Method
Author
Subjects
Outcome
Structural MRI
Schwartzet al. (1997)
19 SAD/19 HC
No change of pituitary volume due to winter depression or season
Miranda-Scippaet al. (2008)
12 SAD/12 HC
No differences in pituitary volume between SAD and HC
Functional MRI
Vandewalleet al. (2011)
14 SAD/16 HC
Increased response to auditory emotional stimuli in the posterior hypothalamusdue to exposure of blue light in SAD
SPECT
[123I]ß-CIT
Willeitet al. (2000)
11 SAD/11 HC
Decreased [123I]ß-CIT binding in thalamus-hypothalamus in SAD
[123I]ß-CIT
Neumeisteret al. (2001)
11 SAD/11 HC
Reduced availability of striatal DAT in patients with SAD
[99mTc]HMPAO
Praschak-Riederet al. (1998)
Increased left frontal rCBF in patients with SAD. Normalisation in rCBF after successful light therapy
PET
[18F]FDG
Cohenet al. (1992)
7 SAD/38 HC
Lower metabolic rates with or without light treatment in SAD
Goyeret al. (1992)
9 summer SAD/45 HC
Altered glucose metabolism in orbital frontal cortex and left inferior parietal lobule in SAD
As mentioned before, SPECT studies using the non-selective radioligand [123I]ß-CIT revealed contradictory results with respect to seasonal effects on SERT binding (Neumeister et al. 2000; Ruhe et al. 2009). A PET study by Praschak-Rieder et al. conducted in a larger group of healthy drug-naïve subjects (n = 88) (Praschak-Rieder et al. 2008) was able to demonstrate a considerable effect of season on SERT by using the specific SERT radioligand [11C]3-amino-4-(2-dimethylaminomethyl-phenylsulfanyl)benzonitrile ([11C]DASB) and PET. This study revealed high [11C]DASB binding potential (BPND) values in autumn and winter in six different predefined regions of interest (ROI). A uniform decrease of regional BPND values was found in spring and summer (Fig. 8.1). Peak differences in [11C]DASB BPND values between months with highest and lowest binding potential values as large as 40 %. Furthermore, [11C]DASB BPND values showed a negative correlation with the duration of daily sunshine and day length. In accordance with this study, Kalbitzer et al. (2010) reported a negative correlation of [11C]DASB BPND values and daylight minutes. In the latter study, 54 healthy subjects were investigated using [11C]DASB PET and genotyped for a polymorphism in the promoter region of the SERT gene (5-HTTLPR). Only carriers of 5-HTTLPR s-allele showed significant effects of season in [11C]DASB binding. In contrast, 5-HTTLPR l-allele homozygous subjects did not exhibit seasonal variation of SERT availability. The methodology used in both studies does not allow for differentiation between the influence of daily sunshine and the astronomical photoperiod (daylight minutes) on [11C]DASB binding because both parameters are highly intercorrelated. Although the negative correlation between [11C]DASB binding and duration of daylight was found in both studies, a study by Murthy et al. (2010) did not replicate these findings.
Additionally, effects of season on SERT binding were demonstrated by Buchert and colleagues (2006) using PET and the radioligand trans-1,2,3,5,6,10b-hexahydro-6-[4-(methylthio)-phenyl]pyrrolo-[2,1-a]-isoquinoline ([11C]-(+)McN5652). This study investigated age-related effects on SERT binding in 29 healthy subjects. In line with the results obtained by [11C]DASB PET, SERT binding measured with [11C]-(+)McN5652 PET was higher in winter, while age did not show any effect on SERT availability in this sample.
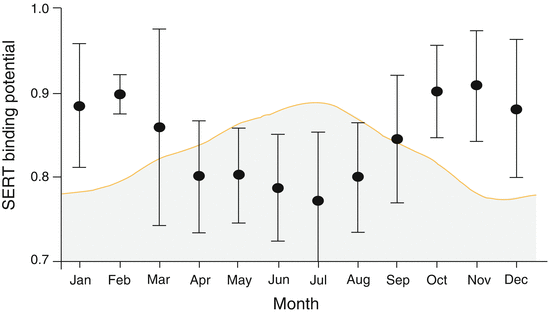
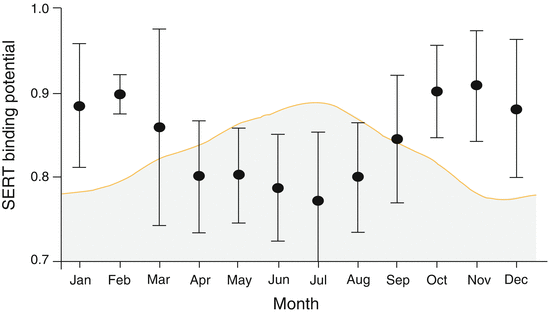
Fig. 8.1
Reciprocal peaks and troughs of serotonin transporter (SERT) binding and duration of sunshine in 88 healthy subjects. Shaded area: duration of sunshine in Toronto, Ontario (range, between 2.4 and 9.2 h a day. SERT BPND measured by the selective SERT radioligand [11C]DASB and positron emission tomography. Circles represent bimonthly moving averages of mean binding potential values in six predefined regions of interest (prefrontal cortex, anterior cingulate, caudate, putamen, thalamus and midbrain). X-axis: calendar months (Modified according to Praschak-Rieder et al. (2012))
In summary, variations in SERT, with higher serotonin transporter availability in times of less light, as shown by the aforementioned studies, may facilitate extracellular serotonin loss during winter, potentially leading to hyposerotonergic symptoms and lower mood. To our knowledge, there are no studies on seasonal variations in SERT binding in patients with SAD. However, the data provided by Ruhe et al. (2009) suggest that there is a similar increase in SERT binding in patients with major depressive disorder in winter.
Recently, a study by Spindelegger et al. (2012) revealed light-dependent alterations of brain serotonin 1A (5-HT1A) receptor binding. Among the different subtypes of serotonin receptors, the inhibitory 5-HT1A receptor has a particular role. Located on GABAergic and glutamatergic neurons in limbic and cortical brain regions, the receptor mediates the inhibition of postsynaptic firing (Varnas et al. 2004). In contrast, 5-HT1A receptors located on serotonergic neuronal somatodendrites inhibit serotonergic cell firing and modulate 5-HT transmitter release into the synaptic cleft. Consequently, these 5-HT1A autoreceptors constitute the decisive factor in a negative auto-regulatory loop of serotonin release (Bundgaard et al. 2006). Alterations in 5-HT1A receptor binding have been reported in several neuropsychiatric disorders such as anxiety (Akimova et al. 2009) and depression (Drevets et al. 2007). One recent animal study provided evidence for seasonal alterations in 5-HT1A receptor expression (Naumenko et al. 2008). The study by Spindelegger et al. investigated 36 healthy drug-naïve subjects by quantifying 5-HT1A BPND using PET and the highly specific 11C-labelled tracer [N-(2-(1-(4-(2-methoxyphenyl)-1-piperazinyl)ethyl))-N-(2-pyridyl)-cyclohexane-carboxamide] (carbonyl-[11C]WAY-100635). Individual exposure to external factors such as global radiation (defined as total of direct solar radiation and diffuse sky radiation received by a unit horizontal surface) correlated with regional 5-HT1A BPND, demonstrating a positive correlation between the accumulated (5 days prior to PET scan) amount of global radiation and 5-HT1A receptor binding. Moreover, this investigation showed a significant difference between the groups of subjects exposed to low versus high amounts of global radiation (see Fig. 8.2). Up to 30 % differences in regional 5-HT1A BPND were found between the different exposure groups.
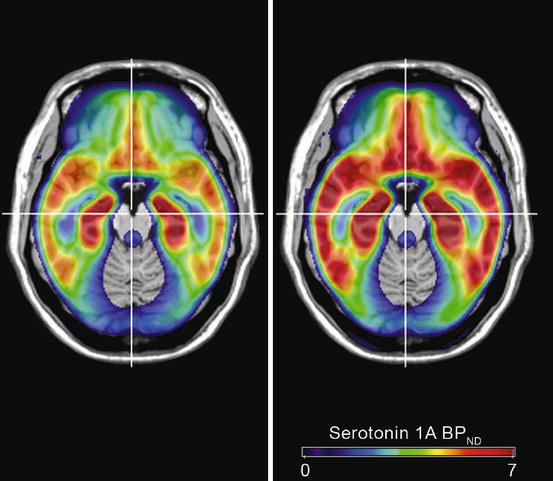
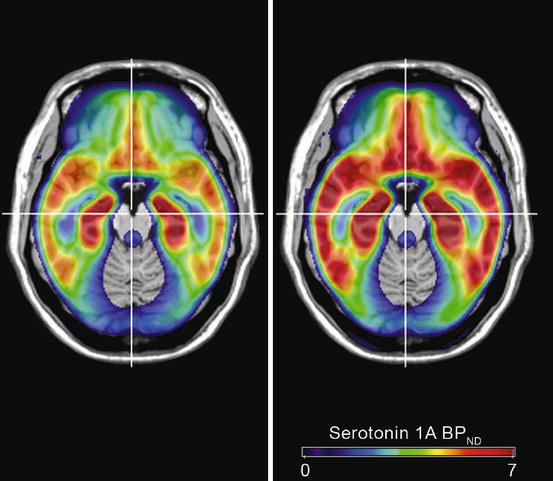
Fig. 8.2
Mean serotonin-1A receptor binding potential (5-HT1A BPND) in subjects exposed to low amounts of global radiation (left) versus subjects exposed to high amounts of global radiation (right) showing 5-HT1A BPND values in the group exposed to a low amount of global radiation, especially in limbic brain regions. Subjects exposed to low amounts of global radiation (n = 22): 5-day accumulation of global radiation was lower than 8,946 J/cm2; subjects exposed to high amounts of global radiation (n = 14): 5-day accumulation of global radiation was higher than 8,946 J/cm2 (Modified according to Spindelegger et al. (2012))
In regard to the effects of season on serotonergic neurotransmission, higher SERT availability in times of less light was revealed in four different studies using SPECT and PET. Furthermore, serotonin receptor binding has been shown to be influenced by external factors such as global radiation. However, these results warrant independent replication. Altogether, these findings underline the importance of research in the field of the effects of season on the serotonergic system, as they provide additional insights into regulatory processes in 5-HT neurotransmission. Consequently, future studies investigating serotonergic target structures such as SERT or serotonin receptors should consider seasonal effects.
Dopamine neurotransmission has been suggested to be regulated in part by photoperiodic and light-dependent rhythms. Dopamine is strongly involved in physiological functions such as motor control, cognition, reward, emotion and memory processes (Dalley and Everitt 2009). Limited evidence for seasonal effects on dopamine neurotransmission is provided by SPECT studies mentioned before (Neumeister et al. 2001; Tsai et al. 2011) and a PET study by Eisenberg et al. (2010) reporting higher striatal fluorine-18-L-dihydroxyphenylalanine ([18F]DOPA) uptake in autumn and winter as compared to spring and summer. Eisenberg and colleagues investigated a large sample of healthy subjects (n = 86) showing higher striatal Ki values in subjects scanned during the fall and winter season. The increased Ki values in the posterior putamen were interpreted as greater presynaptic dopamine synthesis and storage capacity in this region. Based on the resulting higher levels of dopamine in times of less light, these results would be in line with recent findings of lower striatal [123I]IBZM binding in times of less light exposure (Tsai et al. 2011) due to greater competition at postsynaptic D2/3 receptors. Since there is only limited evidence supporting this hypothesis, further investigations are needed to clarify the underlying mechanisms.
8.5 Summary
Seasonal affective disorder and its subsyndromal form constitute a prevalent neuropsychiatric disorder characterised by severe seasonal changes in mood and behaviour. Atypical or reverse vegetative symptoms such as increased sleep duration, hyperphagia and subsequent weight gain are frequent in SAD, and severity of symptoms tends to correlate positively with latitude. During the last decades, only a limited number of studies specifically investigating SAD have been conducted. Apart from brain metabolic changes, monoamine systems in the human brain have been revealed to have a key role in seasonal modulation of behavioural and psychological domains. One of the most consistent findings is the seasonal variation of serotonin transporters with higher availability in winter as shown by four neuroimaging studies using different imaging technologies (Buchert et al. 2006; Kalbitzer et al. 2010; Praschak-Rieder et al. 2008; Ruhe et al. 2009). Other intriguing findings, such as seasonal changes in dopamine neurotransmission (Eisenberg et al. 2010; Tsai et al. 2011) or light-induced alterations in serotonin receptors (Spindelegger et al. 2012), are still awaiting replication. Given the lack of neuroimaging studies in SAD, further research (e.g. seasonal variations in monoamine oxidase activity) is needed to enhance the progress in understanding the molecular background of SAD and seasonal changes in the human brain. Furthermore, knowledge of seasonal effects on brain monoamine function might lead to additional treatment strategies in SAD.
References
Boyce P, Parker G (1988) Seasonal affective disorder in the southern hemisphere. Am J Psychiatry 145:96–99PubMed
Buchert R, Schulze O, Wilke F et al (2006) Is correction for age necessary in SPECT or PET of the central serotonin transporter in young, healthy adults? J Nucl Med 47:38–42PubMed
Campbell S, Macqueen G (2004) The role of the hippocampus in the pathophysiology of major depression. J Psychiatry Neurosci 29:417–426PubMedCentralPubMed
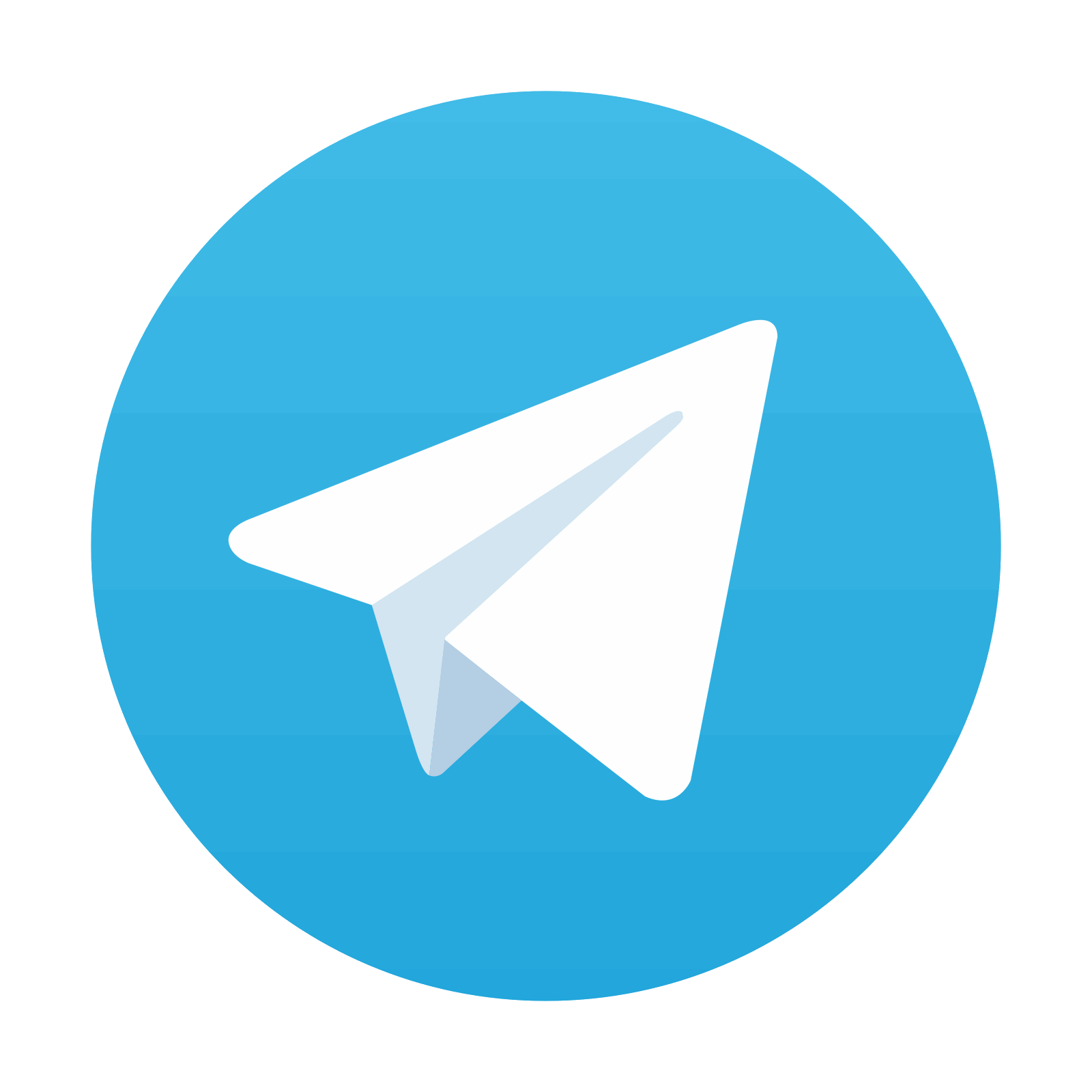
Stay updated, free articles. Join our Telegram channel

Full access? Get Clinical Tree
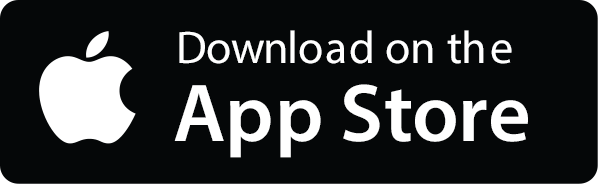
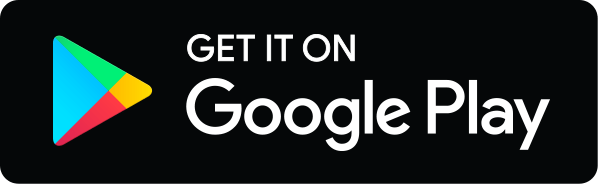