Fig. 33.1
Incidence of pediatric cancers. Age-adjusted and age-specific SEER cancer incidence rates, 2008–2012, all races, male and female, ages 0–19. (Created from data in Howlader N, Noone AM, Krapcho M, Garshell J, Miller D, Altekruse SF et al. Childhood Cancer by the ICCC, Table 29.1: Incidence Rates by Age at Diagnosis: Age-Adjusted and Age-Specific SEER Cancer Incidence Rates, 2008–2012. By International Classification of Childhood Cancer (ICCC) Group and Subgroup and Age at Diagnosis (including myelodysplastic syndromes and Group III benign brain/CNS tumors. All Races, Males and Females. In: SEER Cancer Statistics Review, 1975–2012. National Cancer Institute, Bethesda, MD. 2015. https://seer.cancer.gov/archive/csr/1975_2012/browse_csr.php?sectionSEL=29&pageSEL=sect_29_table.01.html)
Despite an increasing incidence in pediatric cancers (13 per 100,000 in 1975 compared to 17.7 per 100,000 in 2012), there has been an overall decline in cancer mortality of approximately 50% over the same time period [3]. However, despite an equal increase in incidence of brain tumors, the decrease in mortality was only 20% between 1975 and 2012. The apparent increase in pediatric cancers may be, in part, related to earlier identification, increased access to care, and wider availability of advanced imaging and other diagnostic techniques. Nearly two-thirds of children diagnosed with cancer in the mid-1980s are now 20-year survivors of their disease [4], and these numbers are expected to increase as advancements continue in detection and treatment options. As survival continues to improve, the detrimental consequences of treatment on the nervous system, in particular the developing nervous system, are now better appreciated. Precise frequencies and attribution of these effects to the underlying tumor or its treatment are often quite difficult to determine.
As with adults, the incidence of neurologic complications from systemic cancer in children has been increasing. This may be partly due to the better overall recognition of these types of issues, but is also likely a reflection of improved overall survival, particularly in the pediatric population. In the adult population, neurologic effects have been well documented; it is estimated that as many as two-thirds of patients with cancer develop some type of neurological problem during the course of their illness and therapy [5–7]. Neurologic complications of childhood solid malignant tumors have been increasingly described over the past decade, with exact incidence difficult to determine, although the rate may be as high as one-third of patients [8–10]. In general, the focus of prior studies has been on metastatic disease in children [11–13].
As is well appreciated, children are not “mini-adults.” Cancer presentation, manifestations, response to therapies and late effects often differ in children compared to the adult population. Rather than focusing on a particular tumor subtype in children, as has been done for the adult malignancies, this chapter will focus on the unique aspects of pediatric cancer in general. In children as with adults, these complications can be divided into direct tumor-related effects including metastases and paraneoplastic disorders, as well as indirect treatment-related effects from chemotherapy, surgery, cranial irradiation and stem cell transplant (Table 33.1).
Table 33.1
Neurologic complications of cancer in children
Direct, cancer-related toxicities | Treatment-related/iatrogenic |
Local compression | Headache |
Metastatic disease | Seizure |
Leptomeningeal carcinomatosis | Neuropathy |
Paraneoplastic syndromes | Toxic/metabolic encephalopathy |
Indirect, non-metastatic neurologic effects | Cerebral infarction |
Seizure | Drug-specific effects |
Headache/migraine | Radiation complications |
Tic disorder, movement disorder | Central nervous system infections |
Static encephalopathy | Effects of stem cell transplantation |
Direct Neurologic Effects
Brain Metastases
Metastases of systemic cancers to the brain are the most common intracranial tumor in adults, with 15–40% of patients with advanced cancer developing CNS involvement [14–17]. By contrast, central nervous system metastases are much less common in most extracranial pediatric solid tumors, with an estimated 1.5–2.5% incidence in clinical series and 6–13% in autopsy series [12, 18–22]. In adults, the most common primary cancers that metastasize to the brain are lung, breast, melanoma, renal and colorectal. In children, CNS seeding is common in leukemia, but rarer in solid extracranial primary tumors, with the most common being adrenal/renal tumors and sarcomas (Table 33.2) [12, 13, 19, 21, 23]. Similar to cancer incidence in general, the incidence of metastases in pediatric populations seems to be increasing, perhaps due to more effective treatments of the primary cancer, prolonged survival of patients and improvement in neuroimaging techniques. However, most studies are small and there remains debate as to whether routine surveillance for brain metastases is warranted in all patients [24].
Table 33.2
Solid tumors that lead to brain metastases in children
Primary tumor type | Frequency (range) (%) |
---|---|
Osteosarcoma | 5–13 |
Ewing sarcoma | 0–17 |
Soft tissue sarcoma | 2–14 |
Wilms tumor | 1–13 |
Germ cell tumor | 0–50 |
Neuroblastoma | 0–8 |
Other | 2–19 |
Because of the relative rarity of brain metastases in children, information regarding presenting symptoms, pattern of spread, management and further prognosis is quite limited. Children are thought to have more rapid onset of neurologic manifestations compared with adults, perhaps because of a higher proliferative rate of pediatric malignancies [21]. Symptoms are dependent on location of disease and can result from increased intracranial pressure, local compression or disturbance of the tumor microenvironment. The most commonly reported symptoms include headache, nausea, vomiting, hemiparesis, cranial nerve palsies, mental status changes and seizures, the latter with an incidence ranging from 8 to 60% [8, 19, 21].
Patterns of metastatic spread differ by primary tumor site. Pediatric patients who have solid tumors with pulmonary metastases are more likely to have brain metastases, suggesting a hematogenous spread of tumor emboli from the pulmonary metastases [19, 20]. In neuroblastoma, by contrast, metastases are thought to arise from adjacent bone metastases, and in these cases, local invasion of the skull or brain parenchyma and spinal metastases are seen, rather than isolated parenchymal lesions [21, 24]. Additionally, patients with acute myeloid leukemia (AML) can get chloromas (granulocytic sarcomas), which are a subset of parenchymal metastases defined as an extramedullary manifestation of AML in any organ including the brain [25].
Pediatric brain metastases are most often solitary and supratentorial, and by the time CNS involvement is discovered, prognosis tends to be poor despite aggressive therapy. In most cases, surgical extirpation is not attempted, but over time potential treatments have expanded to include stereotactic radiosurgery, whole brain radiation therapy, chemotherapy and targeted agents [22, 26]. In one recent study, patients with Wilms tumor had the longest survival after the diagnosis of CNS metastasis, yet the 1-year survival rates remain approximately 50%. Independent of underlying pathology or treatment, studies report a median survival of 1–12 months after diagnosis of CNS metastases; even with control of brain disease, the majority of patients die of other systemic disease [8, 12, 18–21, 23, 24].
Leptomeningeal and Spinal Metastatic Disease
Leptomeningeal metastases are found most frequently in children with leukemia or primary CNS malignancy [27–29]. Prior to the use of prophylactic CNS therapy, nearly 50% of children with leukemia had CNS disease, which was the major cause of treatment failure, even after bone marrow transplantation [28, 30]. Now, fewer than 10% of patients have CNS leukemia; it is these patients at highest risk who are treated with a combination of intrathecal methotrexate, with or without cytarabine and dexamethasone, and craniospinal irradiation [28]. Leptomeningeal lymphoma is more common in those patients with concurrent disease of the bone marrow [29, 31–33]. Solid tumors can have a leptomeningeal component, either at presentation, as an isolated event, or associated with local disease progression. This is seen most frequently with retinoblastoma [34], neuroblastoma [35–37], rhabdomyosarcoma [38], melanoma [39, 40], and Ewing’s sarcoma [41, 42], and most commonly with advanced systemic disease.
Diagnosis of leptomeningeal disease is confirmed with sampling of cerebrospinal fluid and imaging studies. A negative cytology does not preclude the diagnosis; in adults, initial CSF was positive in 55–70%, but the yield increases to 80–92% with repeated CSF sampling [31, 43]. The diagnosis of CSF leukemia requires definable blasts in the CSF, with a stratification based on number of leukocytes and blasts detected. For germ cell tumors, elevated tumor markers such as alpha fetoprotein and human chorionic gonadotropin may support the diagnosis of leptomeningeal metastases, along with simultaneous serum marker studies. Other potential clues include elevated opening pressure, decreased glucose and increased protein. Imaging studies may be helpful to show bulky disease and to assess CSF flow dynamics.
Leptomeningeal disease and spinal cord compression are both thought to be more treatable and have a better prognosis in children than in adults. Leptomeningeal disease can be bulky, nodular and more focal in nature or have more widespread and diffuse involvement. The most frequent mode of metastases is to the epidural or subarachnoid space or by metastatic spread to the cord parenchyma. Presenting features, therefore, reflect the pattern of involvement and may include both non-specific and focal neurologic symptoms; these symptoms can be the presentation of underlying malignancy in children, either with or without concurrent symptoms of the underlying neoplasm [8, 44]. Patients may also be asymptomatic clinically, with the diagnosis made by surveillance of CSF cytology and/or neuroimaging studies [27]. The most common presentation of leptomeningeal disease is from symptoms of increased intracranial pressure, including headache, nausea and vomiting. There may also be involvement of the cranial nerves or spinal cord involvement with weakness, sensory loss, pain, ataxia or paraparesis. The most common non-traumatic cause of paraparesis in children is neoplastic spinal cord compression [45]. Symptoms of spinal cord compression often include back pain, lower extremity weakness, paresthesias and urinary retention [8, 18, 27, 46]. Occasionally, leptomeningeal disease can mimic a mass lesion in the cerebellum by infiltrating cerebellar pathways [47]. For leukemia, the most common neurologic symptoms overall include weakness and back pain, even without leptomeningeal infiltration [44]. Lymphoma, by contrast, is more likely to show direct meningeal infiltration with spinal cord compression and/or cranial neuropathy [48]. Neuroblastoma may present with spinal cord compression due to epidural spinal metastases.
Treatment for spinal cord compression can include a combination of decompressive laminectomy and/or high-dose steroids followed by therapeutic irradiation and intrathecal and/or systemic chemotherapy. In children, laminectomy is avoided as much as possible, particularly in young patients, because of concerns for development of anterior subluxation, kyphosis or scoliosis; surgery is reserved for cases with rapid neurologic deterioration [49]. Children with neuroblastoma producing spinal cord compression from local disease are often treated with chemotherapy alone (Fig. 33.2).


Fig. 33.2
Sagittal magnetic resonance imaging of a 3-day-old infant, who presented with paraparesis and found to have spinal neuroblastoma and spinal cord compression. He was treated with chemotherapy alone. At 15 months, he now is crawling and standing with stander device
Paraneoplastic Disorders
Paraneoplastic neurological syndromes , which are thought to be autoimmune phenomena associated with specific cancers and marked by specific autoantibodies, are rare complications of malignancy that are seen much more frequently in the adult population. These syndromes affect <1% of all patients with cancer and often occur while the primary tumor is small and otherwise asymptomatic [50–52].
Paraneoplastic syndromes can be broken down into four categories based on the mechanism of action of the autoantibodies [53]. First are the neuromuscular paraneoplastic disorders including myasthenia gravis, Lambert-Eaton myasthenic syndrome, autoimmune autonomic neuropathy, Isaacs syndrome (acquired neuromyotonia) and inflammatory myopathies (polymyositis, dermatomyositis). In these cases, autoantibodies to the NMJ or peripheral nerve membrane have direct physiologic effects and generally respond to immunosuppressive treatment (steroids, IVIG, plasmapheresis). Second are the classical paraneoplastic disorders with antibodies that mark a cell-mediated immune response, but which do not directly cause disease and thus have a poor response to immunotherapy; these include the anti-Hu, anti-Ma/Ta and anti-Yo syndromes that can lead to encephalomyelitis, limbic encephalitis or cerebellar degeneration, respectively. Third are autoantibodies to intracellular synaptic and neuronal surface proteins, such as anti-GAD65, which may be directly pathogenic or mark a T-cell response, as there is often a mixed response to immunotherapy. And finally, there are autoantibodies to extracellular surface epitopes of CNS synaptic and other neuronal membrane proteins that do have direct effects, such as anti-NMDA receptor syndrome and many others that are actively being identified.
Although paraneoplastic disorders are less common in children, as identification of antigenic neuronal membrane proteins continues to progress, the incidence will likely increase. The classic example in pediatric cancer is opsoclonus-myoclonus syndrome (OMS) , which is commonly associated with neuroblastoma. OMS is defined by the classical acute onset of rapid and chaotic eye movements, myoclonic jerks and appendicular and axial ataxia. It can also be associated with behavioral changes and emotional lability. OMS is associated with underlying neuroblastoma in more than one half of cases and occurs in 2–3% of all children with neuroblastoma [54, 55]. A thorough search for neuroblastoma is necessary in all patients with OMS. Despite continued research, there does not appear to be a universal autoantibody detectable, although an autoimmune pathogenesis has been suspected, particularly with the favorable response to steroids and intravenous immunoglobulin [56]. Children with OMS are more likely to have serum antineuronal antibodies than other children with neuroblastoma, but the anti-Ri antibodies detected in adult cases of this syndrome are less frequently identified in children [57].
In at least half of these cases, OMS is the first presenting symptom of the neuroblastoma; the presence of OMS is often thought to portend a more favorable prognosis, with almost all being small, stage I–II, non-MYCN amplified tumors. Despite a better oncologic prognosis, there can be acute as well as chronic neurologic effects [54, 55, 58–60]. Personality changes, developmental regression with loss of speech and language, and motor deficits may remain significant, and many children will continue to experience severe learning and language deficits [61]. Surgical removal of the tumor in addition to prompt and strong immunosuppression remains the mainstay of therapy; however, very few children actually receive early therapy [62]. There was a recently completed Children’s Oncology Group randomized phase III trial for patients with OMS and neuroblastoma to determine the efficacy of immunosuppressive therapy with cyclophosphamide, prednisone and IVIG in treating OMS, with final results still pending (NCT00033293) [63].
A second paraneoplastic neurologic syndrome, paraneoplastic limbic encephalitis (PLE) , has been better defined over the past decade. PLE is thought to be not a single entity, but rather a subset of autoimmune disorders with antineuronal antibodies that primarily affect the limbic system [64]. There is an ever-increasing number of antineuronal antibodies identified, the most common in pediatrics being the anti-NMDA receptor syndrome, seen most frequently in young women with ovarian teratomas. The illness often begins with a viral prodrome, followed by behavioral or cognitive changes, then variably with seizures, movement disorders, eye movement abnormalities and/or autonomic dysfunction. Without treatment, the illness often culminates in coma, but is often improved with immunomodulators [65]. Additionally, antibodies to the metabotropic glutamate receptor 5 (mGluR5) have been described in patients with Hodgkin lymphoma who experience the “Ophelia syndrome,” a constellation of altered mental status, insomnia, short-term memory loss, depression and cognitive impairment [66, 67].
Paraneoplastic neurologic syndromes are clinically heterogeneous and can be quite difficult both to recognize and to diagnose; more research is needed to further understand their pathophysiology and efficacy of treatments with immunotherapy, which appears to depend on the underlying mechanism of action of the specific autoantibody.
Indirect and Treatment-Related Neurologic Complications
Treatment of pediatric cancer is generally tailored to the underlying tumor type and location, as in adults. While these treatments are critical, from surgery to chemotherapy to radiation to stem cell transplant, they often have neurologic side effects that can be long lasting. These complications include headaches, seizures, altered mental status, cerebral infarctions, peripheral neuropathies, sleep disturbances, cognitive effects and secondary tumors. Chemotherapy-related effects in children are in many cases similar to those experienced and reported in adults. The incidence of neurotoxicity from acute lymphoblastic leukemia (ALL) therapy is thought to range from 5% to 18% [68–70]. In addition to toxicities of chemotherapy, radiation and stem cell transplant have their own unique concerns. This section will review some general complications of cancer treatment in children and will discuss several chemotherapeutic agents and their more common neurotoxic effects (Table 33.3).
Table 33.3
Commonly used chemotherapeutic drugs, frequency and type of neurologic side effects
Drug | Common, >10% | Uncommon, <10% | Rare, <1% |
---|---|---|---|
Methotrexate | Leukoencephalopathy, esp. with radiotherapy | Myelitis, arachnoiditis | Seizure |
Cytarabine | Ataxia, arachnoiditis | ||
Vincristine | Peripheral/autonomic neuropathy | Seizures | |
Asparaginase | Mental status Δ | Sinus thrombosis | |
Corticosteroids | Myopathy, tremor, behavioral Δ | Psychosis, seizures, neuropathy | |
Cisplatin/carboplatin | Hearing loss | Peripheral neuropathy, seizure | |
Cyclosporine | Tremor | Seizure, Leukoencephalopathy | |
Thalidomide | Neuropathy |
Headaches
Headaches in children are a common neurologic complaint and the most common cause for neurologic consultation in children with cancer; the difficulty lies in determining the underlying etiology (Table 33.4) [71]. Typical causes of headache can be seen, such as migraine or tension-type headaches. Of 147 childhood ALL survivors in at least 1-year of remission and 5 years from diagnosis, more than 40% had new-onset headaches; of those, approximately 1/3 were migraine, 1/3 were tension-type headache and 1/3 were mixed [72].
Table 33.4
Most common reasons for neurologic assessment in children with systemic cancer
Reason for neurologic evaluation | % complaints |
---|---|
Headache | 34.9 |
Back/neck pain | 25 |
Limb pain/sensory change | 12 |
Extremity weakness | 7.1 |
Seizure | 7 |
Altered mental status | 8.4 |
Visual complaints/diplopia | 6.5 |
Treatment-specific causes of headache can occur, such as adverse drug reactions, post-LP headaches and posterior reversible encephalopathy syndrome (PRES). Common drug effects include fever, anemia or pseudotumor cerebri. Low pressure headaches after lumbar puncture, with or without intrathecal chemotherapy, are frequent with an incidence that ranges from 8% overall [73] to as much as 50% in the adolescent population [74]. PRES is seen particularly in the setting of hypertension, induction chemotherapy or treatment with immunosuppressive agents (e.g., cyclosporine, tacrolimus and corticosteroids).
Complications of the disease itself can cause headaches, from metastases to infection to vascular injury (arterial occlusion, venous sinus thrombosis, hemorrhage). For systemic cancers, brain metastases are the most common structural cause of headache, followed by infections such as abscess and meningitis, and less frequently by intracranial hemorrhage [71]. The significant immunosuppression induced by many cancer treatments makes patients susceptible to many microorganisms, with life-threatening infections mainly caused by viruses (herpesvirus, VZV, CMV) or fungi. Structural disease should be excluded even in the absence of localizing signs, as 25% of children with systemic cancer and headache have an underlying structural lesion [75].
Seizures
The second most frequent reason for neurologic consultation is seizures, which occur in children with both solid tumors and hematologic malignancies, as well as children undergoing bone marrow transplantation. Seizures in children are more frequent than in adults and can result from either tumor- or treatment-related toxicity. Seizure risk may be increased in those patients who are known to have central nervous system involvement. All types of seizures may be observed in children who are treated with chemotherapy. Seizures without evidence for mass lesion are reported in 8–10% of children with leukemia and lymphoma [76, 77], though it is estimated that as many as 50% of children with solid tumors who experience a seizure have an underlying structural abnormality [71]. A thorough evaluation must be performed to search for intracranial lesions, infection, encephalopathy and stroke as potential etiologies.
Primary CNS causes of seizure include primary brain tumor, parenchymal or leptomeningeal metastases, ischemic or hemorrhagic stroke, PRES, leukoencephalopathy or intracranial infection (meningitis, cerebritis, abscess). At least 15% of brain tumor patients will have seizures, which may result from disruption of peritumoral environment, tumoral edema, adjacent scar, local hypoxia, acidosis or metabolic changes that affect neuronal excitability and epileptogenesis in tumor cells [78]. In brain tumor patients, several factors affect the incidence of seizures, including age, location, histology and grade of the tumor. Low-grade and slow-growing tumors tend to be more epileptogenic. Cortical lesions in the temporal, frontal and parietal lobes are more often correlated with seizures than tumors in the occipital lobe or posterior fossa [79].
Treatment-related causes of seizures include toxic-metabolic effects of chemotherapy (cyclosporine, asparaginase, imipenem), medication toxicity or withdrawal, radiation injury, renal or liver failure, latent non-CNS infection, and electrolyte abnormalities (SIADH, hypoglycemia, hypomagnesemia, hypo- or hypercalcemia) [80]. The strongest association with seizures and chemotherapy has been reported with methotrexate, which is used to treat many pediatric cancers, including ALL, lymphoma and sarcomas. Estimates of seizure frequency suggest that 7–20% of children with ALL experience seizures at some point during the course of therapy [76, 81, 82]. One study of survivors of ALL showed that 13% of children had experienced a seizure at some point during their treatment, and all reported patients except one experienced seizure after intrathecal methotrexate or subcutaneous asparaginase [77]. Other agents known to lower seizure threshold include cisplatin and vincristine, as both of these agents can pass through the blood-brain barrier and can secondarily induce seizures secondary to electrolyte disturbances from hypocalcemia, hypomagnesemia or hyponatremia.
Seizure prophylaxis must be carefully considered to determine whether medications are needed and, if so, the specific choice. The choice of anticonvulsant may be challenging, particularly as many of the more traditional anticonvulsants, such as phenytoin, carbamazepine and phenobarbital, can affect metabolism of chemotherapeutic drugs by inducing increased activity of the cytochrome p450 system [83, 84]. This may result in decreased tumor therapy efficacy, decreased seizure control and/or unexpected toxicity. Newer AEDs, especially those without significant drug–drug interactions, are becoming accepted as first-line seizure treatment in children who require ongoing seizure prophylaxis [85, 86].
Change in Mental Status
Alteration of mental status is a relatively common occurrence in children undergoing chemotherapy and has been reported in as many as 11% of children during the course of treatment [87]. Similar to headache and seizure, altered mental status can have multiple etiologies in patients with cancer, both disease- and treatment-related. As in the adult population, the underlying cause of mental status changes in children is toxic-metabolic encephalopathy in two-thirds of the cases and structural causes in the other one-third [71]. Many different agents are known to cause changes in level of alertness and/or somnolence in children, including asparaginase, cisplatin, ifosfamide, methotrexate (including intrathecal), cytarabine, cyclosporine, etoposide and vincristine. Independent of chemotherapy, opioids, benzodiazepines, H2-blockers, antihistamines and antibiotics may cause delirium as well. Although medications may ultimately be the culprit, it is important to eliminate other potential causes, such as seizure, cerebrovascular event, infection, hemorrhage or metabolic derangement, so as to address potentially treatable sources.
Cerebral Infarction
Stroke is becoming an increasingly recognized complication in pediatric cancer patients. As in adults, cerebral infarction in childhood cancer patients may be ischemic or hemorrhagic. Etiologies range from disease-related complications of hypercoagulability, disseminated intravascular coagulation, thrombocytopenia or hemorrhagic metastases, to treatment-related complications of chemotherapy (venous sinus thrombosis, meningitis) or radiation (small and large vessel vasculopathy) [88, 89]. Stroke manifestations may be age-related. In the younger child, presentation may be more insidious with irritability, altered level of alertness and seizures while older children often report headaches, focal deficits such as visual change, speech impairment, weakness and seizures [90, 91].
Ischemic stroke is seen secondary to direct thrombosis, septic emboli, non-septic embolic disease, venous sinus thrombosis or disseminated intravascular coagulation. One large study of children with leukemia reported a 0.47% prevalence of ischemic stroke, all of which were sinovenous thrombosis (Fig. 33.3) [92]. Despite a relatively high frequency of elevated white blood cell counts and decreased platelets in children with cancer, the overall rate of strokes is thought to be quite low as a result of these risk factors. Strokes from chemotherapy itself have been more commonly associated with asparaginase and methotrexate [87, 93, 94]. In the pediatric population, neurologic outcome after sinovenous thrombosis was related to underlying clinical features such as seizures, impaired level of consciousness, coincident intracranial hemorrhage, deep venous location, lack of antithrombotic therapy and young age, less than six years [95–98].


Fig. 33.3
Magnetic resonance venogram in a 4-year-old child treated with L-asparaginase for acute leukemia who experienced sagittal sinus thrombosis and subsequent venous infarction
Hemorrhagic stroke is a serious risk in acute leukemia patients, typically due coagulation abnormalities and most often occurring during induction therapy. Intracranial hemorrhage is reported to be the second leading cause of mortality in AML patients, accounting for up to 70% in some series [99]. Treatment with L-asparaginase is an additional risk factor. Superficial siderosis is a less common complication in CNS tumor patients, with deposition of hemosiderin in the subarachnoid space leading to sensorineural deafness, cerebellar ataxia and myelopathy due to recurrent hemorrhage [100].
Radiation therapy is a primary risk factor for cerebral infarction [101]. Patients with hematologic malignancies and primary brain tumors who receive radiation therapy are at high risk, generally in a dose-dependent manner [102]. Location of radiation is another risk factor for cerebral infarction, with mantle radiation in Hodgkin’s disease (thought to trigger cardiac valvular dysfunction and carotid artery disease) and radiation to the Circle of Willis in suprasellar tumors both increasing risk [103]. Radiation contributes to weakening of the microvasculature within the blood-brain barrier and increasing capillary permeability, leading to increased inflammation and thrombosis of small vessels [104]. Inflammation also leads to premature atherosclerosis, intimal fibrosis and macrophage activation that can lead to stenosis or thrombus [105]. Damaged vasculature can lead to abnormal dilation and tortuosity, causing vascular malformation, aneurysm or moyamoya syndrome, and this risk is higher in children with neurofibromatosis type 1 [106, 107]. These changes can result in seizures, headaches and potentially life-threatening hemorrhage. Hemorrhagic strokes presenting as delayed hemorrhagic radiation vasculopathy, separate from tumor recurrence or secondary tumor and unrelated to treatment dose, have been reported after radiation alone or radiation in combination with systemic chemotherapy in children [108].
Motor Deficits/Neuropathy
Pediatric oncology patients experience motor deficits for a variety of reasons, with localization potentially anywhere along the neural axis. Motor deficits can occur due to primary tumor or metastatic compression of fibers along the motor strip, supplementary motor areas or corticospinal tract. In the brainstem, cranial nerve deficits may also be observed, particularly in patients who already have some cranial nerve dysfunction.
In the spinal cord, acute cord compression is a neurologic emergency in children as in adults and must be investigated emergently for potentially reversible causes. Spinal cord compression occurs in 3–5% of children with cancer, most commonly if the tumor involves epidural or subarachnoid space [109]. Childhood tumors implicated include neuroblastoma, sarcomas, non-Hodgkin lymphoma and germ cell tumors. Symptoms include motor or sensory deficits, sphincter abnormalities or back pain. Steroids should be given immediately, followed by MRI to confirm diagnosis, then more definitive treatment with surgical decompression, radiation and/or chemotherapy. Some patients have persistent neurologic deficits, with severity of deficits at time of diagnosis most predictive of long-term symptoms.
The most well-studied nerve injury in cancer patients is chemotherapy-induced peripheral neuropathy (CIPN), which is known to damage axon, cell body or myelin depending on the mechanism of action. CIPN can be difficult to assess in children due to limited verbal expression and vocabulary to express specific symptoms. Nerve damage is generally length-dependent, with longest axons displaying abnormalities first. Risk factors include higher dose of chemotherapy with specific agents, intensity of treatment schedule, multimodal treatment, preexisting neuropathy and genetic predisposition (e.g., decreased expression of CYP3A5) [110]. Some of the more common agents used in pediatrics include vincristine, a mitotic inhibitor that inhibits tubulin polymerization and can cause sensory, motor and autonomic neuropathies as well as platinum-based agents that bind with DNA strands and induce apoptotic cell death [111]. Platinum drugs more commonly lead to high frequency sensorineural hearing loss; ototoxic effects are related to total dose and often synergistic in patients who have also received cranial irradiation. The underlying mechanism is thought to be related to permanent damage to the mitochondria of the hair cells. Taxanes and epothilones, microtubule-stabilizing agents, can contribute to peripheral neuropathy by inhibition of axonal transport [110]. Finally, the newer antibody and enzyme inhibitor therapies are less well studied, but agents such as bortezomib appear to affect dorsal root ganglion cells, leading to moderate to severe neuropathic pain [112].
Damage to the neuromuscular junction and muscle itself can also occur, due to treatment toxicity or paraneoplastic syndromes. Residual deficits are present in many long-term cancer survivors, with one study documenting sensation, vibration and reflex deficits in adult survivors of childhood ALL treated with vincristine >20 years after completion of treatment [113]. Studies are being conducted to analyze agents with potential neuroprotective factors in children receiving these treatments. In addition to physical and occupational rehabilitation, treatment of neuropathic pain with gabapentin, pregabalin and various antidepressants are being studied in children.
Sleep Disorders
Sleep problems are common in the general population, and even more so in children and adolescents with chronic medical conditions, including cancer. Like many of the other comorbidities described in this chapter, sleep dysfunction can be due to specific cancer-related factors (direct brain injury, chemotherapy, cranial radiation, pain, seizures, endocrinopathies, medications) or other genetic and environmental factors including familial predisposition, stress and other mental health problems [114].
The specific sleep disturbances seen are varied and include symptoms from cancer-related fatigue and excessive daytime sleepiness (EDS) to insomnia, parasomnias and restless leg syndrome [115]. There are limited studies of the prevalence of sleep problems in children with cancer, but the Childhood Cancer Survivorship Study (CCSS) found that 19% of adult survivors were in the most fatigued range, 16.7% reported disrupted sleep and 14% reported EDS [116].
Sleep disorders are particularly common in patients with CNS tumors and metastases; one study showed 46% of children with a neoplasm of the hypothalamus, thalamus or brainstem had sleep-disordered breathing (obstructive sleep apnea, central sleep apnea) and 80% had EDS [117]. In children with leukemia, insomnia was the most common sleep problem identified (39%), especially in patients receiving high-dose steroid treatment. Sleep irregularities are not solely a result of obstructive sleep apnea; for example, in craniopharyngiomas, direct injury to the hypothalamus can result in disruption of circadian rhythms [118].
Sleep dysfunction is associated with reduced quality of life in pediatric cancer patients and should be addressed regularly, and if indicated, objectively measured with a validated sleep scale, actigraphy, multiple sleep latency test and/or nocturnal polysomnography [119, 120]. For treatment, lifestyle modifications should be trialed including weight loss, exercise, avoiding daytime naps and treatment of thyroid hormone or cortisol deficiency. Pharmacotherapy options include melatonin at bedtime or stimulants in cases of EDS related to delayed sleep phase syndrome [121].
Cognitive Effects
Neurocognitive late effects are a multidimensional, globally impacting and potentially modifiable problem in survivors of childhood cancer. The nature and severity of deficits depend on the patient’s neurodevelopmental stage at the time of diagnosis and treatment. One study estimated that 40–50% of acute lymphoblastic leukemia survivors and 70–80% of brain tumor survivors would require special education services during their school years [122]. The cognitive domains most typically affected include memory, attention, processing speed and executive functioning, in addition to motor skills and psychosocial functioning [123]. Risk factors include young age at time of treatment, female gender and dose intensity of treatment.
In a study of nearly 3000 adolescent and young adult survivors of cancer (ages 11–21 years at diagnosis) compared with siblings, the patients had significantly increased rates of depression and anxiety and more problems with task efficiency, emotional regulation and memory [124]. Brain tumor survivors are especially vulnerable to neurocognitive dysfunction given the aggressive CNS-directed therapies required for treatment of their tumors. Deficits can be due to direct brain injury from the tumor (type, size, location), its treatment (surgery, radiation or systemic effects of chemotherapy), or disease-related complications; all of which are further modified by the surrounding environment and psychosocial factors of the child [125].
Craniospinal radiation is particularly injurious, with destruction of white matter tracts and/or failure to develop white matter at a rate consistent with the developmental stage of the child implicated as the mechanism by which cognitive deficits occur [126]. A unique deficit described in children treated for posterior fossa tumors is cerebellar mutism, a typically transient syndrome of paucity of speech often accompanied by emotional and behavioral dysregulation that evolves after cerebellar injury and occurs in approximately 25% of children following posterior fossa tumor surgery [127].
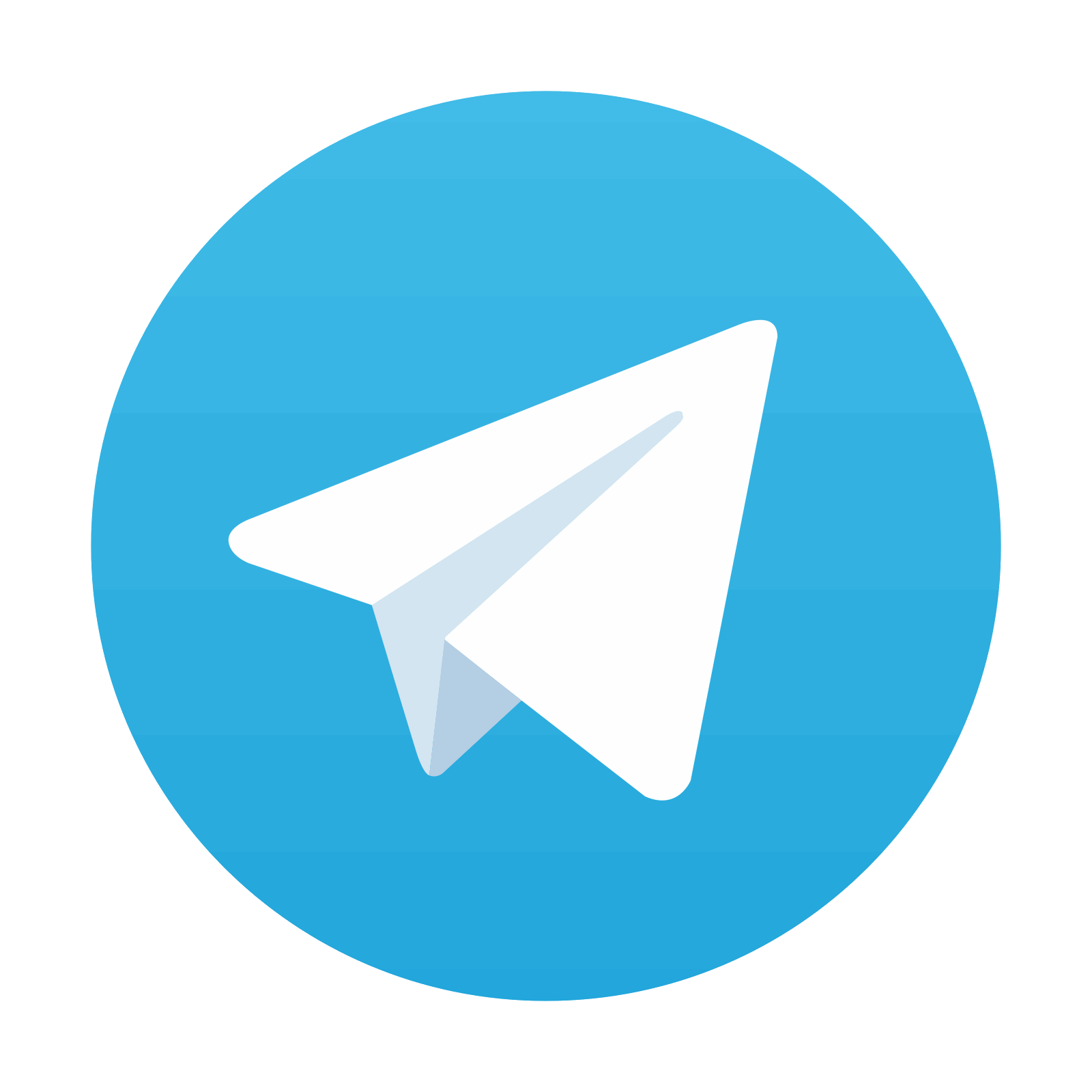
Stay updated, free articles. Join our Telegram channel

Full access? Get Clinical Tree
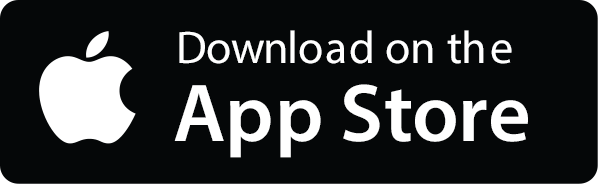
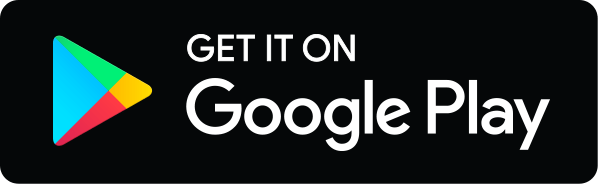