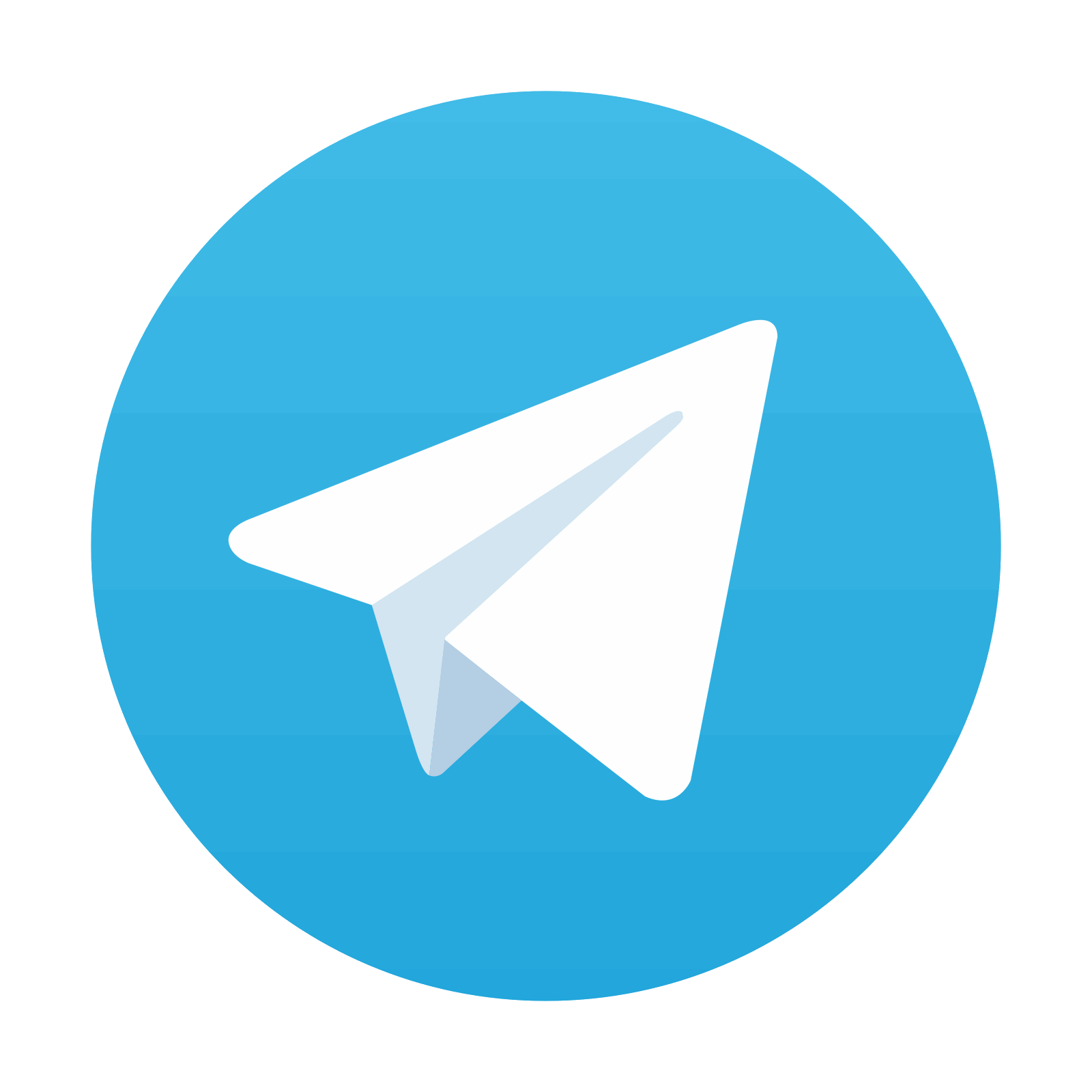
Stay updated, free articles. Join our Telegram channel

Full access? Get Clinical Tree
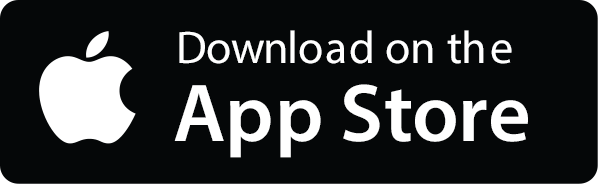
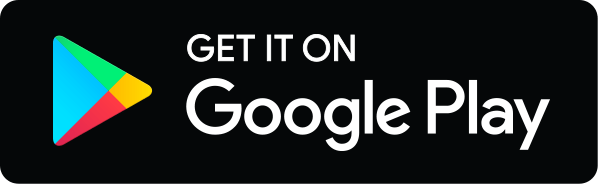
