Although urgent surgical hematoma evacuation is necessary for most patients with subdural hematoma (SDH), well-orchestrated, evidenced-based, multidisciplinary, postoperative critical care is essential to achieve the best possible outcome. Acute SDH complicates approximately 11% of mild to moderate traumatic brain injuries (TBIs) that require hospitalization, and approximately 20% of severe TBIs. Acute SDH usually is related to a clear traumatic event, but in some cases can occur spontaneously. Management of SDH in the setting of TBI typically conforms to the Advanced Trauma Life Support protocol with airway taking priority, and management breathing and circulation occurring in parallel rather than sequence.
Key points
- •
Although urgent surgical hematoma evacuation is necessary for most patients with SDH, well-orchestrated, evidenced-based, multidisciplinary, postoperative critical care is essential to achieve the best possible outcome.
- •
Acute SDH complicates approximately 11% of mild to moderate traumatic brain injuries (TBIs) that require hospitalization, and approximately 20% of severe TBIs.
- •
In most cases, acute SDH is related to a clear traumatic event, but in some cases, acute SDH can occur spontaneously.
- •
Management of SDH in the setting of TBI typically conforms to the Advanced Trauma Life Support (ATLS) protocol with airway taking priority, and management breathing and circulation occurring in parallel rather than in sequence.
Introduction
Acute subdural hematoma (SDH) develops between the dura and arachnoid membranes, usually due to tearing of the bridging veins that extend from the surface of the brain to the dural sinuses. Most cases of SDH result from low-pressure venous bleeding that eventually arrests due to rising intracranial pressure (ICP) and clot tamponade, but it is estimated that up to 20% to 30% of cases can result from arterial rupture. Linear translation of acceleration across the diameter of the skull in the lateral direction can produce stretch or torque injury to veins or arteries, resulting in SDH.
Acute SDH complicates approximately 11% of mild to moderate traumatic brain injuries (TBIs) that require hospitalization, and approximately 20% of severe TBIs. In most cases, acute SDH is related to a clear traumatic event, but in some cases acute SDH can occur spontaneously. Important causes of spontaneous SDH include use of anticoagulants or antiplatelet agents, and rupture of an intracranial aneurysm that is adherent to the arachnoid membrane, with consequent arterial bleeding into the subdural space (with or without associated subarachnoid hemorrhage). Less commonly, SDH also can result from bleeding due to arteriovenous malformations, vascular meningiomas, dural metastases, and spontaneous intracranial hypotension. Identification of a treatable cause of nontraumatic SDH is crucial, because reversal of anticoagulation may be all that is necessary in some patients, whereas others may require treatment of a cerebral aneurysm.
After traumatic acute SDH, coma is present at the onset of injury in 25% to 50% of cases, whereas another 12% to 38% experience progressive neurologic decline to coma over the next several hours. Coma is primarily the result of brain shifting and distortion, followed by elevated ICP and low cerebral perfusion pressure (CPP). Large acute SDH leads to compression and ischemia of the underlying brain within 4 to 6 hours in the absence of emergency evacuation. Posterior fossa SDH is especially treacherous because it can precipitate upward or downward herniation extremely rapidly.
Introduction
Acute subdural hematoma (SDH) develops between the dura and arachnoid membranes, usually due to tearing of the bridging veins that extend from the surface of the brain to the dural sinuses. Most cases of SDH result from low-pressure venous bleeding that eventually arrests due to rising intracranial pressure (ICP) and clot tamponade, but it is estimated that up to 20% to 30% of cases can result from arterial rupture. Linear translation of acceleration across the diameter of the skull in the lateral direction can produce stretch or torque injury to veins or arteries, resulting in SDH.
Acute SDH complicates approximately 11% of mild to moderate traumatic brain injuries (TBIs) that require hospitalization, and approximately 20% of severe TBIs. In most cases, acute SDH is related to a clear traumatic event, but in some cases acute SDH can occur spontaneously. Important causes of spontaneous SDH include use of anticoagulants or antiplatelet agents, and rupture of an intracranial aneurysm that is adherent to the arachnoid membrane, with consequent arterial bleeding into the subdural space (with or without associated subarachnoid hemorrhage). Less commonly, SDH also can result from bleeding due to arteriovenous malformations, vascular meningiomas, dural metastases, and spontaneous intracranial hypotension. Identification of a treatable cause of nontraumatic SDH is crucial, because reversal of anticoagulation may be all that is necessary in some patients, whereas others may require treatment of a cerebral aneurysm.
After traumatic acute SDH, coma is present at the onset of injury in 25% to 50% of cases, whereas another 12% to 38% experience progressive neurologic decline to coma over the next several hours. Coma is primarily the result of brain shifting and distortion, followed by elevated ICP and low cerebral perfusion pressure (CPP). Large acute SDH leads to compression and ischemia of the underlying brain within 4 to 6 hours in the absence of emergency evacuation. Posterior fossa SDH is especially treacherous because it can precipitate upward or downward herniation extremely rapidly.
Emergency resuscitation
Airway, Breathing, Circulation
The objectives in the emergency department are to undertake a rapid and systematic clinical assessment, and to institute immediate life-saving treatment. Management of SDH in the setting of TBI typically conforms to the Advanced Trauma Life Support (ATLS) protocol, with airway taking priority, and management breathing and circulation occurring in parallel rather than in sequence. As is the case with all forms of severe trauma, maintenance of oxygenation (Pa o 2 >60 mm Hg) and blood pressure (BP) at a mean arterial pressure (MAP) of 65 mm Hg or higher is the immediate priority.
Deciding on the optimal timing to secure the airway can be challenging. Intubation and ventilation may allow for expedited imaging, as well as safer interventions in the unconscious or agitated patient. Intubations in the setting of TBI and elevated ICP are more challenging, with higher rates of failure and complications. As a general rule, rapid sequence intubation (RSI) after an initial period of preoxygenation is the preferred strategy. RSI is the concomitant administration of a sedative and a neuromuscular blocking agent to render a patient rapidly unconscious and flaccid so as to facilitate emergent endotracheal intubation and to minimize the risk of aspiration. Multiple studies confirm the high first-pass success rate of RSI, which in experienced hands can exceed 90%.
Induction agents provide amnesia and blunt sympathetic responses, and can improve intubating conditions. Pretreatment with intravenous (IV) lidocaine 1.5 mg/kg 3 minutes before intubation may minimize any increase in ICP that can be associated with airway manipulation. Ketamine 1.5 mg/kg IV or etomidate 0.3 mg/kg are suitable induction agents because they tend to maintain MAP and do not increase ICP. Ketamine is a dissociative anesthetic agent that provides sedation and analgesia. Ketamine preserves respiratory drive and has both a quick onset of action and analgesic properties. Ketamine can cause sympathetic stimulation, and although it is known to maintain BP, there is concern that it may cause ICP elevation in TBI. Etomidate is a sedative-hypnotic agent that is frequently used for RSI that has putative neuroprotective properties. It provides no analgesic effect, so it often is given with fentanyl 1.5 μg/kg, administered during the pretreatment phase of RSI. For paralysis, a short-acting paralytic, such as succinylcholine 1.5 mg/kg IV, is ideal, because it wears off quickly and restores the neurologic examination within 10 to 15 minutes (use with caution in the setting of crush injury or hyperkalemia).
Longer-acting nondepolarizing alternatives include rocuronium (0.6–1.2 mg/kg) or cisatracurium (0.15 mg/kg).
Imaging
Neuroimaging in the form of a noncontrast computed tomography (CT) is integral to the diagnosis of acute SDH due to it widespread availability and speed. The sensitivity now approaches 100% with newer generation scanners. Brain MRI is more sensitive than head CT for the detection of extremely thin SDH, and tentorial and interhemispheric SDH. Acute SDH can be classified by (1) the age of the imaged blood (eg, acute, acute-on-subacute, or acute-on-chronic), and (2) by the maximal thickness of the subdural collection in centimeters ( Fig. 1 ). Additional parameters to assess are the extent of midline shift, the presence of effacement of the basal cisterns, and the extent of trapping of the contralateral ventricle. Because SDH can result from a ruptured cerebral aneurysm, noninvasive or digital subtraction angiography should be performed in suspicious cases of nontraumatic acute SDH.
Reversal of Anticoagulation
Reversal of all forms of anticoagulation is a medical emergency in patients with acute SDH. Coagulation panels are typically obtained on arrival; whether or not the patient is on some form of anticoagulation or antiplatelet therapy should be discerned through the history. The Neurocritical Care Society has published the most up-to-date guidelines for the emergency reversal of anticoagulation. Patients on oral anticoagulation therapy are estimated to have a 4- to 15-fold increased risk for SDH, leading to higher likelihood of hematoma expansion, an increased risk of death, and a worse functional outcome unless anticoagulation is quickly reversed. Patients on vitamin K antagonists, such as warfarin, are optimally reversed with vitamin K 10 mg intravenous push, followed by a 4-factor prothrombin complex concentrate (PCC) (Kentraor BeriplexV; CSL Behring, Inc, King of Prussia, PA) at a dose of 25 U/kg for an international normalized ratio (INR) of 2.0 to 4.0, 35 U/kg for an INR of 4.0 to 6.0, and 50 U/kg for an INR greater than 6.0. Recombinant factor VIIa (rFVIIa, NovoSeven; Novo Nordisk, Copenhagen, Denmark) was studied in TBI a phase II dose-escalation clinical trial and demonstrated a nonsignificant trend toward limiting hematoma contusion and hematoma expansion but no mortality benefit. rFVIIa also has been reported to be useful for emergency reversal of warfarin anticoagulation in patients with acute SDH. Given the associated risk of thromboembolic complications, however, routine use of rFVIIa cannot be recommended.
SDH associated with the newer direct oral anticoagulants (DOACs), such as the direct thrombin inhibitor dabigatran and the Factor Xa inhibitors, such as apixaban, edoxaban, and rivaroxaban, can lead to catastrophic bleeding, but until recently have had no antidote for emergency reversal. That has now changed with the arrival of idarucizumab (Praxbind; Boehringer Ingelheim, Ingelheim am Rhein, Germany) for dabigatran, which is currently approved by the Food and Drug Administration (FDA), and andexanet alfa (IndexXa; Portola Pharmaceuticals, San Francisco, CA) for the direct Factor Xa inhibitors, which is pending FDA approval.
Patients with acute subdural hematoma on antiplatelet agents should be managed initially by discontinuing antiplatelet therapy. Although platelet transfusion has long been considered a standard of care in the absence of evidence, the recently published PATCH trial compared platelet transfusion versus standard care after acute spontaneous intracerebral hemorrhage associated with antiplatelet therapy. This study demonstrated an increased risk of death or dependence at 3 months with platelet transfusion, and a higher rate of adverse events, with platelet transfusion. The Neurocritical Care Society recommends against platelet transfusion regardless of the type of platelet inhibitor, platelet function testing, hemorrhage volume, or neurologic examination. However, in patients who will undergo a neurosurgical procedure, it is reasonable to correct thrombocytopenia to maintain a level greater than 75,000. Additionally a single dose of desmopressin (DDAVP) in patients with antiplatelet-associated intracranial hemorrhage has been recommended (0.4 μg/kg IV), despite the lack of level 1 evidence in patients with intracranial hemorrhage supporting its use.
Severe coagulopathy has been demonstrated in approximately 30% of patients who sustain severe TBI. This is believed to occur due to the systemic release of tissue factor and brain phospholipids into the circulation, leading to disseminated intravascular coagulation and a consumptive coagulopathy. The process is exacerbated by acidosis, hypothermia, and excessive crystalloid administration. Correction of these metabolic abnormalities, along with replacement of fibrinogen and coagulation factors with cryoprecipitate and plasma or a PCC, is the only known treatment.
Critical care management
Intracranial Pressure Monitoring and Treatment
Elevated ICP is associated with increased mortality and worsened outcome after TBI. Clinical studies indicate that ICP is most highly elevated before surgical SDH evacuation, making empiric treatment of intracranial hypertension during the acute period crucial . In one study of 5 patients who were monitored before surgical hematoma evacuation, ICP ranged from 40 to 85 mm Hg and CPP ranged from 5 to 56 mm Hg before craniotomy. Craniotomy resulted in dramatic ICP reductions, and immediate improvement of cerebral blood flow (CBF) and jugular venous oxygen saturation, indicative of improved cerebral perfusion.
Clinical signs, such as decline in level of consciousness, unilaterally dilated or poorly responsive pupils, or extensor posturing, are signs that a patient is actively undergoing brainstem herniation and requires emergent management. Several techniques have been developed to abort signs and symptoms of herniation. The head of the bed should be elevated 30° to improve venous drainage, and care should be taken to ensure that there is no apparatus near or around the neck (such as a constrictive C-spine collar) blocking venous drainage. Hyperventilation lowers ICP by inducing vasoconstriction, although care should be taken to avoid excessive respiratory alkalosis, which may exacerbate secondary ischemia. In one randomized study, patients with TBI hyperventilated to a Pa co 2 of 25 mm Hg for 5 days had a worse clinical outcome than nonhyperventilated controls. Hyperventilation may be used to target a Pa co 2 of 30 mm Hg, and is best used for brief periods due to a wearing off effect that can occur. In a study of 75 patients with acute SDH studied with Xenon CBF, almost all had demonstrated reactive hyperemia postoperatively, which was related to ICP elevation and responsive to controlled hyperventilation.
Bolus osmolar therapy can be initiated with mannitol 1.0 to 1.5 g/kg and/or 30 to 120 mL of 23.4% hypertonic saline. In a study of 178 patients with acute SDH comparing low-dose and high-dose mannitol, the higher dose (1.4 g/kg) was associated with more frequent reversal of pupillary abnormalities, and lower ICP and better clinical outcome postoperatively. The osmotic gradient created by these agents favors efflux of interstitial and intracellular fluid across capillaries and into the circulation, thus reducing brain tissue water content and volume. There is no proven efficacy of targeting a particular sodium level (eg, sodium >155 mEq/L), and no evidence that hyperosmolar therapy loses its efficacy once a given serum osmolality level has been reached (eg, osmolality >320 mOsm/L). Highly elevated sodium levels (>165 mEq/L) have been associated with poor outcome, but this most likely reflects the severity of the primary injury, more than any particular harm caused by the hypernatremia itself. Because hypertonic saline increases intravascular volume and BP, it should be used in favor of mannitol in patients who are dehydrated, hypovolemic, or hypotensive. Conversely, hypertonic saline should be avoided in favor of mannitol in patients who are fluid overloaded or who have congestive heart failure.
In addition to its neuroprotective effects, hypothermia has been shown to decrease metabolic demand and ICP through reductions in cerebral blood volume and edema. A recent randomized controlled trial (EuroTherm 32–34) examining the effects of hypothermia (32–34°C) at the first sign of intracranial hypertension after TBI showed efficacy with regard to reduction of ICP and the need for barbiturate coma and decompressive hemicraniectomy, but not with regard to clinical outcome: in fact, outcomes were worse in patients who underwent systemic cooling. The reason for the worsening of outcome in the intervention arm was not apparent, however, because there was no marked increase in adverse events, such as infection or arrhythmia in the hypothermia group. An important criticism of this trial is that the intervention arm did not reflect standard practice, because hypothermia is typically reserved for cases of ICP refractory to cerebrospinal fluid drainage, sedation, CPP optimization, hyperventilation, and repeated doses of bolus osmotherapy. Based on these data, hypothermia cannot be recommended as a form of salvage therapy for elevated ICP after TBI.
Seizure Prophylaxis
Up to 24% of patients with traumatic SDH develop seizures either on presentation or postoperatively. Prophylactic antiepileptic drugs (AEDs) reduce the frequency of early seizures, which can increase metabolic demand and increase ICP. In the most widely cited trial, prophylactic phenytoin after TBI reduced the frequency of early seizures from 14% to 4%. An observational study found an even greater effect, with 2% of patients with TBI who received prophylactic anticonvulsant medications developing seizures, compared with 32% who did not.
In practice, phenytoin/fosphenytoin is frequently used because it can be loaded intravenously and does not cause significant sedation. Levetiracetam has recently become a popular alternative due to its more favorable adverse effect profile. In a randomized controlled trial and an observational study, there was no difference between phenytoin or levetiracetam in terms of reducing the rates of early seizures and adverse drug reactions.
The use of prophylactic AEDs does not reduce the risk of late seizures or posttraumatic epilepsy. Large-scale trials examining the optimal duration of seizure prophylaxis are lacking. AEDs are generally continued throughout the hospital stay, and in the absence of documented seizures, can be safely stopped before discharge, to avoid the deleterious effects that un-needed AEDs can have on neural recovery during rehabilitation.
Glucocorticoids
There is no limited evidence supporting the routine use of glucocorticoids in TBI. In fact, the use of glucocorticoid therapy was found to be harmful in large, prospective, randomized multicenter trials of patients with moderate to severe TBI.
Ventilatory Support
Hypercapnia and hypoxia can be detrimental in patients with neurologic emergencies; hence, early securement of the airway and optimal ventilation are crucial. Furthermore, mechanical ventilation allows for safer use of sedation. Postintubation sedation with propofol, midazolam, or dexmedetomidine has been found to help prevent neuroexcitation (ie, autonomic storming) and ventilator dyssynchrony. Lung protective ventilation strategies that use low tidal volume ventilation (4–8 mL/kg) and often permissive hypercapnia, should be used only when ICP is monitored to ensure that hypercapnia is not contributing to intracranial hypertension. Iatrogenic hyperventilation therapy (Pa co 2 ≤35 mm Hg) after TBI may compromise CPP at a time when CBF may already be critically educed. A small study examining the effects of varying levels of positive end-expiratory pressure (PEEP) in coma patients failed to show a uniform effect of higher levels of PEEP (10–15 cm H 2 O) on ICP or CPP. In another study, high PEEP (10–15 cm H 2 O) levels increased brain tissue oxygen tension (PbtO2) and oxygen saturation, without increase in ICP or decrease in CPP.
Little is known about the effect of prolonged mechanical ventilation in patients with SDH. One study reported that prolonged mechanical ventilation was associated with pulmonary complications, increased length of stay, and unfavorable discharge destination in patients. These complications were primarily seen in patients with history of alcohol abuse, low admission Glasgow Coma Score (GCS), and patients who required surgical evacuation.
Blood Pressure and Cerebral Perfusion Pressure Management
Normal cerebral autoregulation maintains an optimal CBF across a wide range of BP. It is estimated that 30% of patients with SDH in the setting of severe TBI have impaired cerebral autoregulation. Accordingly, avoidance of wide fluctuations in BP has been thought to be beneficial, because extremes of CPP can lead to increases in ICP ( Fig. 2 ) and worse clinical outcomes. Before evacuation, given the tendency of cerebral infarction to develop in the underlying brain, it seems wisest to avoid any kind of BP reduction. After hematoma evacuation, given the risk of postoperative bleeding into friable tissues and the empty subdural space, it has been suggested that lowering the systolic BPs to approximately 140 mm Hg may be beneficial, as is the case with hypertensive intracerebral hemorrhage (ICH), but this remains speculative.
In patients with ICP monitoring, BP management should be based on CPP targets. As a general rule, CPP should be maintained above 60 mm Hg in adults and in the range of 40 to 65 mm Hg in children. Efforts to optimize CPP should first always start with ICP reduction, if it is elevated. From the cardiac perspective, although acute SDH may be associated with electrocardiogram (ECG) abnormalities, myocardial injury and stunning are infrequent.
Analgosedation
Sedation and analgesia are integral to the management of acute SDH to minimize ventilator dyssynchrony and blunt sympathetic responses. Although this is largely beneficial, analgosedation can produce hypotension and potentially cerebral hypoperfusion. As a general rule, sedation always should conform to 3 basic principles. First, pain always should be treated first. Second, the level of sedation should be targeted to clinical response using scales such as the Richmond Sedation Agitation Scale or the Riker Sedation Agitation Scale. Third, sedation should be interrupted on a regular (at least daily) basis to assess neurologic status and to determine the minimum amount of sedation required moving forward. The only exception to the third rule is when dangerous agitation, refractory ICP, or severe hypoxia might result from a “wake-up” test.
Fentanyl and remifentanil are powerful opioid agents that are useful continuous infusion agents for establishing an adequate level of analgesia. Fentanyl is typically given at 25 to 200 μg/h. Remifentanil has an even faster onset and offset of sedation, making it ideal for neurologic checks, and is given in the range of 0.5 to 2.0 μg/kg per minute. Disadvantages of these opioid agents include hypotension, ileus, and opioid withdrawal after prolonged infusions.
Propofol is an attractive agent to use in acute SDH because of its rapid onset and offset, which makes intermittent neurologic evaluations easier. It can easily be added to a baseline fentanyl infusion to attain a given sedation goal. Propofol also suppresses cerebral metabolism and may lower ICP while also conferring some neuroprotective effects. Propofol is started at a rate of 5 μg/kg per minute (or 0.3 mg/kg per hour) and titrated by increments of increase by 5 to 10 μg/kg per minute (or 0.3–0.6 mg/kg per hour) until desired sedation level is achieved. Significant concerns when using propofol include respiratory suppression, hypotension, infections with prolonged infusions, and propofol infusion syndrome (PRIS). PRIS is a rare but potentially lethal complication seen more frequently with sepsis, serious cerebral injury, and the administration of high doses of propofol (usually doses >83 μg/kg per minute or >5 mg/kg per hour for >48 hours). It is associated with a high mortality rate (up to 33%) and is clinically characterized by circulatory collapse, metabolic acidosis, rhabdomyolysis or myoglobinuria with renal failure, dysrhythmias, and hyperkalemia. As a result, it is suggested that when used in TBI, the infusion rate of propofol not exceed 4 mg/kg per hour and that patients be monitored for ECG changes, lactic acidosis, and elevations in creatinine kinase and myoglobin. Because propofol is delivered in a lipid vehicle, some advocate adjusting dietary calories so as to avoid overfeeding.
Midazolam also is routinely used for inducing sedation in patients with TBI; it is a potent and short-acting benzodiazepine with sedative and amnestic, but not analgesic, effects. It is particularly useful as an add-on to fentanyl in patients with seizures or alcohol withdrawal. The advantages of midazolam are its rapid onset, easy titration, water solubility, and circumvention of the metabolic acidosis associated with the propylene glycol vehicle of other benzodiazepines, such as lorazepam. The major disadvantage of midazolam is the development of tachyphylaxis with prolonged infusions, and the potential for benzodiazepines to cause delirium. One meta-analysis of 4 studies showed that there are no important differences between propofol and midazolam when administered to provide sedation for patients with severe TBI. Dexmedetomidine also may be used as a primary agent or as an adjunct to opioids for sedation. It is a selective centrally acting alpha-2-agonist with anxiolytic, sedative, and some analgesic effects and no deleterious effects on respiratory drive. It is particularly effective for its sympatholytic effects in patients with TBI with autonomic storming. An initial loading dose is typically not required and a typical maintenance dose is 0.2 to 0.7 μg/kg per hour, with dosage increases no more frequently than every 30 minutes. Doses greater than 1.5 μg/kg per hour do not appear to add to the clinical efficacy of dexmedetomidine. Potential adverse effects of dexmedetomidine include bradycardia, hypotension, and nausea.
A meta-analysis of randomized controlled trials found no conclusive mortality benefit associated with the use of any particular analgosedative agent in patients with TBI.
Follow-up Imaging and Advanced Neuromonitoring
After hematoma evacuation, follow-up serial imaging is critical to ensure that recurrent hematoma formation is addressed promptly. Immediate postoperative imaging is important to establish a baseline, and subsequent imaging may be indicated with any deterioration in neurologic examination. Follow-up imaging within 1 to 2 weeks is also commonly pursued in otherwise asymptomatic patients to monitor for signs of progression or recurrence.
After surgical evacuation, management of patients with severe SDH who are comatose focuses on detection and management of neurologic complications. Multimodality neurologic monitoring has emerged as a promising tool in detecting subclinical deleterious physiologic changes by tracking oxygen delivery, CBF, and metabolism with the goal of improving the detection and management of secondary brain injury. Jugular venous oximetry (SjvO2) allows measurement of oxygen saturation in the venous blood exiting the brain. SjvO2 provides information on the global cerebral utilization of oxygen. Normal values range from 60% to 90%; an SjvO2 less than 50% represents cerebral ischemia and is associated with low CPP and worsened outcome, whereas SjvO2 values greater than 90% are indicative of hyperemia.
PbtO2 can be measured using intraparenchymal probes placed in white matter, at a depth of approximately 3 cm. Normal thresholds of PbtO2 range from 30 to 40 mm Hg, with values lower than 15 mm Hg being associated with poor outcome. One case series used oxygen supplementation to maintain PbtO2 higher than 25 mm Hg and found better outcomes compared with historical controls.
Cerebral microdialysis measures extracellular glucose, lactate, pyruvate, and glutamate. Lactate-to-pyruvate ratios are normally 40; ratios less than 20 indicate metabolic distress and enhanced anaerobic metabolism.
Invasive multimodality monitoring can improve real-time situational awareness of secondary brain injury, and allows intensivists to individualize management, including BP, temperature, and serum glucose levels.
Deep Vein Thrombosis Prophylaxis
Deep vein thrombosis (DVT) prophylaxis with low molecular weight heparin or unfractionated heparin should be initiated shortly after admission for SDH, as DVT and pulmonary embolism account for significant morbidity and mortality in bed-bound patients with stroke. Low molecular weight heparin is more effective than unfractionated heparin in preventing DVT. Although there are no randomized controlled trials analyzing the optimal time to start DVT prophylaxis in patients with hemorrhage, it is reasonable to start most patients at 24 to 48 hours after the initial event when an non-contrast CT scan head shows stability of the bleed.
Temperature Management
Extrapolating from the TBI and general critical care literature, normothermia should be the goal temperature for patients with acute SDH. Hyperthermia in patients with neurologic emergencies has been associated with increase in mortality in the first 24 hours after hospitalization, presumably by aggravating secondary brain injury. The evaluation of fever should include an infectious workup to rule out pulmonary and urinary tract infections as well as bacteremia. Central fever, defined as noninfectious temperature elevation due to disturbances in the central mechanisms of thermoregulation, should remain a diagnosis of exclusion. Once the workup and treatment of the underlying cause of fevers has been initiated, core body temperature should be reduced, either with direct cooling or pharmacologic means. Acetaminophen should be used first, followed by ibuprofen. Refractory fevers may require cooling devices for adequate control, which may necessitate active control of shiverin.
Blood Glucose Control
Avoiding severe hyperglycemia is reasonable in patients with SDH, as it is among all critically ill patients, and in general this can be achieved by using insulin sliding scales. It is reasonable to maintain glucose levels of 120 to 180 mg/dL in patients in the ICU. Microdialysis monitoring of patients with severe TBI has shown that critical brain tissue hypoglycemia can occur with tight glycemic control, however. In these patients, higher serum glucose targets, titrated to brain extracellular glucose levels, may be desirable.
Fluids and Nutrition
Several factors should be considered with regard to fluid composition and the optimal volume infused. Existing guidelines suggest targeting euvolemia using isotonic fluids such as 0.9% (normal) saline or a balanced solution such as Plasmalyte. Hypotonic fluids, such as half-normal saline or D5W, may cause water shifts across the blood–brain barrier (BBB), potentially exacerbating cerebral edema and ICP. Aggressive fluid administration aimed at hypervolemia is considered harmful due to the increased risk of lung injury and acute respiratory distress syndrome (ARDS), and should be avoided.
Adequate nutritional support should be instituted as soon as possible (eg, usually after 24–48 hours) because early enteral feeding after TBI has been shown to improve outcome compared with delayed feeding. A hypermetabolic, catabolic response may develop in patients with traumatic SDH leading to caloric requirements that are 50% to 100% higher than normal. Estimates of daily caloric requirements are important, because both underfeeding and overfeeding are associated with complications.
Stress Ulcer Prophylaxis
Gastrointestinal (GI) hemorrhage is a serious complication and potential cause of mortality in critically ill neurologic patients. Although the frequency of stress ulcer–related GI bleeding is relatively low, patients at risk for GI bleeds include those on a mechanical ventilator, patients who are comatose, and patients with coagulopathy or a previous history of GI bleeding. In one study of 51 patients with intracranial hemorrhage that included patients with SDH, the size of the hematoma, development of septicemia, and lower GCS scores were found to be predictors of GI hemorrhages. Although the optimal agent for acid suppression is unknown, both H2 receptor blockers and proton pump inhibitors are considered agents of choice. For critically ill patients who are able to receive enteral medications, oral proton pump inhibitors are preferred over histamine-2 receptor antagonists, sucralfate, or antacids. In cases in which enteral medications are contraindicated, an IV histamine-2 receptor antagonist or IV proton pump inhibitor can be administered.
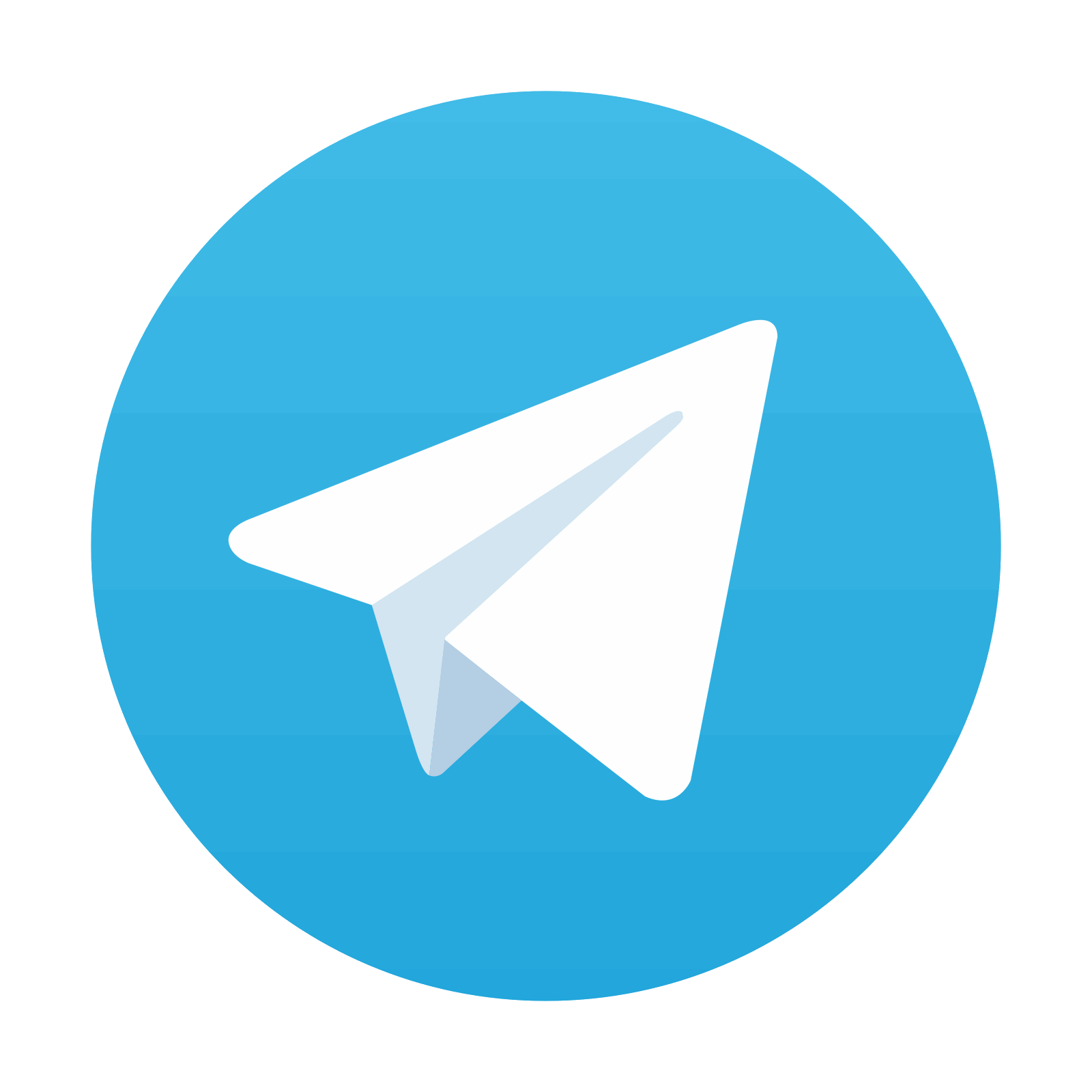
Stay updated, free articles. Join our Telegram channel

Full access? Get Clinical Tree
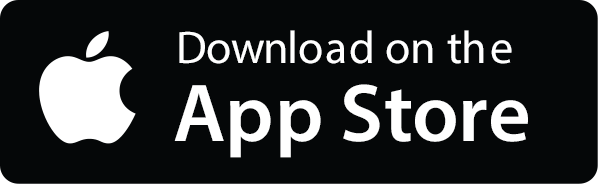
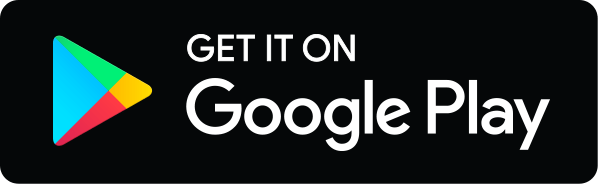