Potentially, any irritant to the abdominal wall and viscera such as
Ulcer
Cholecystitis
Pancreatitis
Hepatitis
Masses
Gastroenteritis
Hemorrhage
Constipation
Bowel obstruction
Superior mesenteric artery syndrome
Tight clothing or materials over the abdomen
Abdominal wall wound infection
Malfunctioning ostomies, tubes, and drains within the abdominal wall
GI interventional procedures
This chapter reviews the anatomy, physiology, function, and neural control of the entire gastrointestinal tract and the specific derangements encountered following SCI. The limited preclinical data following experimental SCI is discussed throughout with particular emphasis on identifying both evidence-based therapies and areas for focused research.
16.2 Background
16.2.1 Gastrointestinal Anatomy
The gastrointestinal tract of vertebrates is the culmination of a complex and sophisticated evolutionary process [15, 16]. At its most elemental level, the vertebrate alimentary canal consists of an epithelial layer enclosed by an innervated muscle layer and organized along an oral to anal arrangement. The so-called through-gut system of vertebrates, however, is more than a mere tube absorbing and propelling nutrients along a longitudinal gradient. Specifically, the gastrointestinal tissue consists of a laminar organization of five distinct layers, each of which plays a vital role in digestion, absorption, and propulsion (Fig. 16.1).


Fig. 16.1
Proceeding along the apical to basolateral direction, gastrointestinal tissue layers consist of mucosa (the tissue in direct contact with the luminal contents), submucosa, circular smooth muscle, longitudinal smooth muscle, and serosa. In addition, the submucosal layer is invested with the submucosal (Meissner’s) plexus, whereas the myenteric (Auerbach’s) plexus innervates the circular and longitudinal muscle layers. Combined, these plexuses provide the local neural control of the gut and are generally conserved across mammalian species
Regional specialization dominates the entire length of the gastrointestinal tract such that differentiated functions are performed along the proximal-distal path. The principal organization of oropharynx, esophagus, stomach, small intestine, and colon is present in monogastric species such as humans and rodents, the primary subjects discussed in this review. The digestive processes of the gastrointestinal tract are augmented by the liver, gall bladder, and pancreas. In non-Western medicine, the spleen is also considered to play an essential accessory role in digestive functions.
16.2.2 Overview of Gastrointestinal Neural Regulation
The principal nutritive functions of the gastrointestinal tract center on the propulsion, digestion, and absorption of nutrients and the maintenance of proper fluid balance. Each is critically dependent upon a hierarchy of enteric, parasympathetic, and sympathetic neural control. In addition, the gastrointestinal tract is the largest endocrine organ in the body, secreting enteric peptides to regulate local gastrointestinal homeostasis as well as regulating organism homeostasis through effects on the brain. The projections and the extent to which each of these complex neural circuits contribute to these processes varies as does the susceptibility to the effects of spinal cord injury.
16.2.2.1 Enteric Innervation
As previously mentioned (Fig. 16.1), the gastrointestinal tract possesses intrinsic innervation in the form of the submucosal and myenteric plexuses that contain the neural circuitry capable of independent reflex function and quasi-autonomous control of the gastrointestinal tract. The extent, and sophistication, of the enteric nervous system has long been evident in the reflexive control that remains even when the gastrointestinal tissues are isolated from extrinsic inputs [17]. The enteric nervous system mediates digestion through localized control over the specialized cells and individual reflex systems for the smooth musculature, secretory glands, and microvasculature of much of the digestive tract (reviewed in [18]). Briefly, functional units of the enteric nervous system are formed by a polysynaptic circuit consisting of sensory neurons, interneurons, and motoneurons, all of which serve in a manner that is similar to many reflexive or integrative neural circuits [19]. For example, sensory neurons (commonly termed intrinsic primary afferent neurons) consist of neurons that respond to either specific chemical cues, mechanical distortion of the mucosa, or distortion of sensory processes embedded within the muscle layers. These afferent neurons terminate onto excitatory or inhibitory interneurons that project in distinct aboral or oral directions. In turn, these interneurons terminate on excitatory or inhibitory motoneurons innervating the smooth musculature. This example illustrates how the enteric nervous system contains the reflex motor programming necessary for propulsive movement as well as retropulsive movement of intestinal contents and segmentation (reviewed in [20]). Of particular relevance to the paced, quasi-autonomous activity of the smooth muscles is the recent interest in the role the interstitial cells of Cajal play in examples of gastrointestinal health and disease [21–23].
Limitations regarding the cross species interpretation of the neurochemical coding of the enteric nervous system have been noted [24, 25]. However, the classical neurotransmitters acetylcholine and serotonin are perhaps the most predominant across species (reviewed in [25, 26]). The gaseous mediator, nitric oxide, is also highly conserved across species (reviewed in [26]). Beyond these neurochemical phenotypes, vasoactive intestinal peptide [27], substance P [28], enkephalins [29], γ-aminobutyric acid (GABA, [30]), and numerous others have also been identified (reviewed in [31]). Evidence is emerging that carbon monoxide and hydrogen sulfide are additional gaseous mediators besides nitric oxide that serve important signaling functions in gastrointestinal physiology [32].
16.2.2.2 Parasympathetic Innervation
Significant parasympathetic innervation of the gastrointestinal tract originates from the brainstem, via the vagus nerve (Fig. 16.2). While vago-vagal reflex circuits modulate digestive processes from the oral cavity to the transverse colon, the level of vagal control diminishes caudally [33–36]. It is generally accepted that vagal input terminates at approximately the second segment (the proximal 2/3) of the transverse colon and the portions of the gastrointestinal tract comprising the distal transverse colon receive parasympathetic input from the sacral spinal cord, via the sacral roots. These parasympathetic fibers distribute to the gastrointestinal tract through the pelvic nerve.


Fig. 16.2
Schematic depiction of the sympathetic and parasympathetic innervation of the gastrointestinal tract. CeG celiac ganglion, SMG superior mesenteric ganglion, IMG inferior mesenteric ganglion
Acetylcholine is the ubiquitous neurotransmitter of the parasympathetic preganglionic neurons and acts through binding to nicotinic receptors [37]. The neurochemical phenotype of the parasympathetic postganglionic neurons is distributed across cholinergic neurotransmission that acts through binding to muscarinic receptors [38] and non-adrenergic, non-cholinergic phenotypes [39–42].
16.2.2.3 Sympathetic Innervation
Preganglionic sympathetic innervation of the gastrointestinal tract originates from the intermediolateral cell column (lateral horn) of the spinal cord between the 5th and 12th spinal thoracic segments (Fig. 16.2). In turn, the sympathetic axons terminate throughout the paravertebral sympathetic chain or form the thoracic splanchnic nerves terminating on postganglionic neurons within either the celiac or superior mesenteric ganglia. The former innervates nearly the entire length of the gastrointestinal tract, from the stomach to the ileum, while the superior mesenteric ganglion projects to the ascending and transverse colon. In addition, sympathetic nerve fibers to the splenic flexure and rectum reside within the 1st through 3rd lumbar segments of the spinal cord. These fibers extend to form the inferior mesenteric plexus and the hypogastric plexus. At the level of the hypogastric plexus, sympathetic fibers are joined by the sacral parasympathetic nerves. Paravertebral sympathetic postganglionic neurons of the chain predominantly innervate the blood vessels of the gastrointestinal tract, while the postganglionic neurons residing in the prevertebral ganglia innervate vasculature and the enteric neurons in both plexuses to influence secretory and motor function (reviewed in [43]).
As with the parasympathetic preganglionic neurons, sympathetic preganglionic neurons are cholinergic. The postganglionic neurons, however, are primarily noradrenergic although other neuropeptides and purines are also released. In particular, adenosine 5′-triphosphate (ATP) plays a critical role in the vasoconstriction of submucosal arterioles [44–46].
16.2.2.4 Gastrointestinal Sensory Innervation
The extrinsic sensory innervation of the gastrointestinal tract is a combination of vagal and splanchnic pathways. While vastly outnumbered by the extensive intrinsic sensory neurons of the enteric nervous system, the vagus conveys considerable sensory information in that vagal afferents outnumber efferents by a 10:1 ratio. Conversely, splanchnic-derived afferents account for less than 10 % of thoracolumbar sensory information.
In order to maintain energy homeostasis of the organism, mechanosensory and chemosensory fibers from the digestive tract are critical for relaying the volume and composition of gastrointestinal contents to the CNS. Briefly, afferent fibers within the vagus nerve innervating the gastrointestinal smooth muscle transduce either the mechanical stimuli generated by distension or contraction, while the chemical coding of pH, nutrient composition, and the postprandial release of neurotransmitters and neuropeptides are conveyed by chemosensitive fibers within the mucosa and submucosa [47]. The transmission, and the ultimate perception, of visceral nociceptive stimuli is generally considered to be relayed through the splanchnic nerves and terminating within the spinal cord [48].
16.2.3 Gastrointestinal Vasculature
The gastrointestinal tract is one of the most highly perfused organ systems in the body (Fig. 16.3). The vascular supply of the oropharynx and the cervical esophagus is through the superior and inferior thyroid arteries. The upper thoracic esophagus is vascularized through esophageal branches of the descending aorta, while the remainder of the gastrointestinal tract is vascularized by the celiac trunk which supplies the distal esophagus, stomach, and the proximal duodenum through three main branches: the left gastric artery, the common hepatic artery, and the splenic artery. The esophagus receives its blood supply from the esophageal artery, which is a branch off of the left gastric artery. The left and right gastric arteries are responsible for the lesser curvature, while the left and right gastroepiploic arteries feed the greater curvature. The duodenum has a “dual” blood supply, arising from both the celiac trunk and the superior mesenteric artery (SMA). Branches that arise from the gastroduodenal artery, a branch of the common hepatic artery, supply the proximal duodenum. These arteries include the supraduodenal arteries, the retroduodenal arteries, the anterior superior pancreaticoduodenal artery, and the posterior superior pancreaticoduodenal artery. The distal duodenum is supplied by the anterior and posterior inferior pancreaticoduodenal arteries, which originate from the SMA. The inferior pancreaticoduodenal arteries form an anastamosis with their superior counterparts, thus creating a collateral circulation between the foregut and midgut.


Fig. 16.3
The principal vascular supply of the gastrointestinal tract arises from the celiac and superior and inferior mesenteric arteries. The jejunal and ileal arteries, which comprise much of the mesenteric arcade and the small intestine, were omitted for clarity
Approximately 15–18 jejunal and ileal arteries, which arise from the SMA, supply the remainder of the small bowel. These arteries travel within the mesentery, unite, and create arcades, which in turn give rise to vasa recta. In the jejunum, the arcades are simple and short with long vasa recta, while in the ileum, the arcades are complex and display short vasa recta. The SMA provides three additional branches that are responsible for supplying the cecum, ascending colon, and proximal 2/3 of the transverse colon. These branches are the middle colic artery, the right colic artery, and the ileocolic artery. The middle colic artery splits and partially travels along the transverse colon, while the other part anastomoses with the right colic artery along the ascending colon.
Lastly, the inferior mesenteric artery (IMA) is responsible for the hindgut, which extends from the distal 1/3 of the transverse colon to the upper anal canal via three main branches: the left colic artery, the sigmoidal artery, and the superior rectal artery. The left colic artery vascularizes the descending colon and the remaining distal 1/3 of the transverse colon. In doing so, the left colic artery anastomoses with the middle colic artery, creating an arterial connection between the midgut and the hindgut. The anastomosis between the left, middle, and right colic arteries gives rise to the marginal artery (artery of Drummond), which extends the entire length of the transverse colon. Lastly, the sigmoidal artery supplies the sigmoid, while the superior rectal artery provides for the rectum and upper anal canal. The inferior rectal arteries (originating from the internal pudendal artery) are responsible for supplying the lower anal canal, while the middle rectal arteries (originating from the internal iliac artery) supply the region in between the upper and lower anal canals.
16.3 Upper Gastrointestinal Function in Health and Following SCI
16.3.1 Esophageal Disorders
The incidence of swallowing dysfunction among persons with SCI has been noted from 17 to 43 % in the literature [49, 50]. A common condition is dysphagia which is defined as difficulty in swallowing and may include impairments of moving solids and/or liquids from the oral cavity into the esophagus.
16.3.1.1 Neurophysiology of the Esophagus
Normal swallowing results in the movement of ingested solids and liquids from the oral cavity to the esophagus. Specifically, the task of swallowing involves a complex motor pattern divided into physiologically relevant brainstem effector programs of the oral cavity, pharynx, esophageal body, and esophageal sphincters. The oral phase of swallowing involves voluntary rearward movement of the contents in the oral cavity that triggers subsequent autonomous and coordinated processes. The pharyngeal phase of swallowing includes motor sequences to move ingested items around the larynx, through the pharyngeal esophageal sphincter, then into the esophagus. Equally important autonomous respiratory reflexes of laryngeal elevation and closure, epiglottis folding over the larynx, and adduction of the arytenoids are integrated with esophageal reflexes for proper airway protection [51]. The esophageal phase promotes transient upper esophageal sphincter relaxation and peristalsis in the pharynx and esophagus that propels contents aborally. Ultimately, transient relaxation of the lower esophageal sphincter permits flow into the stomach. Tonic closure of the upper and lower esophageal sphincters prevents retrograde movement of contents unless the specific motor program for reflexive antiperistalsis is evoked in order to propel gastric contents orally as occurs during belching and emesis [52].
Common to the excitatory and inhibitory reflexes necessary for esophageal peristalsis is the dominant role of central pattern-generating neural circuits within the medulla including the nucleus tractus solitarii, nucleus ambiguus, and adjacent reticular neural circuits (reviewed in [52, 53]). Spinal sympathetic control centers for the esophagus have been implicated in reflex functions for vascular, glandular, and sphincter tone [53–55].
Afferent innervation of the esophagus is a combination of spinal projections through the dorsal root ganglia as well as vagal afferent fibers with cell bodies located within the jugular and nodose ganglia (reviewed in [56, 57]). Vagal afferent distribution within the esophagus of laboratory animals is not uniformly distributed but demonstrates greater density proximally [58, 59]. Similar differences in sensory distribution are inferred for humans based upon functional studies [60]. These limited data suggest that the proximal esophageal mucosa is more sensitive to both mechanical distension and chemical stimulation.
16.3.1.2 Clinical Presentation
Dysphagia symptoms are temporally related to swallowing and may include the inability to voluntarily ingest food, hoarseness, shortness of breath, coughing with swallowing, choking, sensation of food sticking in the esophagus, regurgitation of ingested items into the mouth or nose, sour- or bitter-tasting food and liquids, or chest discomfort [61–63]. Dysphagia following high-level SCI is often accompanied by diminished ability to generate elevated intrathoracic pressures during cough [64], thereby necessitating intensive management of the airway to prevent aspiration pneumonia [65].
16.3.1.3 Clinical Evaluation and Treatment
A bedside swallow evaluation (BSE) is an economical initial evaluation performed at the bedside by a speech pathologist in coordination with a respiratory care practitioner. A positive test is when swallowing dysfunction is identified in any of the phases of swallowing. There are two options for direct visualization using instruments that also will allow for therapeutic interventions: videofluoroscopy swallow study (VFSS) and fiber-optic endoscopic evaluation of swallowing (FEES; [66]). VFSS is a direct visualization of the anatomy and functional test of swallowing. Radiopaque solids and liquids of varying consistencies are visualized using barium and fluoroscopy. Penetration is the movement of solids or liquids entering the larynx, rather than around the larynx. When the movement of solids or liquids flows below the true vocal folds and into the trachea, rather than the normal path of properly flowing down the esophagus, this event is called aspiration. A positive test includes visualization of ingested test materials in the valleculae, pyriform sinus, or larynx or dysfunctional laryngeal elevation or epiglottic closure or inversion. Using a flexible fiber-optic laryngoscope, FEES involves the direct examination of the function of swallowing using trials of varying consistencies of food and liquids. From either VFSS or FEES, therapeutic interventions with “dietary or behavioral” adjustments can be determined. It should be noted that diagnoses of dysphagia in cervical spinal cord-injured population have identified potential confounds. These include causal relationships of dysphagia during artificial ventilation techniques (including tracheotomy) and anterior vs. posterior approaches during spinal stabilization [50, 62, 63]. Treatment of swallowing disorders follows current standard practice for the underlying etiology, and neuromodulatory strategies that promote neural plasticity in combination with standard swallowing rehabilitation practice are research techniques with potential clinical application.
16.3.1.4 Gastroesophageal Reflux
Gastroesophageal reflux disease is the reflux of gastric contents into the esophagus [67]. Normal function is dependent upon the lower esophageal sphincter (LES) and crural diaphragm acting in concert to form a barrier preventing reverse movement of gastric contents into the esophagus. Despite inconclusive evidence of lower esophageal sphincter dysfunction [68, 69], other reports suggest diminished lower esophageal sphincter tone following SCI. Later evidence in spinal cord-injured subjects demonstrated endoscopic and histological evidence of esophagitis as well as diminished esophageal contractility [70].
The incidence of gastroesophageal reflux disease (GERD) for individuals with SCI has been reported at approximately 22 % [71]. Reports note a higher incidence of gastroesophageal reflux and hiatal hernia in persons with spinal cord injury greater than 5 years duration [72].
Esophageal motor dysfunction carries tremendous clinical implications regarding the risk of aspiration, inflammation, and ulceration that accompanies deglutition or esophagogastric reflux, yet there are relatively few reports addressing esophageal function following SCI [68, 69]. Based upon the neurophysiology of the esophagus, it is imperative to note that GERD in the able-bodied is emerging as a complex interaction of altered esophageal sensory and motor neurocircuitry [73]. This is likely to be exacerbated following damage above the levels of the spinal cord receiving dorsal root ganglion projections from the esophagus.
16.3.1.5 Clinical Presentation
Unlike the able-bodied population, the perceived visceral sensations that accompany GERD (e.g., heartburn, chest spasm and regurgitation) are largely under-appreciated and, therefore, infrequently reported to health-care providers [69, 71, 72]. The prevalence of a supine or reclined posture in higher-level paraplegia or tetraplegia may increase the incidence of GERD however quantitative evidence for such a predisposition is lacking. Subsequently, histological and endoscopic evidence of GERD is frequently necessary for a conclusive diagnosis [70].
16.3.1.6 Clinical Evaluation and Treatment
A patient’s taken history alone can be diagnostic for GERD. Testing may include empiric therapy with acid suppression and follow-up for evaluation of resolution of symptoms. If the diagnosis of GERD is unclear or empiric therapy with proton pump inhibitors fails, PH monitoring and evaluation of obstruction, anatomic deformity causing disruption of the gastroesophageal tract, and evaluation for a hiatal hernia may be considered [67–76]. Upper gastrointestinal endoscopy may be ordered for concern of dysphagia, a high risk for developing histopathology including esophageal adenocarcinoma (e.g., Barrett’s esophagus), or failed acid-suppression therapy [74]. Manometric studies may be done for evaluation of esophageal dysmotility (see [66, 75]).
16.3.1.7 Preclinical Evidence
The motor programs for esophageal reflexes involve integrative circuits incorporating the trigeminal nerve (CN V; muscles of mastication), facial nerve (CN Vll; facial muscles, salivary glands, and mucous membranes of soft and hard palate), glossopharyngeal nerve (CN lX; parotid salivary gland and pharynx), vagus nerve (CN X; the tongue, soft palate, pharynx, larynx, and esophagus), and hypoglossal nerve (CN Xll; extrinsic musculature of the tongue) that reside within the brainstem. Each of these circuits remains anatomically intact following SCI. Exception to this would be in the scenario of traumatic or nontraumatic facial and head nerve injuries such as in polytrauma, radiation treatment, or postsurgical interventions. Thus, the prevalence and potential mechanism of esophageal dysfunction after human SCI remain largely unresolved, and no studies modeling the presentation of esophageal dysfunction following experimental SCI have yet emerged.
16.3.2 Gastrointestinal Bleed
Gastrointestinal bleed following SCI is most often associated with gastroduodenal ulceration [76]. Peptic ulceration has been frequently reported following traumatic injuries requiring intensive care [77, 78]. Gastrointestinal bleeding is more prevalent in patients with cervical or high thoracic lesions and has increased frequency in complete injuries [76, 79, 80]. Rates can be as high as 5.5 % in this population [81]. Though variable in studies, the rates of gastrointestinal hemorrhage are similar in traumatic (2.5 %) versus nontraumatic etiologies (2.6 %; [82]).
16.3.2.1 Clinical Presentation
Presentation is often insidious when chronic or abrupt because of the lack of early symptoms. Hemodynamic instability and cardiopulmonary decline are common presenting signs. Upper gastrointestinal bleed (UGIB) classically presents with hematochezia and black tarry stools but, if massive, can also present with bright red blood per rectum. Lower gastrointestinal bleed (LGIB) classically presents with maroon stools (right side of the colon), bright red blood per rectum (left side of the colon), and melanic (rectocecal). Gastrointestinal hemorrhage as a result of perforation may initially go unnoticed until there is clear hemodynamic instability [83]. Localization must be done clinically initially, which can be difficult without patient symptoms for guidance.
Provocating factors are multifactorial and likely include plasma stress hormone-mediated ulceration, diminished supraspinal controls leading to unopposed parasympathetic dysfunction, gastric vascular changes, oxidative stress, as well as the controversial use of steroids for treatment of SCI. While there is some evidence against steroids causing gastrointestinal bleed in SCI [79], high-dose steroids are frequently cited to place the stomach at increased risk of ulceration and hemorrhage in human and animal studies [84–86]. In a retrospective case controlled study, the rate of gastrointestinal hemorrhage in SCI was 2.77 % with 33 % mortalities while receiving high-dose steroids, while there were none in the control group [85]. This prevalence is similar to previously reported rates of 1.9–3.5 % depending on low or high doses of dexamethasone [87].
16.3.2.2 Clinical Evaluation and Treatment
Emergent attention to hemodynamic stability and cardiopulmonary monitoring are required. Identification of the location of the hemorrhage can be categorized as upper and lower gastrointestinal source. When upper gastrointestinal bleed (UGIB) is suspected, upper gastrointestinal endoscopy is the test of choice [88]. When lower gastrointestinal bleed (LGIB) is suspected, fiber-optic flexible colonoscopy is the initial diagnostic tool of choice. Further evaluation would depend on the outcome of initial testing and suspected source [89].
Treatment follows current standard practice for the anatomic location and underlying etiology of the GIB [88, 89]. Stress ulcer prevention with appropriate prophylaxis can reduce the rates of gastritis leading to hemorrhage. The prophylactic administration of proton pump inhibitors or histamine-2 receptor antagonists is widely employed in the intensive care unit and may minimize this particular comorbidity, though whether such practices are justified remains controversial [90, 91]. There is also evidence suggesting that utilizing early nutrition with oral or total parental nutrition to meet the patient’s total energy requirements will reduce the ulceration rate [92, 93].
16.3.2.3 Preclinical Evidence
Studies of gastrointestinal bleed following experimental SCI are limited. Animal models have employed low cervical spinal cord transection to provoke ulcerogenesis rapidly within 6 h post-injury [94–97], though a systematic investigation of the mechanisms provoking ulcerogenesis in experimental contusion models of SCI have not been performed.
16.3.3 Gastrointestinal Dysmotility
16.3.3.1 Neurophysiology
The principal functions of the stomach involve (1) a reservoir component for ingested solids and liquids. (2) reduction of the size of food particles through both digestive secretions and the mechanical milling evoked by gastric contraction and relaxation, and (3) the feedback-mediated propulsion of ingesta into the duodenum. The gastric compartment can be subdivided into the fundus, which serves as reservoir and regulates intragastric pressure, and the more muscular corpus where food is milled until reduced in size in order for contraction of the antrum to facilitate passage through the pylorus leading to the duodenum. The principal functions of the proximal duodenum include (1) neutralization of acid in the chyme delivered from the stomach, (2) enzymatic reduction of particles to simple molecules, (3) passive or active absorption of nutrients across the gastrointestinal wall, and (4) peristaltic movement of intestinal contents.
Unlike the organized patterns of digestion produced within the small and large intestines, the enteric nervous system innervating the stomach lacks the capacity to independently control the moment-to-moment changes necessary for appropriate receptive relaxation reflexes, associated with swallowing, as well as gastric milling and/or emptying reflexes. Considerable evidence has accumulated demonstrating that the proximal portion of the gastrointestinal tract is under direct modulation by extrinsic neural circuits. This parasympathetic neural input is in the form of vago-vagal reflex circuits that dominate gastric function, while sympathetic inputs to these target organs are largely confined to regulation of vascular beds and smooth muscle sphincters by indirect modulation through the enteric nervous system. Considerable feedback mechanisms exist between the antrum, pylorus, and duodenum for the delivery of chyme in a manner that does not exceed the digestive capacity of the small intestine [98, 99]. These mechanisms are certainly affected by diminished gastric emptying and dysregulation of antroduodenal coordination [100]. As described above, following normal exposure to both the appropriate composition of reduced food particles and the rate of transpyloric delivery of chyme, the duodenum releases gastrointestinal peptides and hormones that are integral to these feedback mechanisms [101–103]. Specifically, gastric reflex function is integrated by circuits within the brainstem dorsal vagal complex (Fig. 16.4), which comprises the area postrema, the nucleus tractus solitarius, and the dorsal motor nucleus of the vagus [104].
The dorsal vagal complex contains the vagal gastric neurocircuitry that serves as an integrative hub for activity that is the result of inputs originating from higher central nervous system areas [105–107], from spinosolitary inputs [108–110], as well as from neurohormonal signals from the periphery. Neurohormonal signaling occurs as a result of the fenestrated capillaries within the dorsal vagal complex that permit diffusion of circulating neuromodulators across a “leaky” blood-brain barrier [111]. All of the signals integrated within the dorsal motor nucleus of the vagus finely tune the coordinated emptying of nutrients from the stomach by way of an interaction between two separate postganglionic circuits under the influence of the dorsal motor nucleus. The parasympathetic preganglionic vagal motoneurons comprising the efferent limb of the vago-vagal reflex are cholinergic and activate postganglionic neurons via actions at a nicotinic receptor. Gastric projecting neurons within the dorsal motor nucleus of the vagus exhibit a basal rate of spontaneous firing [112–114], and it is this low frequency firing which regulates an excitatory (cholinergic) circuit that ultimately promotes the antral milling of ingested solids and the delivery of reduced particles to the duodenum [115].
Gastric relaxation can occur as a consequence of inhibiting this tonically active excitatory pathway [39, 116–119]. Conversely, activation of a non-adrenergic non-cholinergic (NANC) inhibitory vagal projection to the stomach elicits a direct inhibition of gastric motor functions. While this inhibition is often attributed to the release of nitric oxide [39–42, 120–122], purinergic and vasoactive intestinal polypeptide mechanisms have also been identified [31, 41, 123].
16.3.3.2 Clinical Presentation
The majority of reports in the clinical literature describe derangements in upper gastrointestinal reflex emptying and motility, especially after spinal cord injuries occurring above the mid-thoracic spinal segments [70, 100, 124–130]. In extreme cases, the high degree of gastric feeding intolerance demonstrated by these patients necessitates aggressive nutrient supplementation through enteral, parenteral, or invasive surgical interventions in order to maintain positive energy and nitrogen balance [12, 13, 131, 132]. Despite these clear alterations, the mechanisms for gastric reflex dysfunction remain poorly characterized and inconsistently managed in the clinic.
16.3.3.3 Clinical Evaluation and Treatment
Recent technologies permit noninvasive assessment of gastrointestinal dysmotility without radioisotopes [130, 133], though there is no indication that such technologies are routinely employed in clinical settings. Early studies on the effects of SCI on small intestinal function reported orocecal transit times using hydrogen breath testing [128, 134]. While this technique revealed delayed mouth-to-cecum transit times, the technique does not provide clear distinction between reduced gastric emptying and intestinal peristalsis. Determination of specific changes in intestinal motility patterns were not achieved until an orally ingested magnetic tracer was employed [7]. These authors replicated the observed reduction in orocecal transit time, though intestinal contractility was unaffected. This latter observation was attributed to enteric-mediated smooth muscle activity. A small sample study of SCI subjects revealed a delay in gastric emptying of solids using the noninvasive [13C]-octanoate breath test [135].
The reflex control of the stomach is under considerable modulation by a wide range of gut hormones [136]. Despite long-established evidence regarding the role of gastrointestinal hormones in nutrient ingestion and passage throughout the gut, the evaluation of this important regulatory system in the spinal cord-injured population is scarce. Motilin, a 22-amino-acid peptide released from the upper intestine, stimulates gastric and intestinal myoelectric activity during phase III contractions of the migrating myoelectric complex of the interdigestive phase. Comparisons of serum motilin levels in a limited sample of uninjured, paraplegic, and tetraplegic subjects revealed that motilin levels were largely similar across all three groups, though there was a trend toward elevated motilin levels in the paraplegic group [137]. Peptide YY is a 36-amino-acid peptide hormone that is similar to gastrointestinal peptides pancreatic polypeptide and neuropeptide Y that is released from epithelial cells within the ileum and colon. However, the actions of circulating peptide YY target the upper GI as an “ileal brake” whereby peptide YY potently diminishes gastric acid secretion, gastric emptying, intestinal propulsion, and pancreatic exocrine secretion [102]. In this same study described above, levels of peptide YY in chronic SCI individuals were similar in the fasted state but were significantly elevated in the early postprandial state of tetraplegic subjects [137]. The limited sample size and the absence of essential physiological parameters, such as gastric emptying rates during the postprandial serum measurements, limit the interpretation of these findings and merit reevaluation in a larger sample from the SCI population. Finally, the neurohormone ghrelin, previously described as being secreted from oxyntic cells within the gastric mucosa [138, 139], is upregulated during periods of negative energy balance, such as before meals, and is downregulated after feeding [140]. Furthermore, there are structural and genetic similarities between ghrelin and motilin receptors [141], though there is no cross talk between the respective ligands [142, 143]. The clinical utility of ghrelin and ghrelin mimetics as an endogenous therapeutic target has garnered widespread attention for the treatment of gastrointestinal motility disorders [144]. A study of uninjured, paraplegic, and tetraplegic individuals reported no differences in levels of serum ghrelin across all groups following an overnight fast [145]. Unfortunately, with the exception of a study on eating attitudes [146], clinical evidence regarding the dietary behaviors of the spinal cord-injured population is lacking. In contrast to the spinally intact clinical population, studies regarding the orexigenic and pro-motility responses to exogenous ghrelin following SCI are nonexistent.
16.3.3.4 Preclinical Evidence
Animal models of spinal transection or studies employing an established model of contusion SCI [147] are beginning to reveal similarities in gastric dysmotility between rodent models and that reported for humans. Expanding upon the initial reports that high thoracic spinal transection delayed the emptying of a liquid test meal [148, 149], diminished food intake has been reported in chronic T3-SCI rats [150]. Further physiological studies have recorded circular smooth muscle contractions [151] at rest, in response to pharmacological challenge, and following elicitation of the esophagogastric reflex [152]. Using techniques to elicit this reflex following T3-SCI, physiological distension of the esophagus failed to elicit a reflex relaxation of the stomach [153]. This impaired accommodation reflex may contribute to dysphagia and reflux of ingested matter and early postprandial fullness. Furthermore, this reduction in gastric reflex activity was not altered by sympathectomy [153]. These results were in agreement with earlier conclusions that post-SCI dysmotility was mediated through possible alterations in the anatomically intact vagal neurocircuitry [149].
Studies using [13C]-octanoate-tagged solid meals in order to indirectly measure gastric emptying in awake animals confirmed that gastric dysmotility is accompanied by a delay in gastric emptying and that both diminished gastric emptying and dysmotility persist up to 6 weeks after T3-SCI [154]. These persistent deficits suggest that delayed gastric emptying is unlikely to be due to “spinal shock” as gastric dysmotility persists long after experimental spinal cord injury animals are generally considered to have stabilized (ca. 3–6 weeks post-injury).
Circulating cholecystokinin has been implicated in stimulation of the gall bladder, pancreas, and gastrointestinal motility (reviewed in [136]). It is also produced by a number of neurons within the central nervous system and is implicated in feeding behavior and nociception. No evidence is available in the clinical literature regarding cholecystokinin function following spinal cord injury; however, experimental studies have tested the sensitivity of vagally mediated gastric reflexes in T3-SCI rats [155]. In regard to gastrointestinal actions, it is well accepted that peripheral cholecystokinin activates C-type vagal afferent fibers that project to nucleus tractus solitarius cells [156–158]. Study of experimental SCI was particularly revealing in that peripheral administration of the sulfated cholecystokinin octapeptide (CCK-8 s) 3 days after injury induced significantly less activation in the nucleus tractus solitarius than in uninjured control rats [155]. In the same experimental animals, c-Fos expression within the adjacent area postrema was similar in both groups, suggesting that gastric neurocircuitry involving the nucleus tractus solitarius was selectively impaired. Previous experimental studies suggest that in addition to peripheral effects upon vagal afferents within the gastrointestinal tract, cholecystokinin acts directly upon brainstem vagal circuits [159–166]. However, T3-SCI rats did not demonstrate a gastric efferent vagal response to central microinjection of CCK-8s into the DVC, and the reduced sensitivity to centrally administered CCK-8s in the DVC persisted at 3 weeks after injury [155]. These data also support the hypothesis that post-injury dysmotility is mediated through alterations in gastric vagal neurocircuits.
In experimental studies, ghrelin is known to exert stimulatory effects upon gastric motility and acid secretion as well as food intake and energy metabolism [167–171]. This stimulatory effect occurs in both fed and fasted states [172, 173]. A central inhibitory effect of ghrelin has been reported for fundic tone of fed animals [171], whereas an excitatory effect has been demonstrated in vitro within brainstem vagal neurocircuits and in vivo with gastric corpus contractions in fasted animals [174]. Similar to the observations for cholecystokinin following experimental SCI, emerging studies with peripheral and central administration of ghrelin also reveal a reduced sensitivity within the nucleus tractus solitarius of T3-SCI rats [175, 176].
The common feature of these aforementioned animal studies is the diminished sensitivity of gastric projecting vago-vagal reflex circuits to important gastrointestinal peptides. As described above, and for any neural reflex circuit, vago-vagal control of gastric motility is regulated by afferent and efferent limbs, and dysfunction in either of these limbs will impair proper gastric function. Recent data has provided convergent anatomical, neurophysiological, and functional evidence for the integrity of gastric-projecting dorsal motor nucleus of the vagus neurons in acute T3-SCI rats [177].
In summary, the apparent reduction in gastric vagal afferent responsiveness to mechanical and chemical stimuli suggests a generalized hyposensitivity of vagal afferent neurotransmission to the brainstem following SCI. Evidence of vagal afferent hyposensitivity has been identified in other GI pathophysiological states [178, 179]. In particular, Xue and colleagues suggest that part of the diminished visceral afferent sensitivity in an inflammation-induced model of functional dysmotility is mediated through an inducible nitric oxide synthase (iNOS) mechanism [179]. While this observation was limited only to afferents within the mesenteric arcade and did not include the vagus, emerging evidence supports specific vagal hyposensitivity following experimental SCI [180].
16.3.4 Gastroenteritis
One implication of delayed orocecal transit in the spinally injured individual is the possibility of small intestinal bacterial overgrowth. The association between bacterial overgrowth and functional gastrointestinal disorders, specifically those that are not linked to neurotrauma, remains controversial [181]. However, the role of the gastrointestinal microbiome in health and disease states is rapidly advancing, and the interactions between nutrient composition, gastrointestinal transit, and the microbiome have only recently started to be explored [182]. Gastroenteritis in SCI patients can be viral or bacterial in nature. While no incidence of either cause has been elucidated, it was determined in one small cross-sectional cohort that 50 % of patients were carriers for Clostridium difficile and 55 % for vancomycin-resistant Enterococcus (VRE).
16.3.4.1 Clinical Presentation
The traditional response of infection on the gastrointestinal system can be blunted or exaggerated and often masked by lack of sensation [183]. The impact of gastroenteritis is measured in the clinical treatment and the persistence of bowel incontinence. In one epidemiologic study, 62 % of SCI patients as compared to 8 % of normal subjects reported a decrease in quality of life due to fecal incontinence [184].
Symptoms may include devastating results to continence in the form of total fecal incontinence or changes in stool patterns. Clinical signs may or may not be associated with fevers and chills. Autonomic dysreflexia could easily be the presenting symptom for gastroenteritis. Pain will be variable depending on the level and degree of impairment of sensation. It may include referred pain from the abdomen such as shoulder pain or may present as bloating pain in the abdomen. The physical exam may indicate a spastic abdomen with hyperactive bowel sounds. Conversely, there can also be distention of the abdomen with decreased bowel sounds, which in one case report was associated with Clostridium difficile colitis [185].
16.3.4.2 Clinical Evaluation and Treatment
Gastroenteritis management and treatment should typically follow current institutional standard practice for the underlying etiology (cf., [186]). Treatment must involve adequate hydration as many chronic SCI patients fluid-regulate to control catheterization volumes, which can put them at risk for dehydration if output is pathologically increased. Additionally, cessation of laxatives in a non-constipated patient is advisable. If bacterial, treatment should be focused on appropriate treatment of C. difficile with metronidazole or oral vancomycin or of VRE with linezolid. If the stool is profuse, a fecal collection system can be employed, and in rare cases, a rectal plug is considered. Surgery is only considered in severe cases of colitis.
If the patient received antibiotics recently, a high suspicion for C. difficile remains, and stool analysis should be performed. An abdominal plain radiograph can be used to evaluate the stool burden in chronic constipation and air-fluid levels in small bowel obstruction. Infection by C. difficile and pseudomembranous colitis may occur more often in SCI patients treated with trimethoprim-sulfamethoxazole. Contact precautions are necessary for prevention of spread of infection to other populations when the patient is hospitalized. Precautions for C. difficile include handwashing because of the decreased ability of alcohol sanitizers to adequately kill the spores.
16.3.5 Neurogenic Bowel (Upper Motor Neuron)
16.3.5.1 Neurophysiology
The colon, rectum, and the internal anal sphincter form an integrated unit for the final digestive processing, storage, transport, and elimination of gastrointestinal contents (reviewed in [187, 188]). As with the upper gastrointestinal tract, smooth circular and longitudinal muscle layers serve either to mix or to propel luminal contents toward the distal-most rectum. Continence is primarily maintained by the resting tone of the smooth muscle of the internal anal sphincter (IAS) and is augmented by contractions of the striated external anal sphincter muscle. The contribution of distal GI tract anatomy and associated pelvic floor musculature that is particular to a given species (especially humans) serves to further augment continence mechanisms by the mechanical alteration of the rectum. In the laboratory rat, pelvic floor muscles such as the levator ani are not involved in defecation reflexes [189], whereas a role for the iliocaudalis and pubocaudalis, beyond a hypothesized model for pelvic organ prolapse [190], remains to be determined.
Although the reflexes involved in defecation control appear to be mediated by sacral segmental circuits, their form, magnitude, and coordination appear highly dependent on descending input from rostral CNS structures. Anal sphincter reflexes are compromised in patients with spinal cord dysfunction, suggesting an important descending or spino-bulbo-spinal component [191]. These patients also exhibit chronic constipation and impaction, which has been attributed, in part, to excessive contractions of the external anal sphincter and dyssynergia with actions of the internal anal sphincter [192]. Similar difficulties with micturition and urethral sphincter musculature are well documented [193]. Clinical evidence suggests that caudal brainstem structures may mediate rectoanal inhibition in that colonic inertia and a loss of rectoanal inhibitory reflexes occur in patients with posterior brainstem lesions [194].
Enteric innervation of the IAS is through a continuation of rectal wall ganglion cells rather than local ganglion cells ([195] cited in Krier [196]). As such, the major innervation of the IAS is through both sympathetic and parasympathetic control. Anorectal sympathetic innervation originates in the autonomic nucleus of the lumbar spinal cord (refer to Fig. 16.2). Numerous preganglionic sympathetic fibers terminate in the inferior mesenteric ganglia and the pelvic plexus, the latter receiving sympathetic input by way of the hypogastric nerve originating in the inferior mesenteric ganglion [196]. Hypogastric nerve stimulation results in an increase in IAS pressure [197]; however, hypogastric-mediated sympathoexcitation of the IAS has been reported to occur only in response to supramaximal levels of the rectal stimuli necessary to evoke the rectoanal inhibitory reflex [198]. The preganglionic parasympathetic innervation of the IAS is through the pelvic nerves [196, 199]. The pelvic nerves modulate both cholinergic and non-cholinergic excitatory as well as non-adrenergic and non-cholinergic inhibitory postganglionic fibers to the rectum and IAS.
16.3.5.2 Clinical Presentation
Neurogenic bowel refers in particular to colonic dysfunction that presents as reduced colonic transit, constipation, disordered evacuation reflexes, and potential incontinence. By virtue of the parasympathetic and somatic neural circuitry located within the sacral spinal cord, neurogenic bowel is a common gastrointestinal disorder accompanying traumatic and nontraumatic SCI. Despite widespread recognition of diminished quality of life stemming from constipation and overflow incontinence, previous reviews of the literature reveal that individuals with spinal cord dysfunction are still offered interventions in response to neurogenic bowel rather than substantial evidence-based or preclinical data (see [200]).
In addition to the necessity of tetraplegics to rely upon assistance from caregivers regarding bowel management, the inordinate amount of time spent on bowel care by many SCI individuals is often cited as a primary factor in patient dissatisfaction and diminution of quality of life [201]. Various options are offered to individuals and range from conservative and noninvasive strategies to surgical treatments [202]. Taken as a whole, these strategies generally offer guidelines for tailoring a bowel management program to meet individual needs.
Recommendations for optimizing diet and fluids provide the most universal management protocol and are applicable in health and disease. Rectal chemical stimulants containing glycerine alone, or in combination with other agents, are commonly used to promote contractile and secretory processes within the colon due to the ease of administration and timing of effect. Oral pharmacological stimulants to promote both peristalsis (e.g., bisacodyl) and/or stool softening may be of limited value due to the rate of onset of effect in a population with diminished or absent rectoanal sensation and ability to maintain voluntary muscle control to aid in continence. Transanal irrigation offers another nonsurgical technique that is reported to be well tolerated [203]. If the individual retains reflexive anorectal-colonic function, digital rectal stimulation may be employed to promote reflex peristalsis and achieve evacuation. In more extreme instances, digital rectal evacuation may be necessary in order to actively remove impacted feces.
Numerous surgical interventions have been identified for more refractory bowel dysfunction. Antegrade continence enemas require a necessary surgical procedure as described by Malone and colleagues [204]. The long-term efficacy and stability of this technique remains poorly explored for the spinally injured population. While the precise mechanism and degree of efficacy for sacral stimulation on neurogenic bowel remains unclear [205], some degree of patient satisfaction has been reported [206, 207]. Colostomy or ileostomy offers greater ease of management and a high degree of satisfaction [208], and recent reports indicate that postsurgical complications and morbidity do not differ from able-bodied patients [209].
For most, if not all, of the procedures listed above, caution must always be exercised in individuals with injuries above T6 regarding triggering of autonomic dysreflexia in both poorly managed and aggressively managed neurogenic bowel [210].
16.3.5.3 Preclinical Evidence
As described previously, the enteric nervous system is capable of independently regulating gastrointestinal contractility and secretion through intrinsic neural circuits that process sensory information, relay that information through interneuronal pools, and ultimately effect activation of motor efferents [196, 211]. When separated from extrinsic input, these intrinsic reflexes are sufficient to set a basic pattern of contractility within the rectum and internal anal sphincter [212]. In vitro and in vivo recordings of IAS muscle reveal an increasing frequency gradient along the oral-aboral extent of the IAS that is substantially higher than that recorded within the rectum [213, 214]. The magnitude of intrinsic nervous system reflexes appears to be complemented by an extrinsic parasympathetic reflex [215], though the relative importance of either intrinsic or extrinsic rectoanal reflexes during defecation remains to be determined.
Studies in rodents have revealed the existence of descending inputs to the spinal neurocircuitry responsible for defecation reflexes [216, 217]. This pudendal circuitry principally consists of the somatic motoneurons innervating the external anal sphincter located dorsomedially in the lumbosacral ventral horn and preganglionic neurons in the sacral parasympathetic nucleus located laterally in the intermediate gray matter [217–220]. Studies that employed retrograde tracers unilaterally injected into the lumbosacral ventral horn region of the spinal cord identified projections from the nucleus raphe obscurus, raphe magnus, raphe pallidus, and lateral nucleus paragigantocellularis [221]. Utilizing this data, anterograde tracer injections were made into the nucleus raphe obscurus [218] or lateral nucleus paragigantocellularis [219] in animals that also received retrograde tracer injections into the external anal sphincter. These studies demonstrated nucleus raphe obscurus and lateral nucleus paragigantocellularis terminal appositions on external anal sphincter motoneurons, which are the only descending projections to the external anal sphincter that have been identified to date.
Physiological experiments have demonstrated that brainstem stimulation reduces anal sphincter reflexes [222] and that after complete spinal cord transection, a persistent hyperreflexia develops that may parallel human SCI [223]. After partial spinal cord contusion lesions, rats recover bowel reflexes over a period of several weeks, depending upon the severity of the lesion [224, 225]. Further research has revealed that the recovery curves for these reflexes (when observed at 3 days, 1, 3, and 6 weeks after surgery) correlate with the optical density of immunofluorescent-labeled serotonergic fibers [225]. These lesions spare some immunolabeled serotonergic axons traversing past the lesion center and are hypothesized to provide the substrate by which serotonergic sprouting may occur in the lumbosacral spinal cord. The most likely candidates for these fibers are the previously identified projections from the nucleus raphe obscurus and the rostral gigantocellular reticular nuclei complex [218, 219]. Functionally, both brainstem nuclei seem to inhibit some pudendal reflexes in that the rostral gigantocellular reticular nuclei complex may have a greater effect upon the bulbospongiosus motoneurons involved in sexual reflexes [226–230] and the nucleus raphe obscurus may have a greater effect upon external anal sphincter control since lesions of this nucleus produce a transient ano-anal reflex increase [231].
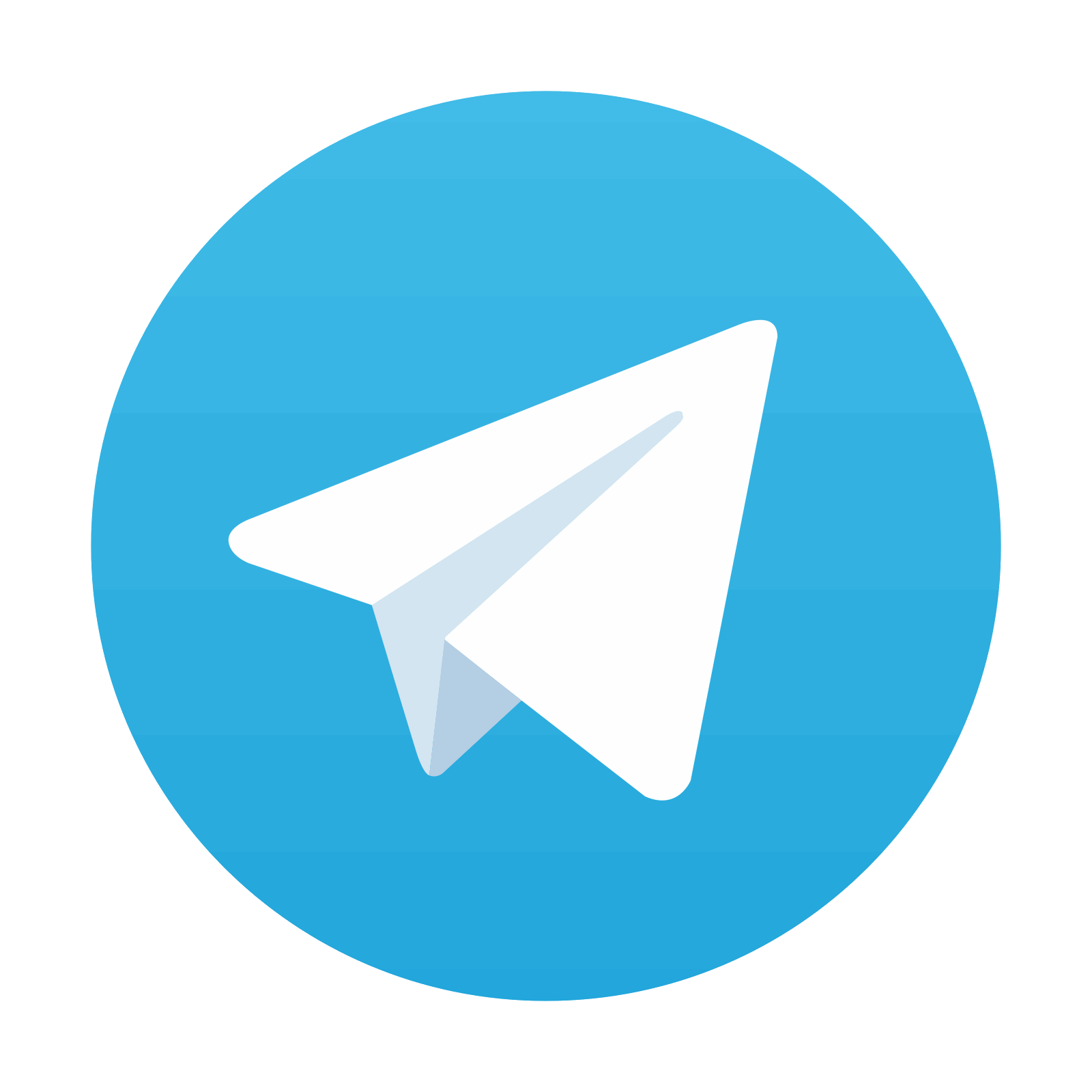
Stay updated, free articles. Join our Telegram channel

Full access? Get Clinical Tree
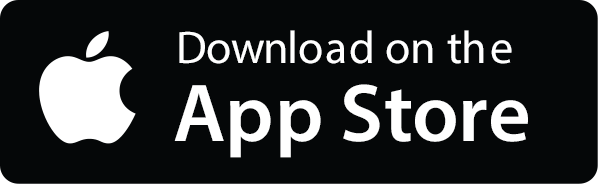
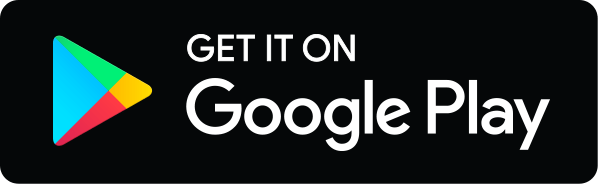