Neuroendocrinology
Charles B. Nemeroff
Gretchen N. Neigh
Definitions and principles
In the late 1950s, a controversy arose in endocrinology and neuroscience concerning whether neurones are capable of manufacturing and secreting hormones; that is to say, is it possible that certain neurones subserve endocrine functions? Much of the ensuing debate, which persisted for approximately two decades, was centred on two major findings. First, led by a husband and wife team, the Scharrers, a number of neurohistologists working with mammalian as well as with lower vertebrate and invertebrate species documented the presence—by both light and electron microscopy—of neurones that had all the characteristics of previously studied endocrine cells. They stained positive with the Gomori stain, which is claimed to be specific to endocrine tissues, and they incorporated granules or vesicles containing the endocrine substance purportedly released by these cells. The second major avenue of investigation centred around the brain’s control of the secretion of the pituitary trophic hormones, which in turn were long known to control the secretion of the peripheral target endocrine hormones such as thyroid hormones, gonadal steroids, adrenal steroids, etc. A critical observation by several investigators had earlier demonstrated the existence of a vital neuroendocrine system, namely the magnocellular cells of the paraventricular nucleus of the hypothalamus that synthesized vasopressin and oxytocin, the nonapeptides that are transported down the axon to the nerve terminals of these neurones in the posterior pituitary (neurohypophysis) and released in response to physiological stimuli. For example, the release of vasopressin, or the antidiuretic hormone, as it is commonly named, acts as a critical regulator of fluid balance, and oxytocin is known to regulate the milk-letdown reflex during breast feeding.
It is now firmly established that neurones are indeed capable of functioning as true endocrine tissues, synthesizing and releasing substances, known as (neuro)hormones, which are released directly into the circulatory system and transported to distant sites of action. The release of vasopressin from the posterior pituitary gland and its action on the kidney is one often cited example; the action of the hypothalamic release and release-inhibiting factors on the anterior pituitary trophic hormone-producing cells is another.
Pleiotropic roles—endocrine factors and neurotransmitters
Although, it was clearly important to document the ability of neurones to function as neuroendocrine cells, particularly those in the central nervous system (CNS), the focus on an artificial classification system with clear demarcations of endocrine versus neuronal versus neuroendocrine, quickly lost its heuristic value. Indeed, we now recognize that the very same substance, often function at one site as an endocrine substance and at another as a neurotransmitter. Thus, adrenaline (epinephrine) functions as a hormone in the adrenal medulla and as a conventional neurotransmitter substance in the mammalian CNS. Similarly corticotrophin-releasing factor (CRF) functions as a true hormone in its role as a hypothalamic hypophysiotrophic factor in the hypothalamic anterior pituitary complex, yet it is apparently a ‘conventional’ neurotransmitter in cortical and limbic brain areas. It may act as a paracrine substance in the adrenal medulla (see Fig. 2.3.3.1). Thus, the field has progressed to the stage where we now strive to characterize the role of a particular chemical messenger in a particular region or endocrine axis. The traditional endocrine and neurotransmitter roles for several peptides alluded to above are firmly established, but the equally important paracrine roles for such substances, namely the secretion of a substance from one cell where it acts upon nearby cells, remain largely unexplored. This is, perhaps, best illustrated in the gastrointestinal tract where several peptides that function as hormones or neurotransmitter substances in other sites, including the CNS, act to influence local cellular function. Examples would include vasoactive intestinal peptide, cholecystokinin, and somatostatin.
Neuroendocrinology
Neuroendocrinology thus, comprises the study of the endocrine role of neuronal or glial cells as well as the neural regulation of endocrine secretion, with a major portion of the latter consisting of the biology of the various hypothalamic-pituitary-end-organ axes and the major neurohypophyseal hormones, vasopressin and oxytocin. Because of the elegant and precise regulation of peripheral endocrine hormone secretion, afforded in part by the feedback of peripherally secreted hormones at pituitary and a variety of CNS sites, the actions of such hormones on the brain has become an integral part of this discipline. The related discipline of psychoneuroendocrinology arose with the realization that there are binding sites (receptors) for peripheral hormones within the CNS that have little to do with the feedback regulation of the hypothalamicpituitary-end-organ axes, and the further recognition of the seminal role of the CNS in regulating endocrine function, for example in the effect of stress on several such measures. Often cited as beginning with the pioneering observations of Berthold, who reported that removing roosters’ testosterone-secreting gonads abolished their sexual behaviour, psychoneuroendocrinology has been expanded to include the effects of hormones on behaviour, as well as the study of endocrine alterations in psychiatric disorders and, the converse, psychiatric symptomatology in endocrine disorders. Indeed, this stepchild of neuroendocrinology and psychosomatic medicine has been one of the most rapidly growing areas of research in psychiatry, now boasting an international society (International Society of Psychoneuroendocrinology), an annual meeting, and its own journal (Psychoneuroendocrinology).
Neuroendocrine window
One of the most commonly used strategies in the 1970s and 1980s, still in occasional use today, is the so-called neuroendocrine ‘window’ strategy. Until the relatively recent development and availability of functional brain-imaging techniques, the brain remained relatively inaccessible for study, with the exception of cerebrospinal fluid (CSF) and postmortem tissue studies. With the emergence of the monoamine theories of mood disorders and schizophrenia, many investigators attempted to draw conclusions about the activity of noradrenergic, serotonergic, and dopaminergic circuits in patients with various psychiatric disorders by measuring the basal and stimulated secretion of pituitary and end-organ hormones in plasma. There is little doubt that such an approach has severe limitations, but the results, now coupled with more modern approaches, have contributed to the substantial progress made in elucidating the pathophysiology of mood and anxiety disorders and, to a considerably lesser extent, schizophrenia. One assumption of the neuroendocrine window strategy is that the monoamine-containing neurones that regulate endocrine secretion are disordered (or not disordered) to the same extent as those monoamine circuits posited to be involved in the pathophysiology of the disorder under study. Such an assumption may well be true in neural circuits in which the cells of origin are found in a circumscribed area and project to widely diffused areas of the CNS (for example, the serotonergic and noradrenergic projections to the forebrain from the raphe and locus coeruleus cells in the brainstem, respectively). In contrast are the various dopaminergic circuits in the CNS, with their well-known topographic point-to-point
distribution. Thus, there is little reason to believe that the activity of the major dopamine-containing hypothalamic projection, the tuberoinfundibular system, with perikarya in the arcuate and periventricular hypothalamic nuclei and projections to the median eminence, is in any way related to the activity of the mesolimbicocortical dopamine pathway, with cell bodies in the ventral tegmentum of the midbrain and projections to the nucleus accumbens, amygdala, and cortical regions. This latter pathway has been implicated in the pathophysiology of schizophrenia. Thus, the study of the dopamine modulation of prolactin secretion in schizophrenia is unlikely to inform about the nature of limbic and cortical dopamine neuronal alterations in this devastating disorder.
distribution. Thus, there is little reason to believe that the activity of the major dopamine-containing hypothalamic projection, the tuberoinfundibular system, with perikarya in the arcuate and periventricular hypothalamic nuclei and projections to the median eminence, is in any way related to the activity of the mesolimbicocortical dopamine pathway, with cell bodies in the ventral tegmentum of the midbrain and projections to the nucleus accumbens, amygdala, and cortical regions. This latter pathway has been implicated in the pathophysiology of schizophrenia. Thus, the study of the dopamine modulation of prolactin secretion in schizophrenia is unlikely to inform about the nature of limbic and cortical dopamine neuronal alterations in this devastating disorder.
Hypothalamic-pituitary-end-organ axes
Because a large portion of neuroendocrinology relevant to psychiatry is concerned with the hypothalamic-pituitary-end-organ axes, alterations in each of these systems in patients with a major psychiatric disorder are described later in this chapter. To avoid repetition, a generic description of the hierarchical organization of the various components of these systems is briefly outlined here. More comprehensive reviews of this subject are available.(1) In general, the hypothalamus contains neurones that synthesize release and release-inhibiting factors. These peptide hormones, summarized in Fig. 2.3.3.1, are synthesized by a process beginning with transcription of the DNA sequence for the peptide prohormone. After translation in the endoplasmic reticulum and processing during axonal transport and packaging in the vesicles in the nerve terminals, the now biologically active peptide is released from nerve terminals in the median eminence and secreted into the primary plexus of the hypothalamohypophyseal portal vessels. They are transported humorally to the sinusoids of the adenohypophysis where they act on specific membrane receptors on their specific target: the pituitary trophic hormone-producing cells. Activation of their receptors results in the release or inhibition of release of the pituitary trophic hormone. The increase or decrease in pituitary trophic hormone secretion produces a corresponding increase or decrease in end-organ hormone secretion. Thus gonadotrophin-releasing hormone (GnRH), a decapeptide, induces the release of the gonadotrophins, luteinizing hormone, and follicle-stimulating hormone from the anterior pituitary gland, which in turn stimulate the secretion of oestrogen and progesterone in women, and testosterone in men. The exogenous, intravenous administration of GnRH, comprises the GnRH stimulation test, a very sensitive test of hypothalamic-pituitary-gonadal (HPG) axis activity. Such stimulation tests are thought to be a sensitive measure of the activity of the axis because it is influenced by GnRH secretion, gonadotrophin secretion, and feedback at the pituitary and brain by gonadal steroids. The organization of, and major feedback mechanisms thus far demonstrated in the hypothalamic-anterior pituitary-end-organ axes are illustrated in Fig. 2.3.3.2.
![]() Fig. 2.3.3.2 Relationships between brain neurotransmitter systems, hypothalamic peptidergic (releasingfactor) neurones, anterior pituitary, and peripheral endocrine organs, illustrating established feedback loops: LHRH, luteinizing-hormone releasing hormone; SRIF, somatostatin; MIF, melanotrophin release-inhibiting factor; GHRF, growth-hormone releasing factor. Other abbreviations as in Fig. 2.3.3.1. |
In summary, neuroendocrinology (and the related discipline psychoneuroendocrinology) broadly encompasses the study of the following:
the neural regulation of peripheral, target-organ hormone secretions, pituitary trophic hormone secretions, and secretions of the hypothalamic-hypophysiotrophic hormones;
the role of neurotransmitter systems in the regulation of the above;
the hormone effects of each of the endocrine axes on the CNS, alterations in the activity of the various endocrine axes in major psychiatric disorders, and conversely the behavioural consequences of endocrinopathies;
the effects of target gland hormones on the CNS in normal individuals—for instance, the effects of synthetic glucocorticoids on memory processes.
The hypothalamic-pituitary-thyroid axis
It has been recognized for more than a century that adult patients with hypothyroidism exhibit profound disturbances in CNS function, including cognitive impairment and depression. In more recent years, attention has focused on more subtle alterations of the hypothalamic-pituitary-thyroid (HPT) axis in depressed patients. Hypothyroidism is most frequently subclassified as in four grades as follows:
Grade 1 hypothyroidism is classic primary hypothyroidism (increased thyroid-stimulating hormone (TSH), decreased peripheral thyroid hormone concentrations, and an increased TSH response to thyrotrophin-releasing hormone (TRH)).
Grade 2 hypothyroidism is characterized by normal, basal thyroid-hormone concentrations, but an increase in basal TSH concentrations and an exaggerated TSH response to TRH.
Grade 3 hypothyroidism can only be detected by a TRHstimulation test; patients have a normal basal thyroid hormone and TSH concentrations, but an exaggerated TSH response to TRH.
Grade 4 hypothyroidism is defined as normal findings on the three thyroid axis function tests noted above, but the patients have antithyroid antibodies.
Left untreated, most, if not all, patients’ progress from grade 4 to grade 1 hypothyroidism. Several studies have revealed an inordinately high rate of HPT axis dysfunction, largely hypothyroidism, in patients with major depression. In our pilot study, patients with comorbid depression and anxiety were especially likely to exhibit HPT axis abnormalities, especially the presence of grade 4 hypothyroidism, i.e. symptomless autoimmune thyroiditis. Patients with other major psychiatric diagnoses including schizophrenia and anxiety disorders appear to exhibit normal HPT axis function. For patients who require thyroid hormone replacement secondary to thyroid ablation, Bunevicius et al.(2) recently reported that treatment with a combination of tri-iodothyronine (T3) and thyroxine (T4) is optimal for mood and cognitive function, rather than the standard medication of T4 alone.
In addition, a blunted TSH response to TRH is observed in approximately 25 per cent of patients with major depression. This observation, first reported by Prange et al.(3) and Kastin et al.,(4) more than 25 years ago, has been replicated in many studies. Unfortunately, the pathophysiological underpinnings of this observation remain obscure, though there is considerable evidence that it may be due, at least in part, to chronic hypersecretion of TRH and subsequent TRH-receptor downregulation in the anterior pituitary gland. Indeed, our group(5) and others have reported elevated TRH concentrations in the CSF of drug-free depressed patients.
TRH was the first of the hypothalamic-releasing factors to be chemically characterized. Immunohistochemical and radio-immunoassay methods revealed a heterogeneous brain distribution of TRH. This was the first in a series of experimental results that led to the inexorable conclusion that this peptide, and, as discussed below, other release and release-inhibiting hormones, function in extrahypothalamic brain regions as neurotransmitter substances. Thus, TRH has been shown to produce direct brain effects, independent of its action on the pituitary thyrotrophs. Antidepressant effects of intravenously and intrathecally applied TRH have been reported,(3) but the results have not been confirmed in large, controlled clinical trials.(6) In contrast, several reports over the last 30 years have documented the efficacy of T3 (25-50 mg daily) in both accelerating the rate of onset of antidepressant response,(7) and in converting antidepressant non responders to responders,(8) the later confirmed in the recent NIMH-funded STAR-D trial.(9) Studies also suggest that T4 (100-300 µg daily) supplementation is a viable strategy for increasing the response to antidepressants.(10) These studies were initiated when it became apparent that patients (and laboratory animals) with hypothyroidism do not respond to antidepressant agents. This led to the hypothesis that patients with subtle forms of hypothyroidism (grades 2-4) may not respond optimally to antidepressants unless they are adequately treated with exogenous thyroid hormone. With the cloning of the thyroid hormone receptor and its localization within the CNS, studies of its regulation in depression, as well as the regulation of TRH biosynthesis, can now be conducted in postmortem brain tissue.
The hypothalamic-pituitary-adrenal axis
Although, first identified in crude form in 1955 by Saffran et al.,(11) corticotrophin-releasing factor (CRF) was not chemically identified until 1981 by Vale and colleagues.(12) This discovery finally permitted the comprehensive assessment of hypothalamicpituitary-adrenal (HPA) axis activity, and also led to scrutiny of the role of this peptide, which is now known to co-ordinate the endocrine, immune, autonomic, and behavioural effects of stress in a variety of psychiatric disorders.
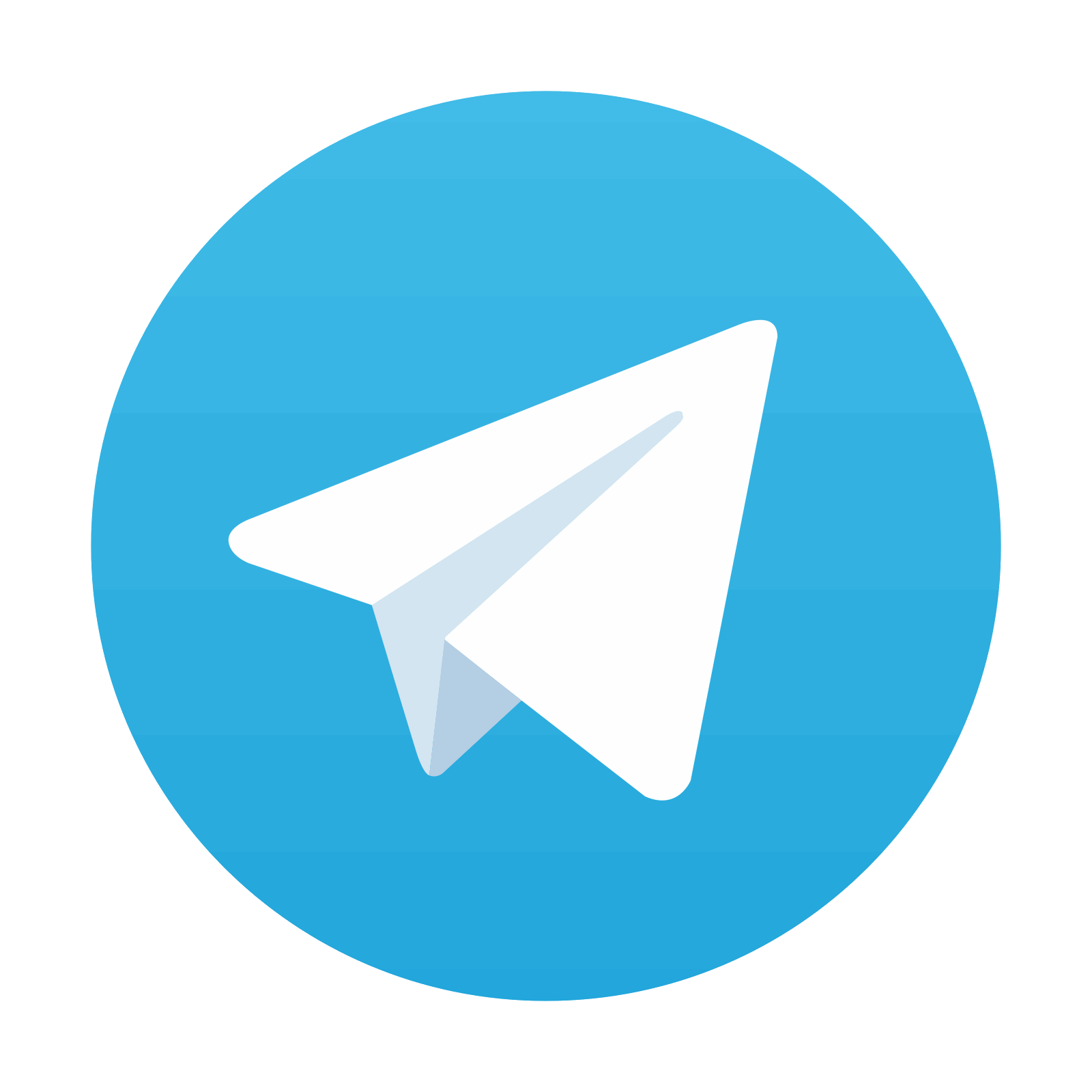
Stay updated, free articles. Join our Telegram channel

Full access? Get Clinical Tree
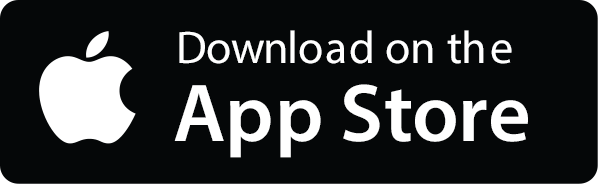
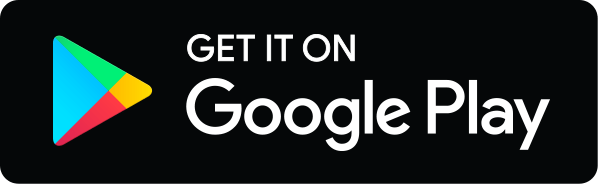