Life-threatening neurologic illness may be caused by a primary disorder affecting any region of the neuraxis or may occur as a consequence of a systemic disorder such as hepatic failure, multisystem organ failure, or cardiac arrest (Table 33-1). Neurologic critical care focuses on preservation of neurologic tissue and prevention of secondary brain injury caused by ischemia, hemorrhage, edema, herniation, and elevated intracranial pressure (ICP). Management of other organ systems proceeds concurrently and may need to be modified in order to maintain the overall focus on neurologic issues.
LOCALIZATION ALONG NEUROAXIS | SYNDROME |
---|---|
Central Nervous System | |
Brain: Cerebral hemispheres | Global encephalopathy Delirium Sepsis Organ failure—hepatic, renal Medication related—sedatives, hypnotics, analgesics, H2 blockers, antihypertensives Drug overdose Electrolyte disturbance—hyponatremia, hypoglycemia Hypotension/hypoperfusion Hypoxia Meningitis Subarachnoid hemorrhage Wernicke’s disease Seizure—postictal or nonconvulsive status Hypertensive encephalopathy Hypothyroidism—myxedema Focal deficits Ischemic stroke Tumor Abscess, subdural empyema Intraparenchymal hemorrhage Subdural/epidural hematoma |
Brainstem/cerebellum | Mass effect and compression Basilar artery thrombosis Intraparenchymal hemorrhage Central pontine myelinolysis Mass effect and compression |
Spinal cord | Disk herniation Epidural hematoma Ischemia—hypotension/embolic Epidural abscess Trauma Myelitis |
Peripheral Nervous System | |
Peripheral nerve Axonal | Critical illness polyneuropathy Neuromuscular blocking agent complications Metabolic disturbances, uremia, hyperglycemia Medication effects—chemotherapeutic, antiretroviral |
Demyelinating | Guillain-Barré syndrome Chronic inflammatory demyelinating polyneuropathy |
Neuromuscular junction | Prolonged effect of neuromuscular blockade Medication effects—aminoglycosides Myasthenia gravis, Lambert-Eaton syndrome, botulism |
Muscle | Critical illness myopathy Cachectic myopathy Acute necrotizing myopathy Thick-filament myopathy Electrolyte disturbances—hypokalemia/hyperkalemia, hypophosphatemia Rhabdomyolysis |
Swelling, or edema, of brain tissue occurs with many types of brain injury. The two principal types of edema are vasogenic and cytotoxic. Vasogenic edema refers to the influx of fluid and solutes into the brain through an incompetent blood-brain barrier (BBB). In the normal cerebral vasculature, endothelial tight junctions associated with astrocytes create an impermeable barrier (the BBB), through which access into the brain interstitium is dependent upon specific transport mechanisms. The BBB may be compromised in ischemia, trauma, infection, and metabolic derangements. Vasogenic edema results from abnormal permeability of the BBB, and typically develops rapidly following injury. Cytotoxic edema results from cellular swelling, membrane breakdown, and ultimately cell death. Clinically significant brain edema usually represents a combination of vasogenic and cytotoxic components. Edema can lead to increased ICP as well as tissue shifts and brain displacement or herniation from focal processes (Chap. 19). These tissue shifts can cause injury by mechanical distention and compression in addition to the ischemia of impaired perfusion consequent to the elevated ICP.
When delivery of substrates, principally oxygen and glucose, is inadequate to sustain cellular function, a series of interrelated biochemical reactions known as the ischemic cascade is initiated (see Fig. 32-2). The release of excitatory amino acids, especially glutamate, leads to influx of calcium and sodium ions, which disrupt cellular homeostasis. An increased intracellular calcium concentration may activate proteases and lipases, which then lead to lipid peroxidation and free radical–mediated cell membrane injury. Cytotoxic edema ensues, and ultimately necrotic cell death and tissue infarction occur. This pathway to irreversible cell death is common to ischemic stroke, global cerebral ischemia, and traumatic brain injury.
Penumbra refers to areas of ischemic brain tissue that have not yet undergone irreversible infarction, implying that these regions are potentially salvageable if ischemia can be reversed. Factors that may exacerbate ischemic brain injury include systemic hypotension and hypoxia, which further reduce substrate delivery to vulnerable brain tissue, and fever, seizures, and hyperglycemia, which can increase cellular metabolism, outstripping compensatory processes. Clinically, these events are known as secondary brain insults because they lead to exacerbation of the primary brain injury. Prevention, identification, and treatment of secondary brain insults are fundamental goals of management.
An alternative pathway of cellular injury is apoptosis. This process implies programmed cell death, which may occur in the setting of ischemic stroke, global cerebral ischemia, traumatic brain injury, and possibly intracerebral hemorrhage. Apoptotic cell death can be distinguished histologically from the necrotic cell death of ischemia and is mediated through a different set of biochemical pathways; apoptotic cell death occurs without cerebral edema and therefore is often not seen on brain imaging. At present, interventions for prevention and treatment of apoptotic cell death remain less well defined than those for ischemia.
Excitotoxicity and mechanisms of cell death are discussed in more detail in Chap. 30.
Brain tissue requires constant perfusion in order to ensure adequate delivery of substrate. The hemodynamic response of the brain has the capacity to preserve perfusion across a wide range of systemic blood pressures. Cerebral perfusion pressure (CPP), defined as the mean systemic arterial pressure (MAP) minus the ICP, provides the driving force for circulation across the capillary beds of the brain. Autoregulation refers to the physiologic response whereby cerebral blood flow (CBF) is regulated via alterations in cerebrovascular resistance in order to maintain perfusion over wide physiologic changes such as neuronal activation or changes in hemodynamic function. If systemic blood pressure drops, cerebral perfusion is preserved through vasodilation of arterioles in the brain; likewise, arteriolar vasoconstriction occurs at high systemic pressures to prevent hyperperfusion, resulting in fairly constant perfusion across a wide range of systemic blood pressures (Fig. 33-1). At the extreme limits of MAP or CPP (high or low), flow becomes directly related to perfusion pressure. These autoregulatory changes occur in the microcirculation and are mediated by vessels below the resolution of those seen on angiography. CBF is also strongly influenced by pH and Paco2. CBF increases with hypercapnia and acidosis and decreases with hypocapnia and alkalosis because of pH related changes in cerebral vascular resistance. This forms the basis for the use of hyperventilation to lower ICP, and this effect on ICP is mediated through a decrease in both CBF and intracranial blood volume. Cerebral autoregulation is a complex process critical to the normal homeostatic functioning of the brain, and this process may be disordered focally and unpredictably in disease states such as traumatic brain injury and severe focal cerebral ischemia.
FIGURE 33-1
Autoregulation of cerebral blood flow (solid line). Cerebral perfusion is constant over a wide range of systemic blood pressure. Perfusion is increased in the setting of hypoxia or hypercarbia. BP, blood pressure; CBF, cerebral blood flow. (Reprinted with permission from HM Shapiro: Anesthesiology 43:447, 1975. Copyright 1975, Lippincott Company.).

The cranial contents consist essentially of brain, cerebrospinal fluid (CSF), and blood. CSF is produced principally in the choroid plexus of each lateral ventricle, exits the brain via the foramens of Luschka and Magendie, and flows over the cortex to be absorbed into the venous system along the superior sagittal sinus. In adults, approximately 150 mL of CSF are contained within the ventricles and surrounding the brain and spinal cord; the cerebral blood volume is also ~150 mL. The bony skull offers excellent protection for the brain but allows little tolerance for additional volume. Significant increases in volume eventually result in increased ICP. Obstruction of CSF outflow, edema of cerebral tissue, or increases in volume from tumor or hematoma may increase ICP. Elevated ICP diminishes cerebral perfusion and can lead to tissue ischemia. Ischemia in turn may lead to vasodilation via autoregulatory mechanisms designed to restore cerebral perfusion. However, vasodilation also increases cerebral blood volume, which in turn then increases ICP, lowers CPP, and provokes further ischemia (Fig. 33-2). This vicious cycle is commonly seen in traumatic brain injury, massive intracerebral hemorrhage, and large hemispheric infarcts with significant tissue shifts.
FIGURE 33-2
Ischemia and vasodilatation. Reduced cerebral perfusion pressure (CPP) leads to increased ischemia, vasodilation, increased intracranial pressure (ICP), and further reductions in CPP, a cycle leading to further neurologic injury. CBV, cerebral blood volume; CMR, cerebral metabolic rate; CSF, cerebrospinal fluid; SABP, systolic arterial blood pressure. (Adapted from MJ Rosner et al: J Neurosurg 83:949, 1995; with permission.)

APPROACH TO THE PATIENT: Severe Central Nervous System Dysfunction
Critically ill patients with severe central nervous system (CNS) dysfunction require rapid evaluation and intervention in order to limit primary and secondary brain injury. Initial neurologic evaluation should be performed concurrent with stabilization of basic respiratory, cardiac, and hemodynamic parameters. Significant barriers may exist to neurologic assessment in the critical care unit, including endotracheal intubation and the use of sedative or paralytic agents to facilitate procedures.
An impaired level of consciousness is common in critically ill patients. The essential first task in assessment is to determine whether the cause of dysfunction is related to a diffuse, usually metabolic, process or whether a focal, usually structural, process is implicated. Examples of diffuse processes include metabolic encephalopathies related to organ failure, drug overdose, or hypoxia-ischemia. Focal processes include ischemic and hemorrhagic stroke and traumatic brain injury, especially with intracranial hematomas. Because these two categories of disorders have fundamentally different causes, treatments, and prognoses, the initial focus is on making this distinction rapidly and accurately. The approach to the comatose patient is discussed in Chap. 19; etiologies are listed in Table 19-1.
Minor focal deficits may be present on the neurologic examination in patients with metabolic encephalopathies. However, the finding of prominent focal signs such as pupillary asymmetry, hemiparesis, gaze palsy, or paraplegia should suggest the possibility of a structural lesion. All patients with a decreased level of consciousness associated with focal findings should undergo an urgent neuroimaging procedure, as should all patients with coma of unknown etiology. Computed tomography (CT) scanning is usually the most appropriate initial study because it can be performed quickly in critically ill patients and demonstrates hemorrhage, hydrocephalus, and intracranial tissue shifts well. Magnetic resonance imaging (MRI) may provide more specific information in some situations, such as acute ischemic stroke (diffusion-weighted imaging [DWI]) and cerebral venous sinus thrombosis (magnetic resonance venography [MRV]). Any suggestion of trauma from the history or examination should alert the examiner to the possibility of cervical spine injury and prompt an imaging evaluation using plain x-rays, CT, or MRI.
Acute brainstem ischemia due to basilar artery thrombosis may cause brief episodes of spontaneous extensor posturing superficially resembling generalized seizures. Coma of sudden onset, accompanied by these movements and cranial nerve abnormalities, necessitates emergency imaging. A noncontrast CT scan of the brain may reveal a hyperdense basilar artery indicating thrombus in the vessel, and subsequent CT or MR angiography can assess basilar artery patency.
Other diagnostic studies are best used in specific circumstances, usually when neuroimaging studies fail to reveal a structural lesion and the etiology of the altered mental state remains uncertain. Electroencephalography (EEG) can be important in the evaluation of critically ill patients with severe brain dysfunction. The EEG of metabolic encephalopathy typically reveals generalized slowing. One of the most important uses of EEG is to help exclude inapparent seizures, especially nonconvulsive status epilepticus. Untreated continuous or frequently recurrent seizures may cause neuronal injury, making the diagnosis and treatment of seizures crucial in this patient group. Lumbar puncture (LP) may be necessary to exclude infectious or inflammatory processes, and an elevated opening pressure may be an important clue to cerebral venous sinus thrombosis. In patients with coma or profound encephalopathy, it is preferable to perform a neuroimaging study prior to LP. If bacterial meningitis is suspected, an LP may be performed first or antibiotics may be empirically administered before the diagnostic studies are completed. Standard laboratory evaluation of critically ill patients should include assessment of serum electrolytes (especially sodium and calcium), glucose, renal and hepatic function, complete blood count, and coagulation. Serum or urine toxicology screens should be performed in patients with encephalopathy of unknown cause. EEG, LP, and other specific laboratory tests are most useful when the mechanism of the altered level of consciousness is uncertain; they are not routinely performed in clear-cut cases of stroke or traumatic brain injury.
Monitoring of ICP can be an important tool in selected patients. In general, patients who should be considered for ICP monitoring are those with primary neurologic disorders, such as stroke or traumatic brain injury, who are at significant risk for secondary brain injury due to elevated ICP and decreased CPP. Included are patients with the following: severe traumatic brain injury (Glasgow Coma Scale [GCS] score ≤8 [see Table 44-2]); large tissue shifts from supratentorial ischemic or hemorrhagic stroke; or hydrocephalus from subarachnoid hemorrhage (SAH), intraventricular hemorrhage, or posterior fossa stroke. An additional disorder in which ICP monitoring can add important information is fulminant hepatic failure, in which elevated ICP may be treated with barbiturates or, eventually, liver transplantation. In general, ventriculostomy is preferable to ICP monitoring devices that are placed in the brain parenchyma, because ventriculostomy allows CSF drainage as a method of treating elevated ICP. However, parenchymal ICP monitoring is most appropriate for patients with diffuse edema and small ventricles (which may make ventriculostomy placement more difficult) or any degree of coagulopathy (in which ventriculostomy carries a higher risk of hemorrhagic complications) (Fig. 33-3).
TREATMENT OF ELEVATED ICPElevated ICP may occur in a wide range of disorders, including head trauma, intracerebral hemorrhage, SAH with hydrocephalus, and fulminant hepatic failure. Because CSF and blood volume can be redistributed initially, by the time elevated ICP occurs, intracranial compliance is severely impaired. At this point, any small increase in the volume of CSF, intravascular blood, edema, or a mass lesion may result in a significant increase in ICP and a decrease in cerebral perfusion. This is a fundamental mechanism of secondary ischemic brain injury and constitutes an emergency that requires immediate attention. In general, ICP should be maintained at <20 mmHg and CPP should be maintained at ≥60 mmHg.
Interventions to lower ICP are ideally based on the underlying mechanism responsible for the elevated ICP (Table 33-2). For example, in hydrocephalus from SAH, the principal cause of elevated ICP is impairment of CSF drainage. In this setting, ventricular drainage of CSF is likely to be sufficient and most appropriate. In head trauma and stroke, cytotoxic edema may be most responsible, and the use of osmotic agents such as mannitol or hypertonic saline becomes an appropriate early step. As described above, elevated ICP may cause tissue ischemia, and, if cerebral autoregulation is intact, the resulting vasodilation can lead to a cycle of worsening ischemia. Paradoxically, administration of vasopressor agents to increase mean arterial pressure may actually lower ICP by improving perfusion, thereby allowing autoregulatory vasoconstriction as ischemia is relieved and ultimately decreasing intracranial blood volume.
Early signs of elevated ICP include drowsiness and a diminished level of consciousness. Neuroimaging studies may reveal evidence of edema and mass effect. Hypotonic IV fluids should be avoided, and elevation of the head of the bed is recommended. Patients must be carefully observed for risk of aspiration and compromise of the airway as the level of alertness declines. Coma and unilateral pupillary changes are late signs and require immediate intervention. Emergent treatment of elevated ICP is most quickly achieved by intubation and hyperventilation, which causes vasoconstriction and reduces cerebral blood volume. To avoid provoking or worsening cerebral ischemia, hyperventilation, if used at all, is best administered only for short periods of time until a more definitive treatment can be instituted. Furthermore, the effects of hyperventilation on ICP are short-lived, often lasting only for several hours because of the buffering capacity of the cerebral interstitium, and rebound elevations of ICP may accompany abrupt discontinuation of hyperventilation. As the level of consciousness declines to coma, the ability to follow the neurologic status of the patient by examination lessens and measurement of ICP assumes greater importance. If a ventriculostomy device is in place, direct drainage of CSF to reduce ICP is possible. Finally, high-dose barbiturates, decompressive hemicraniectomy, and hypothermia are sometimes used for refractory elevations of ICP, although these have significant side effects and have not been proven to improve outcome.
SECONDARY BRAIN INSULTSPatients with primary brain injuries, whether due to trauma or stroke, are at risk for ongoing secondary ischemic brain injury. Because secondary brain injury can be a major determinant of a poor outcome, strategies for minimizing secondary brain insults are an integral part of the critical care of all patients. Although elevated ICP may lead to secondary ischemia, most secondary brain injury is mediated through other clinical events that exacerbate the ischemic cascade already initiated by the primary brain injury. Episodes of secondary brain insults are usually not associated with apparent neurologic worsening. Rather, they lead to cumulative injury limiting eventual recovery, which manifests as a higher mortality rate or worsened long-term functional outcome. Thus, close monitoring of vital signs is important, as is early intervention to prevent secondary ischemia. Avoiding hypotension and hypoxia is critical, as significant hypotensive events (systolic blood pressure <90 mmHg) as short as 10 min in duration have been shown to adversely influence outcome after traumatic brain injury. Even in patients with stroke or head trauma who do not require ICP monitoring, close attention to adequate cerebral perfusion is warranted. Hypoxia (pulse oximetry saturation <90%), particularly in combination with hypotension, also leads to secondary brain injury. Likewise, fever and hyperglycemia both worsen experimental ischemia and have been associated with worsened clinical outcome after stroke and head trauma. Aggressive control of fever with a goal of normothermia is warranted but may be difficult to achieve with antipyretic medications and cooling blankets. The value of newer surface or intravascular temperature control devices for the management of refractory fever is under investigation. The use of IV insulin infusion is encouraged for control of hyperglycemia because this allows better regulation of serum glucose levels than SC insulin. A reasonable goal is to maintain the serum glucose level at <10.0 mmol/L (<180 mg/dL), although episodes of hypoglycemia appear equally detrimental and the optimal targets remain uncertain. New cerebral monitoring tools that allow continuous evaluation of brain tissue oxygen tension, CBF, and metabolism (via microdialysis) may further improve the management of secondary brain injury.
FIGURE 33-3
Intracranial pressure and brain tissue oxygen monitoring. A ventriculostomy allows for drainage of cerebrospinal fluid to treat elevated intracranial pressure (ICP). Fiberoptic ICP and brain tissue oxygen monitors are usually secured using a screwlike skull bolt. Cerebral blood flow and microdialysis probes (not shown) may be placed in a manner similar to the brain tissue oxygen probe.

Insert ICP monitor—ventriculostomy versus parenchymal device General goals: maintain ICP <20 mmHg and CPP ≥60 mmHg. For ICP >20–25 mmHg for >5 min:
|
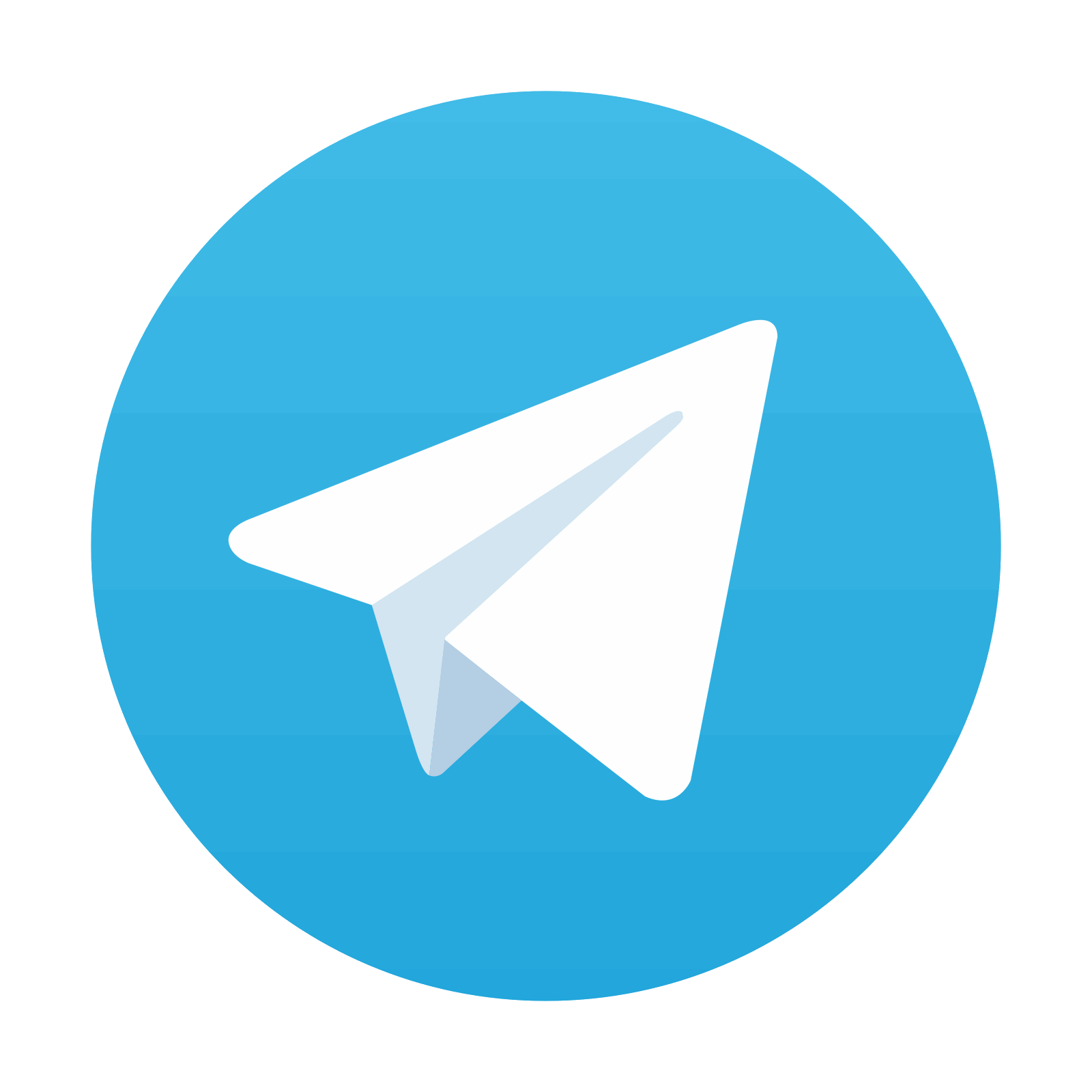
Stay updated, free articles. Join our Telegram channel

Full access? Get Clinical Tree
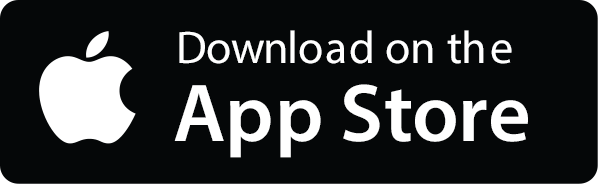
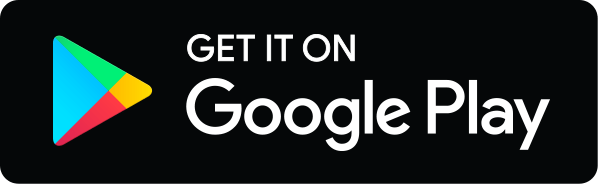
