Neurodevelopment
Karl Zilles
Neural induction
The central nervous system originates from the midline region of the embryo as a specialized area of ectoderm, the neuroectoderm, or neural plate. FGF (fibroblast growth factor) signalling and BMP (bone morphogenetic protein) as well as Wnt (wingless gene) inhibition are required as steps for neural induction.(1) However, the complete set of factors and their respective interactions are presently not sufficiently understood in the mammalian embryo. As the neuroectodermal cells proliferate the neural plate is transformed into an indentation, the neural groove. The lateral parts of this groove approach each other and join in the midline, forming the neural tube. The folds begin to fuse in the central part of the groove but the most rostral and caudal parts close only later, leaving initially rostral and caudal neuropores. A small transitional zone between the neural plate and the surrounding ectoderm provides the cells of the neural crest, which develop into the postganglionic cells of the sympathetic and parasympathetic nervous system, the sensory neurons of the spinal ganglia and ganglia of cranial nerves, Schwann cells, and chromaffine cells of the suprarenal glands.
Neural tube formation requires a controlled expression of cell adhesion molecules in the lateral folds of the neural groove. If the rostral neuropore fails to close, the development of the forebrain is impaired leading to anencephaly. If the caudal neuropore fails to close, the most severe result is rachischisis, a malformation with a dorsally exposed neural groove. The mildest result is spina bifida occulta which is a cleft of a vertebral arch covered by epidermis.
As development continues, the neural tube and crest move to a position between the ectoderm and the notochord. The rostral part of the neural tube differentiates into the brain; the caudal part (behind the fifth somite) differentiates into the spinal cord.
Organogenesis of the central nervous system
The embryonic brain has three vesicular enlargements: the forebrain, telencephalon and diencephalon, the midbrain, mesencephalon, and the hindbrain, rhombencephalon. Because the brain grows much faster than the rest of the embryo, it becomes deflected ventrally. A dorsally convex cephalic flexure marks the border between hindbrain and midbrain, and a cervical flexure marks the border between spinal cord and hindbrain (Fig. 2.3.2.1). The ventrally convex pontine flexure forms the hindbrain. During week 5, the forebrain differentiates further into the rostral telencephalon and the more caudal diencephalon. The telencephalon consists of two
hemispheric vesicles connected by a thin lamina terminalis, the most dorsal part of which develops into the commissural plate, the Anlage of the corpus callosum. The ventral part of the lamina terminalis differentiates into the anterior commissure. The central cavity of the diencephalon (the third ventricle) is connected with the cavities of the hemispheric vesicles (the lateral ventricles) by the interventricular foramen. The diencephalon develops bilateral evaginations, the eye vesicles, which differentiate into the retina and the optic nerve. Meanwhile the hindbrain becomes subdivided into a rostral metencephalon and a caudal myelencephalon.
hemispheric vesicles connected by a thin lamina terminalis, the most dorsal part of which develops into the commissural plate, the Anlage of the corpus callosum. The ventral part of the lamina terminalis differentiates into the anterior commissure. The central cavity of the diencephalon (the third ventricle) is connected with the cavities of the hemispheric vesicles (the lateral ventricles) by the interventricular foramen. The diencephalon develops bilateral evaginations, the eye vesicles, which differentiate into the retina and the optic nerve. Meanwhile the hindbrain becomes subdivided into a rostral metencephalon and a caudal myelencephalon.
The cerebellum starts to develop from the metencephalon during week 6 (Fig. 2.3.2.1). At first, the enlarged central cavity of the neural tube (the future fourth ventricle) has a thin roof plate bordered by two thickenings of the neural tube, the rhomboid lips, which merge in the midline. These thickenings develop into the cerebellar hemispheres, while the midline part develops into the vermis of the cerebellum. Fissures appear in the cerebellar hemispheres, forming the anterior and posterior lobes and the uvula.
The rhombencephalon is temporarily divided into eight rhombomeres,(2) whose borders are specified by specific combinations of transcription factors (e.g. Hox, Krox, Wnt genes) disappear during further development. Local expression of homeobox genes leads to the formation of the pallium and the ganglionic hill in the forebrain.
The hindbrain develops in close association with the visceral archs, which appear during week 4. It innervates these archs and the organs derived from them by a group of branchial nerves, which later become the trigeminal (V), facial (VII), glossopharyngeal (IX), vagal (X), and accessory (XI) cranial nerves. Other cranial nerves develop connections between the hindbrain and peripheral organs not derived from the visceral archs. They are the oculomotor (III), trochlear (IV), abducens (VI), vestibulocochlear (VIII), and hypoglossal (XII) nerves. The olfactory (I) and optic (II) nerves arise separately as evaginations of the forebrain.
Histogenesis of the spinal cord
The neural tube initially consists of a single layer of neuroepithelial cells surrounding a central canal filled with the cerebrospinal fluid. The outer surface of the future spinal cord has an external limiting membrane, and the inner surface bordering the central canal has an inner limiting membrane. The entire wall of the early neural tube is called the ventricular zone.(3)
The cells of the ventricular zone proliferate, and the surface of the spinal cord enlarges. The cord then thickens as cells divide further to produce a multilayered epithelium. The daughter cells have different potentialities: one type of cell (the neuroblast) retains the capability for mitosis, whereas another type (the proneurone) is postmitotic and represents an immature neurone. The proliferation of neurones is almost complete around birth.
Some neuroepithelial cells develop into precursors of glial cells, glioblasts, which differentiate into astroglial, oligodendroglial, and microglial cells. The first glioblasts differentiate into radially extended cells spanning the entire width of the wall of the spinal cord (the same occurs in the cerebral hemispheres and the cerebellar cortex as described below). During later development these cells are transformed into ependymal cells and astroglia.
The histogenesis of the spinal cord starts at the cervical level and progresses in a caudal direction. After week 3, a longitudinal sulcus limitans is recognizable on the inner surface of the neural tube, dividing the wall into dorsal (alar) and ventral (basal) plates. The dorsal plates of both sides are connected by a thin roof plate, and the ventral plates by a thin floor plate. The dorsal plate differentiates into a sensory zone, the dorsal horn of the adult spinal cord, and the ventral plate differentiates into a motor zone, the ventral horn. The sympathetic preganglionic neurones form the lateral horn, which is present only at thoracic levels. The subdivision into dorsal and ventral plates is functionally important not only in the spinal cord, but also in the brainstem (see below).
During week 5, three concentrically organized zones—a cell-dense ventricular zone, a less-dense intermediate or mantle zone, and a superficially located marginal zone free of neuronal cells— develop in the wall of the spinal cord. Proneurones leave the ventricular zone and migrate along radial glial cells into the intermediate zone where they become organized into cell groups, nuclei. The motor neurones develop the axons of the ventral root, and the processes from spinal ganglionic cells grow into the spinal cord to
form the dorsal roots. Synapses develop first in the motor zone, and later in the sensory zone, during the weeks 10 to 13.
form the dorsal roots. Synapses develop first in the motor zone, and later in the sensory zone, during the weeks 10 to 13.
During the third month, the ventricular zone is reduced to a small rim surrounding the central canal and is finally transformed into the ependymal cell layer. The intermediate zone becomes organized into dorsal, ventral, and (at thoracic levels) lateral horns. The ascending and descending fibre tracts of the spinal cord are increased in size in the marginal zone. During weeks 14 and 15, oligodendrocytes begin to myelinate these fibre tracts. The corticospinal, or pyramidal, tract is visible for the first time during week 14 and reaches its target neurones, mainly motor neurones of the ventral horn, between weeks 17 and 29. The myelination of the pyramidal tract is completed between the first and second postnatal years. This late myelination explains the presence of the Babinski reflex in newborns and its disappearance during the first 2 years.
Histogenesis of the brainstem and cerebellum
At the level of the fourth ventricle the various zones of the hindbrain are arranged in a lateral-to-medial sequence (somatosensory-viscerosensory-visceromotor-somatomotor). In the hindbrain, proneurones not only migrate radially, as in the spinal cord, but also tangentially and longitudinally. This complex migration and the growth of fibre tracts lead to changes of the lateral-to-medial sequence of cranial nerve nuclei (e.g. the facial nucleus) in the adult.
In the cerebellum, between weeks 10 and 11, neuroblasts migrate from the ventricular zone through the intermediate zone into an area (the external granular layer) at the surface of the marginal zone. During weeks 12 and 13, proneurones from the ventricular zone begin to migrate along radially extended glial cells, Bergmann glia, into a region below this external granular layer, where they form the Purkinje cells of the ganglionic layer of the cerebellar cortex. Other proneurones from the ventricular zone develop into the cerebellar nuclei. Proneurones from the external granular layer then migrate inwards to form the internal granular layer and the basket and star cells of the molecular layer. The migration of cerebellar proneurones is not completed until the first postnatal year. During weeks 16 and 26, synapses develop and afferent fibre systems begin to form. The external granular layer finally disappears during the first 2 years of life, leaving the three-layered organization (molecular, ganglionic, and internal granular layers) of the adult cerebellar cortex.
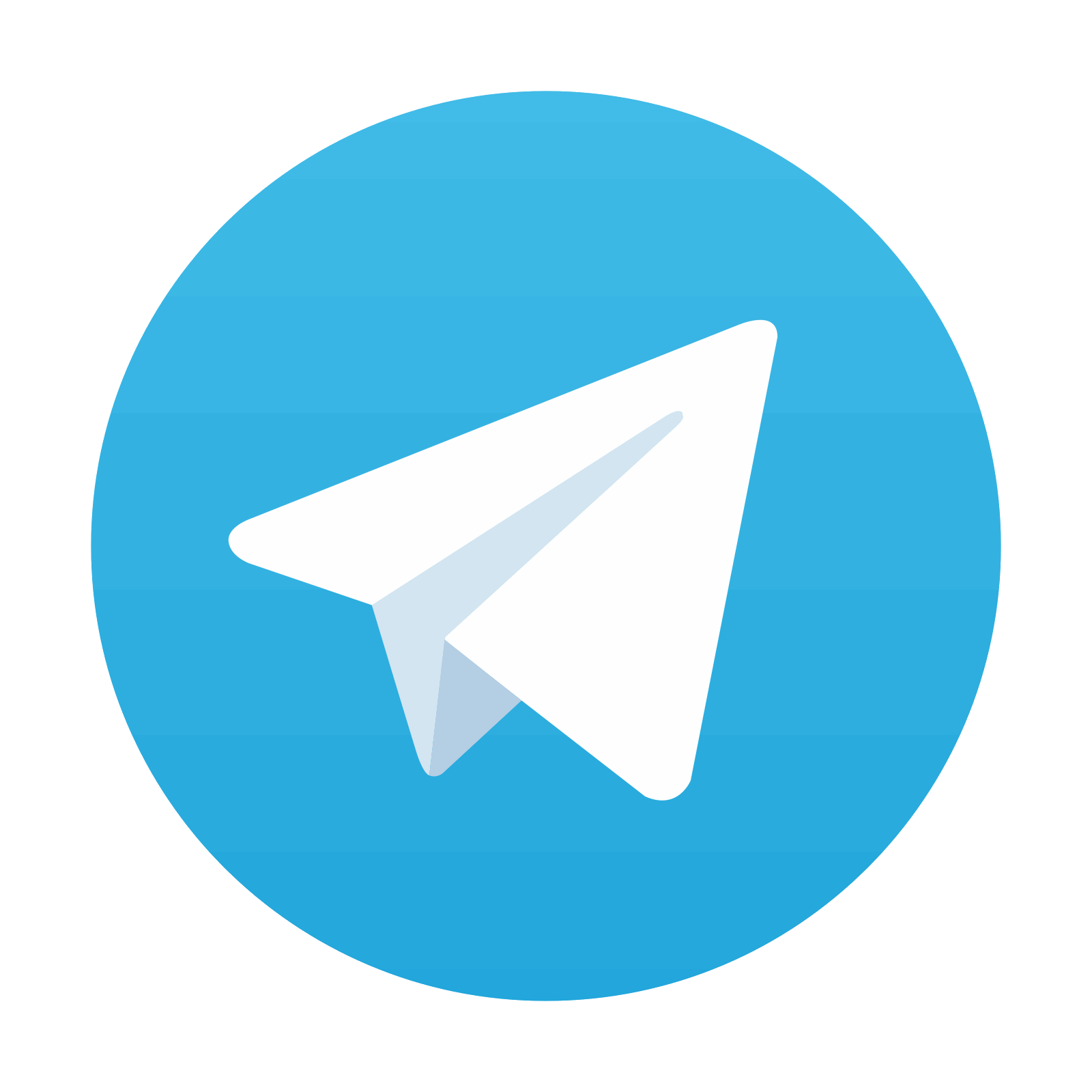
Stay updated, free articles. Join our Telegram channel

Full access? Get Clinical Tree
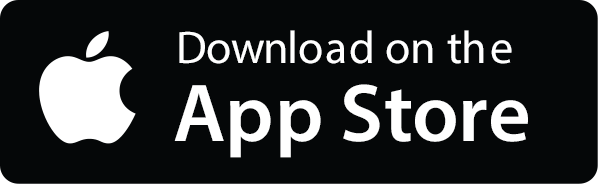
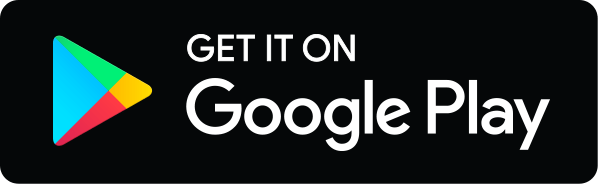