CHAPTER 2
Neurogenetics
I. The Central Dogma of Genetics
A. Deoxyribonucleic acid (DNA)
1. DNA is the molecular blueprint.
2. The genome refers to a species’ entire collection of DNA:
a. Human genome: 3 billion nucleotide bases subdivided into 23 paired chromosomes
b. Chromosomes 1 through 22 are autosomal chromosomes; X and Y are sex chromosomes.
i. Females are 46, XX; Males are 46, XY.
B. Genes
1. The human genome contains approximately 20,000 genes.
2. Genes specify amino acid sequences of proteins.
3. Approximately 1.5% of the human genome consists of protein-coding regions.
4. Noncoding sequences have structural or regulatory functions.
5. Gene structure:
a. Promoters: sequences of DNA upstream from the start codon
i. Binding sites for transcriptional machinery
ii. Transcription factors can activate or repress transcription at this site.
b. Exons: sequences of DNA that code for specific amino acid sequences
i. Codons: groups of three nucleotides (trinucleotides) that code for particular amino acids
ii. Start and stop codons are trinucleotides recognized by transcriptional complexes at the beginning and the terminus of the exon, respectively.
c. Introns: intervening DNA sequences between exons that are not expressed in the final amino acid sequence
6. Copies of the same gene are called alleles.
7. Noncoding regions: help regulate gene expression
a. Distal promoters, enhancers, silencers, insulators, five-prime untranslated region (5’ UTR), and the three-prime untranslated region (3’ UTR)
b. Intergenic noncoding regions: important for chromosomal structure and replication
i. Examples: the centromere and telomere
C. Transcription: DNA is transcribed into heteronuclear ribonucleic acid (RNA) containing both introns and exons (and UTRs).
1. Splicing: heteronuclear RNA is spliced, or edited, at specific sequences known as splice sites.
a. Introns are excised and adjacent exons are fused together.
b. Splicing yields messenger RNA (mRNA).
c. Splice-site variants: allow a single gene to produce more than one mRNA, yielding similar proteins with different biological functions
D. Translation: mRNA is translated into a protein on cytoplasmic ribosomes.
1. Transfer RNA (tRNA):
a. Binds to one specific amino acid
b. Contains a trinucleotide anticodon that binds a specific mRNA codon
c. tRNA links together specific amino acids and mRNA, allowing amino acids to bind together (i.e., be translated) into the primary sequence of the protein.
d. Posttranslational modifications: Linear amino acid strands will be further processed, folded, and shuttled to the protein’s functional site.
i. Many posttranslational modifications, such as glycosylation, occur in the Golgi or cytosol, are enzyme-mediated, and require chaperone proteins.
ii. Modifications are typically covalent additions of functional groups such as glycosylation, phosphorylation, methylation, ubiquitination, nitrosylation, acetylation, lipidation, or removal of regulatory subunits.
E. Mutations: changes in the genome that affect the production of proteins. There are myriad causes of mutations with varying effects. The following are common types of mutations, arranged by size from smallest to largest:
MUTATION | MUTATION TYPE | LEADS TO |
Point mutation: Single-nucleotide base change | Silent | Same amino acid, results in a normally functioning protein |
Neutral | Similar amino acid substitution, results in a normally or almost normally functioning protein | |
Missense | Amino acid substitution and potentially abnormal protein | |
Nonsense | Results in a premature stop codon, resulting in an abnormal or no protein | |
Insertions or deletions | Frame shift | Disrupts the “reading frame,” so the three-nucleotide codons are out of sequence; results in abnormal or no protein |
In-frame mutation | Insertion/deletion resulting in a sequence divisible by 3; may cause abnormal or no protein | |
Chromosomal deletion | Interstitial loss of DNA affecting all genes in that region; may result in fusion gene formation; effects depend on location and size of the deletion | |
Chromosomal duplication | Duplication of a part or whole chromosome; effects depend on the location and size of the duplication | |
Chromosomal translocation | An exchange of part of one chromosome with another nonhomologous chromosome; effects depend on the location, size, and whether associated deletions or duplications are present; fusion genes may form | |
Chromosomal inversion | A portion of a chromosome becomes reversed within the chromosome; effects depend on the location, size, and whether associated deletions or duplications are present; fusion genes may form | |
Splice-site mutation | Any of the above mutations that disrupt recognition of a splice site during mRNA processing | Improper inclusion of intronic RNA or exclusion of exonic RNA in the mRNA, resulting in an abnormal or no protein |
Gene fusion | Production of a single mRNA from two typically separate genes | Chimera protein that may or may not have loss or gain of function |
Dynamic mutations | Trinucleotide repeat expansion | Increasing number of trinucleotide repeats, typically glutamate codons, resulting in larger mRNAs; increased risk of genetic disease with larger trinucleotide repeats. Genetic anticipation refers to earlier disease expression in subsequent generations, and is a common consequence of trinucleotide repeat expansion. |
1. Mitochondrial DNA (mtDNA):
a. Mitochondria have their own DNA separate from the chromosomal DNA.
b. mtDNA has approximately 16,500 nucleotide bases and codes for 37 proteins (including 22 tRNAs).
i. mtDNA mutations: point mutations, deletions, duplications, or insertions in mtDNA
ii. Can result in abnormal proteins, many of which are critical for mitochondrial function
iii. Note: there are over 1,000 chromosomal genes encoding mitochondrial proteins, and mutations affecting these genes can result in mitochondrial dysfunction and disease.
F. Effects of mutations
1. Gene dosage effects:
a. Haploinsufficiency: loss of a gene copy resulting in less protein production than is required by a cell for normal function. Some cancers result from haploinsufficiency of a tumor suppressor gene.
2. Loss of function: mutations that disrupt protein structure, binding sites, functional domains, or loci of posttranslational modifications. These proteins may be less functional or nonfunctional, may not localize properly within the cell, or may be degraded by a cell more quickly than the normal protein. Loss of function mutations are common in many recessive neurologic diseases.
3. Gain of function: a mutation resulting in increased activity, increased half-life, or the creation of a new and abnormal function that disrupts other functions in the cell. Gain of function mutations in the SCN9A gene have been implicated in some patients with idiopathic small fiber neuropathy.
4. Dominant negative: a mutation in one copy of a gene may result in an abnormal protein that disrupts the function of the normal protein made by the nonmutated copy of the gene. Dominant negative mutations in the ClC-1 gene give rise to both dominant and recessive forms of the channelopathy myotonia congenita.
5. Lethal mutations: these are not compatible with life and often result in spontaneous abortion, intrauterine fetal demise, or death in the neonatal period.
G. Nonmutational DNA variants
VARIANT | SIZE OF VARIANT | CHARACTERISTICS |
Single-nucleotide polymorphism (SNP) | Single base change, typically in noncoding regions | Common occurrences, ~1% or more frequent in a population |
Typically binary (e.g., A or T) | ||
–1 million SNPs across the human genome | ||
SNP allele frequencies vary across ethnicities | ||
May be linked with disease or phenotype | ||
SNPs can alter transcriptional regulation | ||
Copy-number variation (CNV) | Vary from 1,000 nucleotides (1 kilobase or Kb) to. 1,000 Kb (megabase, or Mb) | A deletion or duplication of a region of a gene |
–13% of the human genome consists of CNVs | ||
Stable and heritable from parents to offspring | ||
CNV gains are more common than losses | ||
May be responsible for phenotypic variation, increased or decreased susceptibility to diseases | ||
Variants of unknown significance (VUS) | Variable | These are genetic changes that may be as small as single base substitutions or large copy-number variants. These VUSs have yet to be characterized, and their effects are not known. Some VUSs occur in coding regions of genes known to be associated with disease and are likely pathogenic. Others may be found in both unaffected parents and their affected offspring, and are less likely to be deleterious. |
H. Patterns of inheritance
1. Autosomal dominant (AD): inheritance or de novo mutation of one allele is sufficient to produce disease; 50% of offspring of an affected individual will receive the disease allele and therefore have the disease.
Penetrance: The degree of phenotypic expression of a mutation. For AD-inherited diseases this can be variable even within a family, with some members minimally affected while others with the same mutation will be severely affected. When all carriers are affected, this is 100% penetrance.
The absence of a family history does NOT preclude an autosomal dominant disease because variable penetrance, questions of paternity, and de novo mutation can all result in an affected offspring.
2. Autosomal recessive (AR): two mutated alleles must be present for the disease to manifest. If both parents are each unaffected carriers of an AR mutation, on average 25% of their offspring will have two normal copies of the gene (noncarriers), 50% will be unaffected carriers with a single copy of the mutated gene, and 25% of the offspring will inherit both mutated alleles and be affected. Typically, carriers are asymptomatic.
3. X-linked recessive: Gene mutations on the X chromosome can be inherited by both male and female offspring.
a. Males only have one X chromosome (from their mother); therefore in X-linked recessive diseases, a male receiving a mutated allele will manifest the disease, and a female offspring will not.
b. An unaffected carrier female has a 50% chance of transmitting an X-linked recessive disease to her sons.
c. Males with X-linked diseases CANNOT transmit the disease to male offspring, although 100% of their female offspring will receive the disease-causing allele and be asymptomatic carriers.
d. If a male with an X-linked recessive disease and an unaffected female carrier have children:
i. 50% of their male offspring will be expected to inherit the disease (from their mother)
ii. 50% of their female offspring will be unaffected carriers (from either mother or father)
iii. 50% of their female offspring will also inherit the disease (one copy from each parent)
4. X-linked dominant: these mutations are often so deleterious that survival of the offspring is contingent on having at least one normal functioning copy of the gene.
a. Because males only have one X chromosome, these mutations are typically lethal in male fetuses and likely result in spontaneous abortion, although rarely male patients are found with X-linked dominant diseases.
b. Because females contain two X chromosomes, they can survive with the disease-causing mutation.
c. The degree of severity of X-linked dominant diseases varies between individuals due to X-inactivation, a process by which one copy of a female’s two X chromosomes is transcriptionally silenced. Rett syndrome, caused by a mutation in the MECP2 gene, is an example of an X-linked dominant disease.
5. Genomic imprinting: DNA and histone methylation allow transcriptional silencing of individual genes, as well as regions of chromosomes. Genomic imprinting is the regulation of gene expression depending on the parental origin of the gene. In paternal imprinting, genes inherited from the father are silenced through methylation, and only those from the mother are expressed. Maternal imprinting is the opposite.
a. Prader-Willi: Paternally inherited mutation (maternal imprinting). You can remember this because both Prader and paternal start with P.
b. Angelman’s: Maternally inherited mutation (paternal imprinting). You can remember this because there is an M in Angelman’s.
6. Mitochondrial: at fertilization, the ovum contributes all of the mitochondria, and the sperm contributes none. Thus, mitochondrial inheritance is exclusively maternal, and ALL her offspring are at risk of manifesting a mitochondrially inherited mutation.
a. Heteroplasmy: within individual cells there exist thousands of copies of mtDNA, some of which may be mutated, and others normal. This is called heteroplasmy.
i. The degree of heteroplasmy correlates with disease severity, with a critical level of heteroplasmy above which symptoms are more likely to manifest.
ii. Patients with mitochondrial disease tend to accumulate heteroplasmy throughout their lives. Thus, patients with high levels of heteroplasmy at birth are at increased risk of earlier-onset disease.
II. Genetic Testing
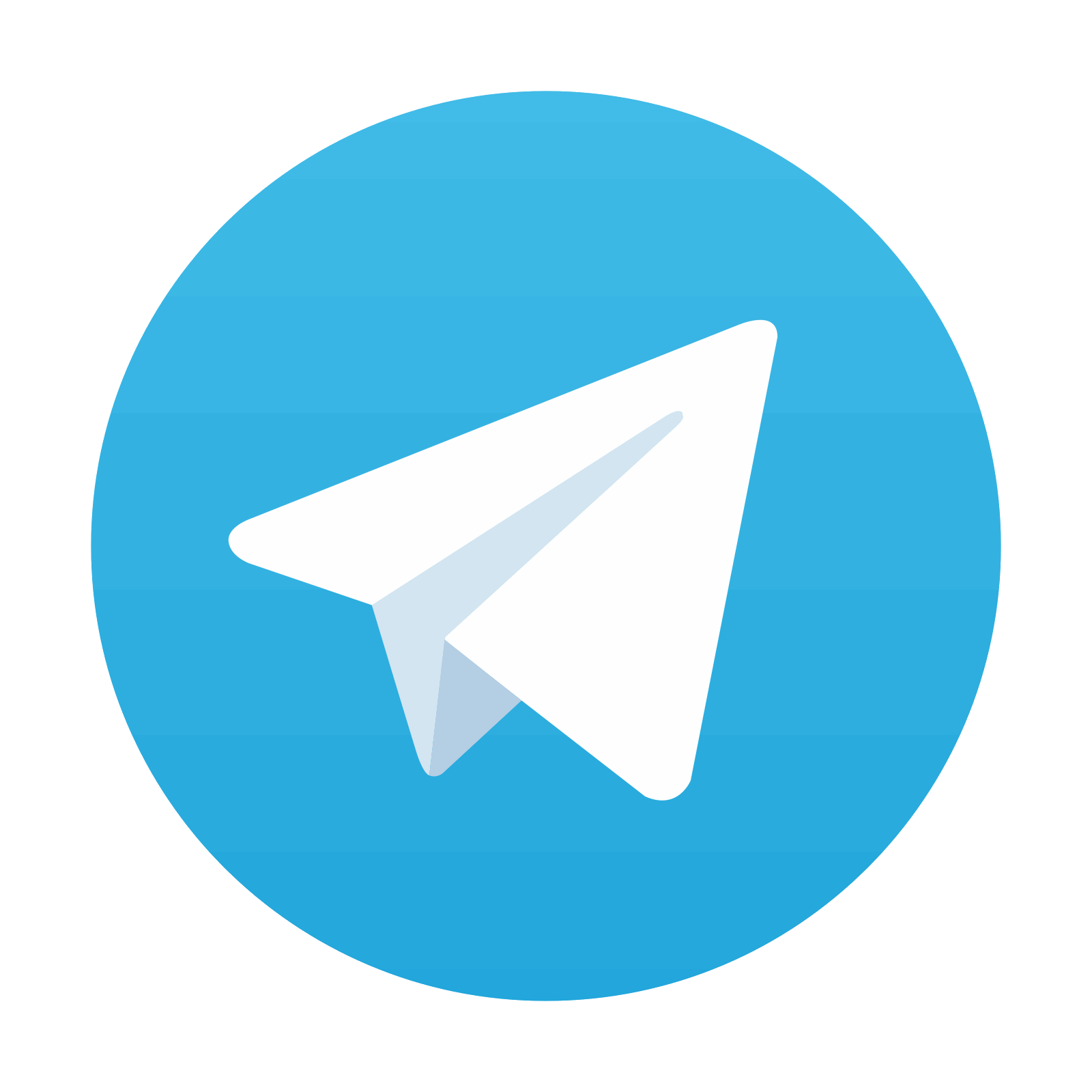
Stay updated, free articles. Join our Telegram channel

Full access? Get Clinical Tree
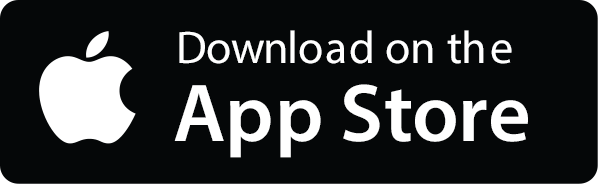
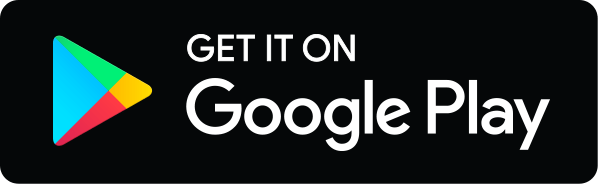