Metastatic complications of breast cancer
Central nervous system
Cranial
Parenchymal brain metastases
Skull base metastases
Dural metastases
Spinal
Intramedullary spinal cord metastases
Spinal epidural metastases
Leptomeningeal metastases
Peripheral nervous system
Peripheral nervous system metastases
Neoplastic plexopathy
Non-metastatic, non-treatment-related complications
Paraneoplastic syndromes
Meningiomas
Treatment related complications
Central nervous system
Encephalopathy
Cognitive injury
Radionecrosis
Cardiovascular complications
Meningitic syndromes
Myelopathy
Peripheral nervous system
Plexopathy
Radiculopathy
Neuropathy
Peripheral neuropathy
Myopathy
Metastatic Complications
Central Nervous System Metastases
Parenchymal Brain Metastases (Fig. 23.1)
Breast cancer (BC) is one the most frequent systemic cancers resulting in brain metastases (BM). Between 10 and 30% of BC patients will develop BM [1]. BM often have a significant clinical impact by reducing the QoL and compromising survival [2].


Fig. 23.1
Brain metastases. Brain MRI, T1 W gadolinium-enhanced axial images
BM in BC most often are metachronous in presentation and seen in patients with known cancer (80%), but can also be the first manifestation of cancer (5–10%) [3] or discovered concomitantly with systemic disease. BM are solitary in 20 to 30% of patients and are oligometastatic (2 or 3 lesions) in the same proportion [2, 3]. Signs and symptoms of BM are related to the anatomic localization of the metastases. Approximately 50% of patients present with headache, and 30% present with focal neurological signs, mostly seizures [4]. Cranial computed tomography (CT) scan is able to detect BM; however, the sensitivity and specificity of magnetic resonance imaging (MRI) are considerably higher [5]. Contrast MRI permits a more precise evaluation of the number and location of BM. BM are located in the cerebral hemispheres (80% overall) and less frequently in the cerebellum (15%) or brainstem (5%). Once developed, the neurological symptoms/signs do not always resolve, even in patients responding to treatment. Early diagnosis and serial follow-up after BM are diagnosed as important to minimize emergence of CNS disease that may compromise QoL and survival. In the CEREBEL study of human epidermal growth factor receptor 2 (HER2)-positive metastatic BC, nearly 20% of the patients had asymptomatic BM. Considering the high proportion of BM in BC and the increasing treatment options in the HER2-positive population, serial brain MRI appears clinically appropriate [3].
The incidence of and the time to presentation of BM vary according to the subtype of BC (categorized as luminal A: ER/PR positive, HER2 negative; luminal B: triple positive; HER2: HER2 positive, ER/PR negative; and basal: triple negative). In a cohort of 383 BC patients treated for BM, both the median time between BC and BM diagnoses and the median overall survival (OS) following discovery of BM were significantly different among the different BC subtypes [6]. The shortest interval between BC diagnosis and BM diagnosis was observed in the basal/triple negative and the HER2 subtypes (Table 23.2) [7, 8]. The overexpression of HER2 is associated with a higher risk of BM; between 30 and 50% of HER2-positive patients will develop BM [9–12]. Several risk factors for BM have been identified in BC patients, including overexpression of HER2, estrogen receptor negativity, triple negative status, young age, nodal involvement, high-grade tumors, and larger tumor size [7, 8, 13–15].
Table 23.2
Incidence, median delay between breast cancer (BC) diagnosis and brain metastases (BM) diagnosis, and median overall survival after BM diagnosis
Incidence of the different BC subtypes in BM (%) | Median delay between BC diagnosis and BM diagnosis (months) | Median survival after BM diagnosis (months) | References | |
---|---|---|---|---|
Luminal A | 20 14 | 54.4 30 | 10.0 9.3 7.4 5 | Sperduto [6] Niikuara [16] Aversa [15] Bachmann [8] |
Luminal B | 25.5 35 | 47.4 96 33.5 | 22.9 16.5 19.2 16.5 | Sperduto [6] Niikuara [16] Aversa [15] Hayashi [17] |
HER2 | 31 49 | 35.8 36 33.5 | 17.9 11.5 7 11.5 | Sperduto [6] Niikuara [16] Aversa [15] Hayashi [17] |
Basal /triple negative | 23.5 22 | 27.5 35 | 7.2 7.3 4.9 4.9 5 | Dawood [399] Sperduto [6] Niikuara [16] Aversa [15] Bachmann [8] |
In a Japanese retrospective cohort of 1256 BC patients with BM, the median OS was 8.7 months [16]. Shortest OS was observed in the non-HER2-positive subtypes in the cohort of Sperduto et al. [7, 8, 15]. No significant difference in OS was observed among HER2-positive tumors in a large cohort of 423 HER2-positive BC patients with BM [17]. In this cohort, BM patients treated with trastuzumab and lapatinib had a significantly longer survival as compared to patients treated with trastuzumab alone, lapatinib alone, or no HER2-targeting agent. The subtype of BC appears to not only influence prognosis but cause death as well. Half of all patients with HER2-positive BM die of CNS disease progression, whereas patients with triple negative BC die most commonly of systemic disease [9, 18].
The Breast Graded Prognostic Assessment (Breast-GPA) instrument developed by the RTOG (Radiation Treatment Oncology Group) can assist in determining the prognosis of BC patients with BM and guide treatment. For BC, the GPA instrument used a single prognostic factor, the Karnofsky Performance Status (KPS) [19]. In another RTOG cohort of newly diagnosed BC patients, significant prognostic factors included the KPS, the HER2, ER/PR status, and the interaction between ER/PR and HER2 [6]. The initial Breast-GPA was revised based on a larger cohort of BC patients with BM and now includes, KPS, BC subtype, and age (Table 23.3). The median OS was estimated at 3.4 months for patients with a Breast-GPA score >1 and at 25.3 months for patients with a BC-GPA score between 3.5 and 4.0 [6]. Notably, the number of BM was not integrated in the revised Breast-GPA; however, a recent analysis of another large cohort of patients with BC and BM suggested that the number of BM (>3 vs. ≤3) has a significant impact on survival [20]. In the aforementioned Japanese cohort, multivariate analyses found increased survival in patients diagnosed with BM within 6 months of metastatic BC diagnoses, with asymptomatic BM, or with HER2-positive/estrogen receptor-positive tumors [16]. In another retrospective cohort of 215 BC patients, non-luminal subtype, presence of extracranial disease, time to CNS metastases <15 months, the presence of >3 BM, and a low Breast-GPA were associated with a shorter survival [21].
Table 23.3
Revised breast cancer graded prognostic assessment
Factor | 0.0 | 0.5 | 1.0 | 1.5 | 2.0 |
---|---|---|---|---|---|
KPS | ≤50 | 60 | 70–80 | 90–100 | – |
Genetic subtype | Basal | – | Luminal A | HER2 | Luminal B |
Age | ≥60 | <60 | – | – | – |
Group | Breast-GPA | MST (months) (95% CI) | 1 year OS (95% CI) | 2 year OS (95% CI) | 3 year OS (95% CI) |
---|---|---|---|---|---|
Group 1 | 0.0–1.0 | 3.4 (2.4–4.9) | 15 (4–33) | 0 | 0 |
Group 2 | 1.5–2.0 | 7.7 (4.8–9.7) | 32 (23–41) | 13 (6–20) | 6 (2–13) |
Group 3 | 2.5–3.0 | 15.1 (10.8–17.9) | 55 (46–63) | 29 (21–37) | 19 (11–27) |
Group 4 | 3.5–4.0 | 25.3 (20.4–30.4) | 77 (69–84) | 53 (43–61) | 31 (22–40) |
Relatively few trials have been prospectively studied in BC patients with BM. Indeed, in the majority of BM trials, lung cancer patients represent the largest population. As BC and lung cancer share a better radiosensitivity relative to melanoma [22], the management of BC BM can be in part extrapolated from the results of these trials. Initial treatment for newly diagnosed BC-related BM includes surgery, whole-brain radiotherapy (WBRT), stereotactic radiotherapy (stereotactic radiosurgery [SRS] and stereotactic radiotherapy [SRT]), systemic therapy, clinical trials, and palliative care. The choice of treatment depends on the number, localization, and volume of BM, the neurological and overall status of the patient, and the control of the systemic disease [23, 24]. The intent of BM-directed treatment in BC can be curative or palliative. Additionally, supportive care is important following BM diagnosis. The lowest effective dose of steroids is recommended for the management of vasogenic edema to avoid steroid-related toxicity that may further compromise QoL. According to the American Academy of Neurology and European guidelines, prophylactic antiseizure treatment is not recommended in patients with BM [25, 26]. In a patient with seizures and BM in whom an antiepileptic drug is indicated, the use of non enzyme-inducing agents is recommended to minimize interactions with systemic anticancer treatments.
Surgical resection is considered for patients with accessible and limited BM. In a cohort of 42 BC patients with BM, the median OS after surgery was 16 months [27]. In this case series, age was the only factor significantly correlated in multivariate analyses with OS. In another cohort of 116 BC patients with BM, the median OS was 11.5 months [28]. Surgical resection is performed in patients with a solitary BM in non-eloquent brain regions and in patients with well-controlled systemic disease. Surgical resections of all lesions in patients with up to 3 BM may improve survival as well [29, 30]. Indications are less clear in patients with multiple BM or uncontrolled extracranial disease, but in some cases, surgery can provide rapid relief of symptoms due to intracranial hypertension and obstructive hydrocephalus, particularly in patients with a large symptomatic lesion [31]. In summary, resective surgery is indicated for (1) therapeutic indications including a large symptomatic or asymptomatic lesion; (2) diagnostic indications including the absence of known primary tumor, doubt regarding the metastatic origin of the lesion, uncertainty between radionecrosis, and progression after brain irradiation; and (3) histological and molecular biological documentation. Advances in surgery include functional MRI (fMRI) with fiber tract mapping, transcranial stimulation, and intra-operative MRI. Piecemeal resection should be avoided as the recurrence rate is 1.7 times higher than tumors removed en bloc, particularly for lesions in the posterior fossa and in contact with CSF pathways [32, 33]. When feasible, a microscopic total resection with a 5-mm margin of normal-appearing tissue may lead to a reduced incidence of local recurrence [34]. When surgery is performed, histological and molecular biomarkers should be re-examined to verify the concordance between the primary BC and the BM and to identify potential druggable targets.
The indications for stereotactic radiosurgery (SRS) or stereotactic RT (SRT) include BM in patients with surgically inaccessible metastases, post-surgical treatment of the operative cavity, and progressive BM in patients previously treated with WBRT (re-irradiation). SRT is used in patients with a limited number of BM, limited lesional volume, and controlled systemic disease. SRS is often used for patients with less than 5 BM. However, large retrospective cohorts with up to 15 lesions have reported similar outcomes to those with 1–4 BM [35–38]. The total volume of tumor has been suggested to be more important than total number of lesions [39]. Local control rates observed after SRS range from 64 to 94% [31]. SRS alone may be as effective as surgery and SRS of the resection cavity [40–42]. In a cohort of 136 BC patients presenting with 1–3 BM and treated with SRS, local failure rate was 10% at 12 months and the median OS was 17.6 months. On multivariate analyses, patients with >1 lesion, triple negative BC, and poorly controlled extracranial disease had a shorter OS [43]. In another cohort of 131 BC patients with BM treated by SRS, the median OS varied significantly according to the type of BC, ranging from 7 months in triple negative patients, to 16 months in ER-positive HER2-negative patients, 23 months in ER-negative HER2-positive patients, and 26 months in ER-positive HER2-positive patients [44]. The dose of SRS varies according to the volume of the lesion and the potential risk of radionecrosis. In a small study, the dose of SRS in BC patients with 1–3 BM was evaluated by comparing 20 patients treated with 20 Gy versus 10 patients treated with 16–18.5 Gy [45]. The local control rates were 94% after 20 Gy and 48% after 16–18.5 Gy.
WBRT has long been the standard treatment for BM, though its role has changed with the advent of SRS/SRT, new methods of WBRT, such as hippocampal avoidance, and improved knowledge of outcome based upon BC subtype. Notably, however, WBRT decreases local and distant failures after either surgery or SRS/SRT [46]. Addition of WBRT results in a clinically significant benefit in 64–83% of patients with BM and is associated with an increase of 2–6 months in overall survival [47]. BC, as well as non-small cell lung cancer (NSCLC), are more likely to respond to WBRT compared to other histologies [48]. Response rates to WBRT in BC patients with BM vary from 65 to 82% [48–50]. The combination of WBRT and systemic agents constitutes another potential treatment for BC BM. The addition of temozolomide to WBRT in BC patients did not improve local control or survival in a phase II randomized trial (NCT 00875355) [51]. The addition of veliparib, an oral PARP inhibitor, to WBRT was well tolerated and showed encouraging preliminary efficacy data in a cohort with 31% of BC patients [52].
A phase III European Organization for Research and Treatment of Cancer trial (EORTC 22952-26001) assessed the impact of WBRT after surgery or SRS on the preservation of functional independence in patients with 1–3 BM (>90% with a solitary BM) from solid tumors (excluding small cell lung cancer) with stable extracranial disease and a 0–2 WHO performance status (PS) [53]. In this study, 199 patients underwent SRS and 160 underwent surgery. The median time to a deterioration of WHO PS by more than 2 points was 10.0 months after observation and 9.5 months after WBRT. OS was not significantly different between the 2 arms (10.9 months after WBRT and 10.7 after observation). The 2-year relapse rate at both the initial treated site and at new sites of disease was significantly reduced after WBRT, although BC represented only 12% of all tumors in this trial. The role of WBRT added to SRS in BC patients with 1–3 BM has been reported in a retrospective cohort of 30 patients treated by SRS alone and 28 treated by SRS plus WBRT [54]. The addition of WBRT to SRS resulted in significantly longer survival without new BM but no difference was observed in survival between the 2 groups. In the EORTC trial, a significantly better health-related quality of life (HRQOL) was observed in the observation arm compared to the WBRT arm during the first year after treatment [55]. The authors conclude that WBRT was detrimental to several elements of the HRQOL. This study also reported that frequent monitoring for BM recurrence did not have a negative impact on HRQOL. The impact of WBRT on cognition was assessed in a small randomized controlled study (NCT 00548756). In this trial, patients with 1–3 newly diagnosed BM were randomly assigned to SRS plus WBRT or SRS alone. The primary endpoint was cognition measured by Hopkins Verbal Learning Test-Revised (HVLT-R) total recall at 4 months. The study was stopped in accordance with trial stoppage guideline, after determination of a 96% probability that patients in the SRS + WBRT group were significantly more likely to have cognitive decline as compared to patients treated by SRS alone [56]. In the Alliance trial (NCT00377156), 213 patients with 1–3 BM, each <3 cm were randomized between SRS alone or SRS plus WBRT [57]. The primary objective was to evaluate cognitive decline. After SRS alone, intracranial tumor control with SRS was 66.1% at 6 months and 50.5% at 12 months. After SRS plus WBRT, intracranial tumor control was 88.3% at 6 months and 84.9% at 12 months. Median OS was 10.7 months after SRS alone and 7.5 months after SRS plus WBRT. Moreover, despite better local control after WBRT without improvement of OS, the cognitive decline was higher after SRS plus WBRT.
The incidence of radiation-induced white matter injury has been assessed in a retrospective cohort of 35 BC patients treated by SRS plus WBRT and 30 patients treated by SRS alone. A higher incidence of white matter hyperintensities by brain MRI was observed after combined treatment. At one year, 71.5% of the patients treated with both SRS and WBRT demonstrated white matter lesions (limited periventricular hyperintensity in 42.9%, diffuse white matter hyperintensity in 28.6%), whereas only one patient treated with SRS developed white matter lesions [58].
The use of WBRT is increasingly deferred, given evidence that WBRT negatively impacts QoL and cognition, and because BC patients, especially those with HER2 positive tumors, are living longer. Initial treatment with SRS and close radiographic monitoring is thus preferred in patients with a limited number of BM. The indications for WBRT include multiple and disseminated BM, and failure of stereotactic radiotherapy [31]. The incidence of cognitive decline as assessed by a decline in the Mini-Mental Status Examination (MMSE) was determined in a prospective randomized trial comparing WBRT plus SRS versus SRS alone in 110 patients with 1–4 BM [59]. Deterioration in the MMSE was seen in 39% of patients in the WBRT plus SRS group and 26% of patients in the SRS only group. On average deterioration occurred at 13.6 months in the WBRT plus SRS group and at 6.8 months in the SRS group. Recognizing the limitations of the MMSE as an instrument to assess cognition, these data suggested that cognitive impairment in patients with treated BM may result from radiation-related toxicity as well as recurrence of BM. Thus the control of brain disease is of particular importance for preservation of cognition.
Several approaches have been evaluated to minimize RT-associated cognitive decline. Memantine, a N-methyl-d-aspartate (NMDA) receptor agonist, was assessed in a randomized double-blind placebo controlled trial in patients treated with WBRT [60]. Memantine was well tolerated but the difference in delayed recall, the primary endpoint, was not significant between treatment arms. Nonetheless significantly longer time to cognitive deterioration, reduction in the rate of decline in delayed recognition, executive function and processing speed was observed with memantine. Perihippocampal stem cells injury as a consequence of WBRT may contribute to RT associated cognitive decline, and thus shielding the hippocampal neural stem cell compartment was evaluated in a phase II study utilizing hippocampal avoidance WBRT (HA-WBRT) in patients with BM (RTOG 0933) [61]. In this study, the median OS was 6.8 months. The mean relative decline in Hopkins Verbal Learning Test-Revised Delayed Recall (HLVT-R DR) at 4 months (primary endpoint) was 7.0%, significantly lower than historical controls.
The efficacy of liposomal doxorubicin in combination with cyclophosphamide was evaluated in a retrospective study of 29 BC patients. The objective response rate in brain was 41.4% after 3 cycles (50% in the absence of prior brain RT). The median OS from brain diagnosis was 23 months [62]. BM responses have also been reported with anthracycline-based regimens, CMF (cyclophosphamide, methotrexate and 5-fluorouracil), and high-dose methotrexate [23]. Temozolomide as single agent in metastatic BC and BM has little activity based upon two small phase II studies in which the objective response rate was <6% and transient [63, 64]. Similarly gefitinib has no activity, based on a single phase II trial [65]. Interim analyses of the phase II did not meet the efficacy point [23]. TPI-287, a microtubule-stabilizing agent, designed to bypass the MDR-1 drug efflux resistance mechanism is undergoing study [23]. Other new agents under development include cabazitaxel (NCT01913067), ANG1005 (NCT01480583), TPI-287 (NCT01332630) and 2B3-101 a glutathione-pegylated doxorubicin (NCT01386580).
Targeted therapies are highly efficacious in BC. Genomic and epigenomic profiling of metastases may provide a basis for future therapeutic strategies [66, 67]. Endocrine therapies represent the oldest targeted therapies in BC, and responses of BM to tamoxifen, aromatase inhibitors and megestrol acetate have been reported in small case reports [3]. However, loss of hormonal receptor positivity is frequent in BM (when compared to the matched primary tumor) often obviating the use of hormonal agents [68]. In a cohort of 36 paired samples of the primary BC and BM, the discordance rates were 28% for ER and 20% for PR [69]. Additionally the majority ER-positive BC is endocrine-refractory when BM is diagnosed [23].
Anti-HER2 targeted agents play an important role in the management of BM in HER2 positive breast cancer. In the cohort of 36 paired tumors mentioned above, the discordant rate was only 3% only for HER2 status [69]. Improved OS has been reported in several retrospective studies in HER2 positive BC patients with BM when treated with trastuzumab [12, 70, 71]. Approximately one third of metastatic BC patients receiving trastuzumab develop BM [12, 72]. In 50%, BM are diagnosed when systemic disease is controlled. BM has been shown to develop later in patients treated with trastuzumab [70]. Furthermore, a survival benefit has been reported with continuation of trastuzumab-based therapies after development of BM [70, 73–77]. However, the impact on OS may relate more to control of extracranial disease.
The efficacy and the safety of lapatinib alone was first evaluated in 39 patients with HER2 positive progressive BM all previously treated by trastuzumab and by WBRT in 37 cases [78]. The objective response rate was low (2.6%). The median time to progression was 3.0 months suggesting very limited activity as a single agent. In a phase II study, 242 HER2 positive BC patients with progressive BM previously treated with trastuzumab and cranial RT were treated with lapatinib alone [79]. The objective response rate was 6%; 21% had a volumetric response of 20% or more. Of the 50 patients enrolled in the extension phase of the study that added capecitabine to lapatinib, a CNS objective response was observed in 20% (according to RECIST criteria) and 40% (based on volumetric response of 20% or more). Importantly, the effect of capecitabine alone cannot be excluded in the extension phase, although lapatinib may have had an additive effect. The modest activity of lapatinib in the CNS replicates that seen in systemic disease as well [80]. The efficacy of the combination of lapatinib and capecitabine was confirmed in a cohort of 22 evaluable HER2 positive BC patients with previously treated BM [81]. Partial responses were observed in 7 patients and stabilization was observed in 6 patients. The median brain PFS was 5.6 months and the median OS was 27.9 months, significantly longer than in patients treated with trastuzumab-based therapies after CNS progression (16.7 months). The response rates observed with lapatinib and capecitabine were replicated in several expanded access programs [18% response reported by Boccardo and 21% reported by Sutherland] [82, 83]. In combination with capecitabine, CNS response rates vary from 18 to 38% [78, 79, 81–84].
Excess toxicity and lack of efficacy was observed in a study comparing the combination of lapatinib plus topotecan versus lapatinib plus capecitabine [84]. The efficacy of the combination of lapatinib and capecitabine was studied in the LANDSCAPE phase II trial (NCT00967031) in untreated asymptomatic BM with HER2 positive BC [85]. A high partial response rate of 66% was seen. The median time to progression was 5.5 months. This approach constitutes an option for patients with HER2 positive tumors that are low volume and paucisymptomatic. The CEREBEL trial, designed to demonstrate that the combination of lapatinib plus capecitabine could reduce the incidence of BM compared to trastuzumab plus capecitabine, was closed early due to a low incidence of BM (3% in the lapatinib plus capecitabine group and 4% in the trastuzumab plus capecitabine group) [86].
In a retrospective study, Bartsch evaluated the impact of trastuzumab and lapatinib on 80 HER2 positive BC patients with BM [71]. Median OS was 13 months in patients treated with trastuzumab after diagnosis of BM, 9 months in patients treated with chemotherapy and 3 months in patients treated with RT only. Addition of lapatinib to trastuzumab, either sequentially or concomitantly, prolonged OS compared to trastuzumab alone. Thus, the combination of lapatinib and trastuzumab may be an option for BC BM.
The combination of trastuzumab and WBRT resulted in a response rate of 74% suggesting possible radiosensitization [87]. The potential radiosensitizing impact of lapatinib in combination with WBRT was evaluated in a phase I trial in which a response rate of 79% was observed [88]. Further studies are ongoing. New anti-HER2 therapies (pertuzumab, TDM-1) have been approved recently; however, their impact on BM is not yet well defined. Regimens containing afatinib, an irreversible HER2 and EGFR inhibitor, failed to improve PFS in a phase III trial, compared to either trastuzumab or lapatinib [89]. Only a few responses were observed with neratinib in a phase II study enrolling 40 BC patients with progressive BM previously treated (NCT01494662) [90]. The association of neratinib and capecitabine is also under investigation [91]. Other new anti-HER2s, including ARRY-380, a HER2-selective tyrosine kinase inhibitor, are in development [24, 91].
A CNS response of 63% was observed on prespecified volumetric criteria in a phase II trial evaluating the combination of carboplatin and bevacizumab in BC BM patients [92]. By RECIST criteria, the response rate was 45%. In another phase II trial, the objective CNS response rate according to RECIST criteria was 77% for the combination of bevacizumab, etoposide and cisplatin [93]. The median CNS PFS and OS were 7.3 and 10.5 months, respectively.
mTOR, PI3 K and dual mTOR/PI3 K inhibitors are currently being explored in breast cancer. Everolimus, a rapamycin analogue that inhibits mTOR signalling, is being evaluated in a phase II trial in combination with trastuzumab and vinorelbine in HER2 positive BC with BM. BKM20, an oral pan-PI3 K inhibitor, is also being studied in HER2 positive BC patients with BM (NCT01132664). PARP inhibitors, which disrupt DNA repair, such as ABT-888, are also being investigated in BM patients (NCT00649207). Iniparib, initially developed as a PARP inhibitor but subsequently shown not to have any PARP inhibitor activity, showed a modest activity in BM [24]. Immune-based approaches including ipilimumab and anti-PD-1 therapies may also be new promising approaches if activity is confirmed in BC.
Skull Base Metastases
Skull base metastases occur in approximately 4% of cancer patients [94], and are found in 22% of autopsy cases [95]. BC is one of the most common causes of skull base metastases, accounting for 20.5–40% of all cases [94–96]. Skull base metastases are most often diagnosed in patients with disseminated disease, particularly to other skeletal elements [96]. Direct hematogenous spread is the primary route of dissemination to the skull base. Rarely, retrograde seeding through the valveless venous plexus of Batson has been proposed [97, 98]. Progressive ipsilateral involvement of cranial nerves is the main presentation of skull base metastases. The clinical manifestations depend on the size, location and growth rate of the metastases. The etiology of signs and symptoms is multifactorial and includes stretching of the dura, compression of cranial nerves, irritation of adjacent brain and occlusion of dural venous sinuses [99]. Five main clinical syndromes have been defined based on anatomic site of disease (Table 23.4) [96].
Table 23.4
Main clinical syndromes associated with skull base metastases
Clinical syndrome | Clinical description |
---|---|
Sellar and parasellar syndrome (29%) | Sellar Characterized by co-occurring pituitary metastases Most often silent as near complete destruction of the adenohypophysis is necessary to produce clinical manifestations Posterior metastases: diabetes insipidus Anterior metastases: hypopituitarism and visual loss Lateral extension: cranial neuropathies affecting oculomotor and trigeminal nerves Parasellar Oculomotor and trigeminal nerve palsies Frontal headache, facial paresthesia or pain, and periorbital swelling are also reported |
Occipital condyle syndrome (16%) | Unilateral severe and constant pain in the occipital region followed by ipsilateral 12th cranial nerve palsy leading to dysarthria and dysphagia, atrophy of the ipsilateral tongue with associated fasciculations Meningismus is frequent Radiation of pain to the forehead Isolated hypoglossal nerve palsy |
Orbital syndrome (12.5–15%) | Progressive frontal headaches particularly over the ipsilateral affected eye with associated blurred vision and diplopia Sensory loss of the forehead due to trigeminal sensory involvement Proptosis and ophthalmoplegia of the involved eye Local signs such as periorbital swelling |
Middle fossa syndrome, or Gasserian ganglion syndrome (6%) | Facial paresthesias, numbness, and pain of the face sparing the forehead Headache uncommon Sensory loss of the second and third divisions of the trigeminal nerve and more rarely the first division Motor deficits of the trigeminal nerve or of the abducens nerve common |
Jugular foramen syndrome (3.5%) | Hoarseness, dysphagia, and unilateral dull pain in the occipital and pharyngeal areas Paralysis with involvement of cranial nerves 9th–11th observed Glossopharyngeal neuralgia-associated syndrome with syncope or papilledema |
Numb chin syndrome | Numbness of the chin usually unilateral |
Hemibasis syndrome | Progressive ipsilateral paralysis of at least seven cranial nerves |
Contrast MRI is the technique of choice for the diagnosis of skull base metastases. Fat suppression techniques are particularly useful. Bone metastases are characterized by a hypointense lesion on non-enhanced T1-weighted MRI sequence [100]. Contrast enhancement is variable on T1 weighted sequences with fat suppression. MRI further permits determination of invasion of the cavernous sinus, the dura or cranial nerves. Orbital CT may help by demonstrating bone anatomy and associated erosion. Importantly, a normal imaging study does not exclude the diagnosis [94]. Radionuclide bone scan can reveal skull base metastases, but sensitivity is poor particularly for osteolytic lesions [101]. FDG-PET has a similar accuracy to that of bone scintigraphy [102]. Rarely, biopsies are needed to establish the diagnosis. The role of a cerebrospinal fluid (CSF) analysis is to exclude co-associated leptomeningeal metastases (LM).
Skull base metastases are most often a late event in the course of cancer [94].The overall prognosis depends of the type of cancer, remaining therapeutic options, and the site and extent of the skull base metastases. In the cohort of Laigle Donadey, BC had the longest survival, with a median survival of 60 months [94]. Cranial nerve palsies are associated with a poorer OS [94].
Asymptomatic skull base metastases can be followed [100] and symptomatic patients treated with supportive therapy including steroids, analgesics, and bisphosphonates, as needed. Radiotherapy, alone or in combination with chemotherapy or surgery, is the most frequently used therapy (in >70%) [94]. Excellent pain relief and regression of cranial nerve dysfunction may occur and improved neurological function is seen if treatment commences early [93, 103]. SRS may be an alternative therapy, in particular for previously irradiated areas and for small metastases [94, 100, 104]. Lastly, surgery may be used in selected patients [94, 105]. Chemotherapy and endocrine therapies may be an option for patients with chemoresponsive disease [94].
Dural Metastases
Dural metastases are localized to dural and surrounding space (epidural and subdural) [106] and are most frequently associated with breast, prostate, lung, head and neck, and hematologic malignancies. Overall, BC is the primary cancer most often associated with dural metastases, occurring in 16.5–34% of cases [106–108]. Intracranial dural metastases occur in up to 10% of BC patients in autopsy series [106, 107]. Four mechanisms of dural seeding have been proposed: direct extension from skull metastases, hematogenous dissemination, seeding through Batson’s plexus, and spread from the lymphatic circulation [107]. Those derived from direct extension of skull metastases account for 60% and dural metastases from hematogenous spread for 36% [106, 108]. In most patients with BC and dural metastases, recurrent/progressive systemic disease (>80%) is found [106].
In a retrospective cohort of 122 patients with intracranial dural metastases, the most frequent symptoms include headache (due to increased intracranial pressure) and cranial nerve palsy (due skull base location), followed by visual disorders, alterations in mental status, and seizures [106]. Signs and symptoms are related to the location and the extent of the dural metastases. Between 11 and 20% of patients are asymptomatic and diagnosed incidentally by brain imaging [106, 107]. Non-traumatic subdural hematoma, also called “pachymeningitis interna hemorrhagica,” can be observed in 15–40% of cases and generally are asymptomatic [106–108]. Cerebral venous thromboses have been described secondary to infiltration of the cerebral venous sinuses [106]. In one study, 56% of the patients had a single dural metastasis and 25% demonstrated diffuse dural enhancement. The most common sites of dural metastases were parietal (36%) or frontal (32%). Infratentorial lesions were observed in 11%. Dural metastases can appear as a localized thickening of the dura or as nodules, usually biconvex or lenticular in shape [106, 108]. A combination of diffuse dural enhancement and dural nodules can be observed as well. Homogeneous intense post-contrast enhancement is most often seen. MRI findings include a dural tail (44%), vasogenic edema (53%), and brain invasion (34%), most commonly by direct extension from skull [106]. Skull metastases are observed in 70% of cases [106]. Other findings may include venous sinuses involvement and subdural hematoma or effusions. Meningioma is the main differential diagnosis [106, 108]. Infections or inflammatory lesions may be considered as well in appropriate context.
Treatment is not standardized. Surgery should be considered as the first option in patients with a resectable solitary lesion, controlled extracerebral disease, and acceptable surgical risk [106–108]. In the cohort of Nayak, 25% of the patients underwent surgical resection, consisting of complete resection in 63% of the cases. Focal radiotherapy, SRS/SRT, and WBRT are also options, alone or in combination with surgery. In the cohort of Nayak, 52% of patients received RT of whom 47% received WBRT and 53% focal RT or SRS. Systemic treatment may be an option in some cases as these lesions are outside the blood–brain barrier [106]. Best supportive care, including corticosteroids, may be indicated in some patients.
In a literature review, the median OS was 6 months varying whether surgery was performed, to control extracranial disease and the primary tumor type [108]. In this review, dural metastases from BC were associated with a more favorable outcome compared to other tumor types, with a median OS of 9 months. In the cohort of Nayak, the median PFS was 3.7 months and the median OS was 9.5 months. The initial site of progression was intracranial in 30% of the patients and both intracranial and systemic in 21%. The intracranial progression was local in the dura in 86% of patients, distant in the dura in 37%, intraparenchymal in 41%, and leptomeningeal in 6% [106]. In this study, surgical resection was shown to improve PFS and OS. Chemotherapy prolonged PFS, but not OS. An impaired PS was associated with a worse outcome.
Intramedullary Spinal Cord Metastases
BC is the second most common solid tumor responsible for intramedullary spinal cord metastasis (ISCM), after lung cancer. In a meta-analysis of 96 autopsy-proven cases with ISCM, lung cancer (49%) and BC (14%) were the most common primary cancers [109]. Treatment options include best supportive care, surgery, RT, and systemic therapy. Treatment depends on the number, volume, and location of the metastases, the extent of systemic disease, the general and neurological status of the patient, and life expectancy [110]. In all cases, the treatment is urgent so as to forestall paraplegia. Symptomatic treatment with corticosteroids can be a useful adjunct. After supportive care only, clinical deterioration is observed in 89% of the cases [111].
In highly selected patients with solitary metastases, with a reasonable life expectancy, and without leptomeningeal, brain, or widespread metastases, the prognosis may be improved by surgery [111–114]. A gross total resection can sometimes be achieved in these otherwise well-circumscribed metastases [114]. After surgery, improvement in clinical symptoms can be observed in 58% of the cases, no change in 31%, and clinical deterioration in 11%. Adjuvant RT following surgery should be administered [112].
As BC is radiosensitive, RT may be effective and is the most commonly used treatment for the majority of patients. After RT, transient stabilization of neurological disease progression and a reduction in pain is often achieved [109, 111]. The schedule of RT should be shortened so as to account for the poor OS [115]. Stereotactic radiotherapy is also an option. After SRT treatment, clinical improvement is noted in 21% of the cases, no change in 63%, and clinical deterioration in 11% [110]. BC is chemosensitive as well, and systemic agents may be effective depending on the neurological status, the volume of the metastases, available systemic treatment options, and the extent of the systemic disease. Nevertheless, no clear impact on survival has been shown [110].
For a more complete review of ISCM please refer to Chap. 6 of this text.
Spinal Epidural Metastases (Fig. 23.2)
Metastatic epidural spinal cord compression (ESCC) is seen in 5–10% of all patients with BC [114, 116–120], usually in the context of known metastatic disease [114] and often (25%) with concurrent CNS metastases [121]. Median survival of patients with ESCC may be longer for patients with breast cancer in comparison to other tumor types. While the median overall survival for all patients with ESCC has been reported to range between 4 and 14 months [121], survival in BC patients with ESCC was the longest (21.5 months) in a study of 81 patients that included 23.6% BC patients [119].


Fig. 23.2
Epidural spinal cord compression. Spinal MRI, T1 W gadolinium-enhanced sagittal image
For a complete review of spinal epidural metastases and ESCC, including presenting symptoms, treatment, and prognostic factors, please refer to Chap. 6.
Leptomeningeal Metastases (Fig. 23.3 a, b)
Leptomeningeal metastasis (LM) or neoplastic/carcinomatous meningitis is diagnosed in 1–8% of patients with solid tumors during the course of the cancer; however, it is diagnosed at autopsy in 19% of patients with premorbid neurological signs and symptoms [122–126]. BC, in addition to lung cancer and melanoma, is one of the most common primary cancers responsible for LM. Risk factors of LM in BC include an infiltrating lobular carcinoma and cancers negative for estrogen and progesterone receptors [18, 127–130]. Triple negative status in BC has been reported as being a risk factor of LM [129, 131, 132]. In contrast, LM is observed in only 3–5% of HER2-positive BC; thus, HER2-positive status is not considered a risk factor for LM [18]. Other risk factors have been identified and include piecemeal resection instead of en bloc resection [33], surgical resection of parenchymal cerebellar metastases [133–135], surgical resection of supraventricular BM that violates the ventricular system [136–138], deferring WBRT after BM resection [139], and improved survival due to more effective systemic therapy often associated with poor CNS penetration [124].


Fig. 23.3
a, b Leptomeningeal metastases. a Brain MRI, T1 W gadolinium-enhanced axial images. b Spinal MRI, T1 W gadolinium-enhanced sagittal image
The median OS of untreated patients with LM is limited to 4–6 weeks [123–125]. Despite aggressive treatment, survival is limited to a few months. Compared to patients with LM disease from other solid tumors, BC patients have a better prognosis [123–125, 140, 141]. The median OS in BC patients is estimated at 3.3–5 months [123, 124, 130, 140–153]. One-year survival varies from 7–24% [154]. Prognostic factors enumerated by the NCCN CNS guidelines include a poor risk group defined by a KPS below 60, multiple neurologic deficits, extensive systemic disease with few treatment options, bulky CNS disease, and the presence of a LM-related encephalopathy [155]. On multivariate analyses, an association has been observed between OS and the PS, age at LM diagnosis and treatment (number of prior chemotherapy regimens, receipt of combined treatment modality, coadministration of systemic chemotherapy, and intra-CSF chemotherapy) [140, 141, 156]. Other prognostic factors in BC patients include histological characteristics (histological grade and hormone receptor status), number of prior chemotherapy regimens, status of systemic disease, initial response to treatment, cytologic response to treatment, and in one study, the concentration of cyfra 21-1 (a fragment of cytokeratin 19 thought to represent a tumor marker) in the CSF [130, 143–146].
Treatment of LM should ideally be initiated as early as possible before the appearance of disabling neurologic deficits [124]. In the majority of patients, progressive extracranial disease coexists and must be taken into account in the management of LM. Only one randomized trial, prematurely closed for low accrual (n = 35), was performed in LM BC patients comparing systemic therapy and RT with or without intra-CSF methotrexate [157]. No significant difference between groups was observed in clinical response (neurologic improvement: 41 vs. 39%; disease stabilization: 18 vs. 28%) or median survival (18.3 vs. 30.3 week). However, treatment-related complications were higher in patients treated with intra-CSF chemotherapy compared to the no intra-CSF chemotherapy arm (47 vs. 6%). Further studies are needed to define the role of intra-CSF therapy in the treatment of BC LM. Combined treatment (both systemic chemotherapy and intra-CSF chemotherapy) is preferred when possible in patients considered for LM treatment due to an improvement in survival.
Chemotherapy allows simultaneous treatment of the entire neuraxis and can be administered systematically or intra-CSF. The choice of agent is based on the chemosensitivity profile of the primary tumor and the ability of drug to penetrate into the CSF compartment [124, 125]. Paclitaxel and docetaxel are effective in metastatic BC, but have poor penetration into the CNS [158].
Capecitabine in case reports has produced responses and disease stabilization in BC LM [159–163]. As in BC BM, temozolomide for the treatment of LM was disappointing [164]. The efficacy of new agents such as eribulin has not been yet demonstrated. High-dose methotrexate or cytarabine is not often administered due to significant systemic toxicity and the requirement for hospitalization. Efficacy of endocrine therapies has been demonstrated in case reports, but acquired resistance is often present at this stage of the disease. Bevacizumab, a monoclonal antibody targeting the vascular endothelial growth factor (VEGF) ligand, has shown prolonged responses in case reports with LM [165–168]. Prospective trials are ongoing to confirm the role of bevacizumab in LM (NCT NCT00924820). A response to trastuzumab emtansine has been reported [169]. The efficacy of other anti-HER2 therapies such as lapatinib and pertuzumab has not been demonstrated. A glutathione-pegylated liposomal formulation of doxorubicin and anthracycline is under investigation in BC LM (NCT01818713).
Intra-CSF treatment, in combination with systemic therapy, is often used for the treatment of LM notwithstanding its superiority relative to systemic therapy only has never been established in a randomized trial [123, 124]. The goal of intra-CSF treatment is to bypass the blood–CSF barrier which is only partially disrupted in LM and increase drug exposure in the CSF compartment while mitigating systemic toxicity [124, 125]. Three main intra-CSF chemotherapy agents are used: cytarabine (free or liposomal), methotrexate (standard and high-dose regimens), and thiotepa with differing doses and schedules (Table 23.5). The optimal dose and schedule, particularly concerning maintenance therapy, are not well defined [125, 154, 170]. Many studies have reported no differences in efficacy among these agents, including in patients with BC-related LM [130, 143–150]. Liposomal cytarabine, which resulted in a significant longer survival as compared to methotrexate in one randomized trial, requires less frequent clinic visits and may have less impact on QoL [171]. Combination (multi-agent) intra-CSF chemotherapy has not demonstrated improved results and is not recommended for the treatment of BC-related LM. Intra-CSF etoposide has shown modest efficacy [172]. Prolonged responses have been reported after intra-CSF trastuzumab in case studies [173–176]. Two studies are currently ongoing to define the safety and efficacy profile of intra-CSF trastuzumab in HER2-positive BC patients (NCT01325207 and NCT01373710).
Table 23.5
Intra-CSF chemotherapy dose and schedule
Drug | Description of the drug | CSF half-life | Description of the regimen |
---|---|---|---|
Liposomal cytarabine | Pyrimidine nucleoside analogue, cell cycle specific | 14–21 days | 50 mg every 2 weeks (total, 8 wks) then 50 mg once every 4 weeks |
Methotrexate | Folate antimetabolite, cell cycle-specific drug | 4, 5–8 h | Standard regimen 10–15 mg twice weekly (total, 4 wks), then 10–15 mg once weekly (total, 4 wks) then 10–15 mg once monthly Low-dose regimen 2 mg/d (d1–d5) every other week High-dose regimen 15 mg/d (d1–d5) every other wk |
Thiotepa | Alkylating ethyleneimine compound, cell cycle non-specific drug | 3–4 min | 10 mg twice weekly (total, 4 wks) then 10 mg once weekly (total, 4 wks) then 10 mg once a month |
For a complete review of LM, please refer to Chap. 5.
Peripheral Nervous System Metastases
Neoplastic Plexopathy
Neoplastic plexopathy typically occurs at the time of local or regional progression of cancer. BC is the second (32%) most common cause of neoplastic brachial plexopathy after lung cancer, and the third (11%) leading cause of lumbosacral neoplastic plexopathy after lung cancer and soft tissue sarcoma [177, 178]. Neoplastic plexopathy manifests as severe pain, followed by motor weakness and sensory disturbances in the distribution of plexus involvement [177, 178]. When the brachial plexus is involved, the lower trunk is most frequently affected. Pain is observed in 75–83% of the patients and is usually located in the shoulder and axilla [177, 179]. Radicular pain is common and radiates into the arm with extension into the fourth and fifth digits. Motor weakness and loss of reflexes are seen in 75% of cases, mostly in the lower plexus distribution. More widespread signs of whole plexus involvement are occasionally noted. A Horner’s syndrome occurs in 23% of the patients with a neoplastic brachial plexopathy [177]. In lymphedema-associated plexopathy, patients present with neuropathic extremity pain (90%), followed by weakness (86%), sensory loss (73%), reflex loss (64%), and limb edema (47%) [177]. Tumor spread to the plexus most commonly occurs by direct invasion of the plexus or hematogenous spread from distant metastases. Adjacent structures, such as infiltrated lymph nodes or soft tissues, can also result in direct compression of the plexus [177, 179, 180].
Contrast MRI is more sensitive than CT in the identification of neoplastic plexopathy [177, 179]. Increased T2/FLAIR MRI intensity in nerve trunks with or without contrast enhancement is commonly observed. If no lesion is observed, a repeat MRI examination within a 4- to 6-week interval commonly reveals tumor not previously appreciated on initial examination [177]. The presence of tumor recurrence, especially in the area of plexus, in combination with a supportive examination, is presumptive for a diagnosis of metastatic plexopathy. The sensitivity and specificity of PET are not clear, but PET can be helpful in instances of negative MRI. Electromyography demonstrates a unilateral lesion often with more extensive denervation than clinically predicted [177]. Lower medial trunk/cord lesions or whole plexus lesions are more frequent than upper trunk lateral cord lesions [179].
The main differential diagnosis is radiation-induced plexopathy (Table 23.6). The pattern of distribution of weakness and the results of nerve conduction studies may help to distinguish between neoplastic and radiation-induced brachial plexopathy [181]. A more complete discussion on this topic is featured later in this chapter. Other differential diagnosis include LM, ESCC, paraneoplastic plexopathy, complications of regional intra-arterial chemotherapy, post-infectious plexopathy, and primary plexus tumors [177]. Radiotherapy of the involved area is the most commonly used treatment and often results in durable responses. Chemotherapy and endocrine therapy may be used with some utility in chemosensitive cancer [180]. Pain should be treated according to the WHO guidelines. Physical therapy may help to augment and preserve neurologic function.
Table 23.6
Differential diagnosis: Neoplastic brachial plexopathy and radiation-induced brachial plexopathy
Presentation | Neoplastic plexopathy | Radiation associated plexopathy |
---|---|---|
Clinical presentation | Severe and early pain, occasional edema, involvement of the lower brachial plexus, Horner’s syndrome common, No tissue necrosis | Paresthesia and weakness, pain late during the course of the disease, edema common, impairment of the whole plexus, Horner’s syndrome unusual, local tissue necrosis common |
Electromyography | Myokymia unusual | Myokymia usually present |
MRI | Nerve contrast enhancement | Usually no nerve contrast enhancement |
PET scan | High SUV max | Low SUV max |
Non-metastatic, Non-treatment-related Complications
Paraneoplastic Syndromes
Paraneoplastic neurological syndromes (PNS) affect less than 1% of patients with BC [182]. BC is most often associated with the following PNS: cerebellar degeneration, sensory neuropathy, encephalomyelitis, opsoclonus myoclonus, stiff person syndrome, myelopathy, and brainstem encephalitis (Table 23.7) [183, 184]. Subacute cerebellar degeneration is the most common PNS seen with BC [185]. Other paraneoplastic syndromes, such as retinopathy, have been reported in BC (Table 23.8). Associated systemic symptoms including weight loss, anorexia, fever, and fatigue may be observed [186].
Table 23.7
Autoantibodies and common paraneoplastic syndromes (PNS) associated with breast cancer
Autoantibodies | Common paraneoplastic syndrome |
---|---|
Amphiphysin | Stiff person syndrome, myelopathy and myoclonus, encephalomyelitis, sensory neuronopathy |
Ri (ANNA2) | Brainstem encephalitis, opsoclonus myoclonus |
Yo (PCA1) | Paraneoplastic cerebellar degeneration |
AMPAR | Limbic encephalitis |
CAR | Retinopathy |
Table 23.8
Most frequent paraneoplastic syndromes (PNS) in breast cancer
Name of the PNS | Description of the paraneoplastic syndrome |
---|---|
Subacute cerebellar degeneration | Development in <12 weeks of a severe pancerebellar syndrome without cerebellar atrophy on MRI other than age expected Initial gait ataxia followed by appendicular ataxia Initial symptoms may also include dizziness, visual problems (diplopia, blurry vision, oscillopsia), nausea/vomiting, or dysarthria These rapidly progressive symptoms stabilize after culminating in a severe pancerebellar syndrome Other neurological symptoms and signs that may be seen include cognitive impairment, lethargy, bulbar palsy, or limb weakness Other PNS (paraneoplastic peripheral neuropathy or Lambert–Eaton myasthenic syndrome) sometimes associated Cerebellar atrophy on MRI in the late stages of the disease often associated with anti-Yo antibodies |
Sensory neuronopathy | Subacute and asymmetric numbness, paresthesia, pain, cramps, proprioceptive loss of upper and lower extremities and abolition of deep-tendon reflexes Radicular symptoms and signs sometimes associated Involvement of the sensory fibers on electroneurography |
Encephalomyelitis | Involvement of multiple parts of the CNS such as the hippocampus, brainstem, spinal cord, or dorsal root ganglia Manifests as limbic encephalopathy, brainstem encephalitis, dysautonomia, myelitis, and sensory neuronopathy |
Opsoclonus myoclonus syndrome | Spontaneous large amplitude saccades occurring in all directions of gaze Myoclonus of the trunk and limbs Encephalopathy sometimes associated Often associated with Ri antibodies |
Stiff person syndrome | Slowly progressive stiffness and rigidity of axial musculature Followed by proximal muscle rigidity Leads to difficulties in walking and fixed deformities. Associated with superimposed muscle spasms that are increased by sensory stimuli Variant form with an onset of stiffness in upper limbs Antibodies against amphiphysin Continuous motor activity at rest on electroneurography |
Subacute progressive myelopathy | Progressive symptoms and signs of myelopathy Symmetric MRI T2 abnormalities and gadolinium enhancement that involves the spinal gray matter in usually >3 segments Antibodies against amphiphysin |
Brainstem encephalitis | Rapidly progressive symptoms and signs of brainstem dysfunction. Antibodies against Ri (ANNA2) |
The best characterized onconeural antibodies associated with BC and PNS are amphiphysin associated with the stiff person syndrome, myelopathy, myoclonus, encephalomyelitis, and sensory neuronopathy; Ri (ANNA2) associated with brainstem encephalitis and opsoclonus myoclonus; and Yo (PCA1) associated with paraneoplastic cerebellar degeneration [184]; AMPAR (amino-3-hydroxy-5-methyl-4-isoxazolepropionic acid receptor) associated with limbic encephalitis; and anti-Ma2 (anti-Ta) associated with limbic encephalitis, brainstem encephalitis, and cerebellar degeneration [184, 187, 188].
PNS precedes the diagnosis of cancer in more than 80% of the cases by several months to years [183, 189]. In most cases, an underlying cancer is identified within 5 months after the diagnosis of PNS. The risk of a coexistent cancer decreases after 2 years and is considered as highly unlikely after 4 years [183]. When BC is considered in a patient with a PNS, a cancer screen should include a clinical examination and mammography followed by breast MRI and body FDG-PET in cases with negative initial screening [184, 188]. When the initial screen is negative, repeat assessments should be performed every 6 months for at least 2 years [188].
For a complete review of PNS, please refer to Chap. 13.
Meningiomas (Fig. 23.4)
Meningioma is one of the most common primary tumors of the CNS in adults and accounts for 13–26% of all intracranial tumors [190–192]. The only well-established risk factors are prior ionizing brain RT and some rare hereditary diseases such as neurofibromatosis [190–195]. Mutation in the NF2 gene and genetic variants in genes involved in DNA repair pathway are often identified in meningioma [196]. Several observations suggest a link between a patient’s hormonal status and meningioma. In intracranial meningioma, the female: male ratio is 2–3.5, which is even higher in spinal meningioma (4:1 ratio) [194, 197, 198]. Progesterone receptors are detected in 70–98% of meningiomas; estrogen and androgen receptors are seen in 20–40% of meningiomas [193, 195, 199, 200]. Increased meningioma growth rate is reported during pregnancy [193, 194]. An association between BC and meningioma has been demonstrated [193, 195, 201]. In addition, a link between use of exogenous hormones and meningioma has been reported [194], as well as a positive association with endometriosis or uterine fibrosis [196]. A non-significant association has been shown between age at menarche, age at menopause, menopausal status, parity, age at first birth, and meningioma [193, 194, 202–204]. A protective role of breastfeeding has also been observed [204–207]. The evidence that use of oral contraceptives and hormone replacement therapy increase the risk of meningioma remains controversial; however, in most studies [194, 204], no association was found [193–195, 203–206, 208–213].


Fig. 23.4
Meningioma. Brain MRI, T1 W gadolinium-enhanced sagittal image
An association between BC and meningioma has been postulated. In a large Swedish cohort, an increased risk of meningioma was observed after BC with a relative risk of meningioma estimated at 1.6 [214]. In another US population-based retrospective cohort analysis, the risk of BC in patients previously diagnosed with a meningioma was 1.54, and the risk of meningioma in BC patients was 1.40 [211]. In a five-state US cancer registry, the incidence of meningioma was 2.6 cases per 100,000 women and the incidence of BC was 61 cases per 100,000 women. The association between the two cancers was higher than expected [201]. The pathophysiology of this association is not well understood. BRCA1 and BRCA2 mutations do not seem to contribute to the development of meningioma [215]. In a large US SEER registry, associations between BC and meningioma were reported [216]. BC preceded meningioma by >2 months in 48% of the women, and tumors were synchronous (within 2 months) in 20%. Hormone receptor status of BC was not different between BC only and BC plus meningioma groups. BC disease stage was more advanced in the BC plus meningioma group than in the BC only group. Contrary to this study, in a large retrospective study of BC patients, the 10-year cumulative incidence of meningioma was only 0.37%, suggesting no increased risk [217]. Use of aromatase inhibitors confers a non-significant increased risk of meningioma in patients with BC [217], whereas tamoxifen use may be protective. The risk of meningioma has been shown to be lower in patients treated with tamoxifen compared to those not treated with this agent [192].
Complications of Breast Cancer Treatment
Central Nervous System Complications
Encephalopathy
Any anticancer drug given at conventional dose can be responsible for causing a toxic encephalopathy [218]. Toxic chemotherapy-related encephalopathy may manifest as a confusional state, posterior reversible leukoencephalopathy, seizures, a cerebellar syndrome, myelopathy, or cerebrovascular complications [219, 220]. The signs and symptoms can vary from mild and transient to severe and chronic [219, 221]. 5-FU (5-fluorouracil) and capecitabine represent the most frequent chemotherapy agents responsible for CNS toxicity in BC. The symptoms can originate within days to months after the initial administration of the drug. Most toxic encephalopathies are transient, last only days, and are associated with complete neurologic recovery [222–225]. MRI changes seen with toxic encephalopathies resolve more slowly than clinical manifestations. The pathophysiology of toxic encephalopathy is not clearly understood. Direct endothelial cell injury has been proposed with associated vasogenic edema [222, 226]. Most occurrences of encephalopathy are believed to be idiosyncratic and with a low risk of recurrence [219, 220, 227].
Several antineoplastic agents used in BC may induce an encephalopathy (Table 23.9). Methotrexate results in encephalopathy in less than 2% of patients [219, 228]. Administration of methotrexate and brain RT further increases the risk of CNS neurotoxicity [221]. Acute encephalopathies have been described after the administration of paclitaxel or very rarely docetaxel, vinorelbine, and pegylated liposomal doxorubicin in BC patients, notwithstanding limited penetration of the CNS with these agents [229–233]. Anti-VEGF agents such as bevacizumab are rarely the cause of posterior reversible encephalopathy or PRES [234, 235]. Leukoencephalopathy and encephalopathy were observed in 2.5 and 5% of patients with LM following lumbar injection and 7.5 and 5% after ventricular injection of liposomal cytarabine in a large cohort of patients treated for LM [236]. In a smaller cohort of patients treated with intraventricular methotrexate, leukoencephalopathy was observed in 64% of patients [237]. Leukoencephalopathy can be delayed, developing 3–15 months after intra-CSF methotrexate [237]. Patients may manifest with personality changes, cognitive deficits, and apathy followed by hemiparesia or quadriparesia and coma. Lesions predominate in the periventricular white matter, and histological examination demonstrates demyelination, axonal degeneration, astrocytosis, and necrosis [238]. An elevation in MBP (myelin basic protein) can be observed in the CSF. Risk factors include high cumulative doses of intra-CSF methotrexate and concomitant systemic methotrexate plus WBRT [237]. An accidental overdose of intra-CSF methotrexate can lead to death. An acute transient mild encephalopathy with fever may be observed after intra-CSF methotrexate and which likely represents expansion of a treatment-related chemical meningitis discussed below [237].
Table 23.9
Breast cancer chemotherapy associated with toxic encephalopathy
Agent | Clinical description | Brain MRI |
---|---|---|
5-FU (5 fluorouracil) and capecitabine | Cerebellar syndrome or multifocal leukoencephalopathy with altered mental status ranging from confusion to coma, memory deficits, ataxia, dysarthria, nystagmus, headache, seizures, trismus, slurred speech, diplopia, and paresthesias | Normal or showing diffuse white matter alterations, with T2 and FLAIR periventricular hyperintensities of both hemispheres |
Methotrexate | Acute encephalopathy to severe delayed chronic encephalopathies with cognitive deficits, aphasia, and hemiparesis | Diffuse T2/FLAIR abnormalities |
Bevacizumab | Headache, visual disturbances, seizures, confusion, and relative hypertension | Parieto-occipital white matter abnormalities on T2/FLAIR sequences Less frequently involvement of anterior brain regions |
Other potential causes of encephalopathy must be excluded including CNS metastases (e.g., BM or LM), other toxic agents (e.g., antiepileptics, opioids, benzodiazepines), metabolic encephalopathies (such as hyper or hypoglycemia, azotemia, hepatic failure, electrolyte disorders), stroke, and infectious etiologies [222]. Cessation of the offending drug is indicated until resolution of symptoms. Neurological manifestations usually clear within a few days after discontinuing the chemotherapy [218, 222–224]. Nonetheless, in some patients, persistent neurological deficits are reported after discontinuation of the offending agent [223]. Steroids are of questionable benefit [223–225]. Dihydropyrimidine dehydrogenase deficiency, a genetic risk factor for MTX neurotoxicity, should be excluded [224]. In the absence of alternative chemotherapy, careful re-introduction of the drug at reduced dose and under close supervision is usually safe and associated with a low risk of recurrence [224].
Cognitive Impact of Endocrine Therapy and Chemotherapy
Cognitive impairment associated with chemotherapy is referred to as chemobrain or chemofog [239]. There are no established criteria that define the syndrome, related in part to methodological inconsistencies observed between studies discussed below. In BC, post-chemotherapy cognitive deficits have been observed in 17–75% of patients, as compared with 4–11% in healthy controls in retrospective cross-sectional studies [240–251]. Most longitudinal studies have confirmed a negative cognitive effect of chemotherapy in 15–25% of patients [244, 252–254]. In several prospective cohorts of BC patients, objective cognitive impairments have been observed in 20–35% at baseline before any systemic therapy without clear explanation as to cause [239, 241, 244, 255, 256]. Cognitive impairment due to chemotherapy emerges months to years after completion of treatment [257]. In prospective cohorts, cognitive decline was observed within the first 6 months after initiation of chemotherapy [246]. The duration of cognitive impairment is poorly defined. In longitudinal studies, cognitive impact appears to resolve over time, and stable or improved cognition has been observed 12–18 months following completion of chemotherapy [245, 252, 258]. Improvements in cognition may be delayed, and there exist a small subset of patients with refractory and intractable cognitive complaints after completion of chemotherapy [258–260].
Cognitive changes observed during chemobrain are often mild to moderate in severity and differ clinically from encephalopathy observed after chemotherapy [261]. The most common cognitive deficits include difficulties in attention and concentration, working memory, and executive and psychomotor functions [251, 258]. Deficits in verbal learning, nonverbal and visuospatial memory, psychomotor processing speed, mental flexibility, memory retrieval, confrontational naming, complex visuoconstruction, and fine motor dexterity have been reported as well [246, 250, 258, 262]. Patients report an inability to concentrate, organize their daily activities, or multitask. They also endorse memory loss, confusion, slow thinking, and fatigue [258]. The impact of these cognitive deficits in day-to-day activities varies and may be related to the patient’s pre-illness level of function, type of work or lifestyle, ability to manage, coping style, premorbid cognitive reserve, age, and other comorbidities such as mood or personality disorders, fatigue, and psychosocial issues [234, 239, 258, 261, 263–265]. Different studies have demonstrated that cognitive complaints are better correlated with emotional distress, coping skills, and cancer-related fatigue than with objective neuropsychological testing [239, 243, 261, 262, 266, 267].
The role of chemotherapy dose is not clear. One study demonstrated a greater deficit among women treated with high-dose chemotherapy compared to women treated with standard doses of chemotherapy [268]. In another longitudinal study, progressive cognitive decline was observed at each subsequent time point. This suggests a dose–response relationship with linear worsening following each cycle of chemotherapy [269]. Patients treated with combined chemotherapy and endocrine therapy have the most extensive pattern of cognitive deficits [245, 252, 260, 262]. Pretreatment cognitive impairment has been shown to be independent of fatigue or emotional distress, surgical factors, or medical comorbidities [261]. The exact mechanisms underlying the effects of chemotherapy on cognition are not clearly understood [244, 261]. Chemotherapy may have a direct neurotoxic effect and may induce oxidative stress, DNA damage, telomere shortening, damage to neural stem cells, hormonal changes, immune dysregulation, activation of pro-inflammatory cytokines, thrombosis in brain microvasculature, white matter abnormalities, metabolic abnormalities including anemia, and reduced brain functional connectivity [241, 261, 262, 270]. The role of genetic factors such as apolipoprotein E (APOE) and catechol-O-methyltransferase (COMT) has also been suggested [241, 244, 262, 271].
An evaluation of treatment-related cognitive disorders requires a neuropsychological assessment. In order to improve between-study comparisons, the International Cognition and Cancer Task Force (ICCTF) has developed recommendations for neuropsychological testing [242]. The Hopkins Verbal Learning Test-Revised (HVLT-R), Trail Making Test (TMT), and the Controlled Oral Word Association (COWA) of the Multilingual Aphasia Examination measuring learning and memory, processing speed, and executive function are recommended [242]. Baseline evaluation and an appropriate control group are suggested as is an evaluation of emotional functioning [261].
Volumetric brain MRI and diffusion tensor MRI (DTI) have shown widespread reduction in white matter tract integrity and gray matter volume over time in patients treated with chemotherapy and with cognitive deficits [251, 261]. A correlation has been observed between reduction in brain activation by fMRI and cognitive performance after chemotherapy in a longitudinal study [272]. Studies using fMRI have demonstrated alteration in brain activation and connectivity in BC patients after chemotherapy treatment [251, 258, 261]. Decreased activation by fMRI appears to relate to impaired cognition, whereas increased activation, typically seen in the context of absent or mild cognitive deficits, is thought to represent compensatory neural activation required to be maintained. PET shows similar activation patterns as seen with fMRI [241, 258, 261, 273]. Electrophysiological studies have shown a reduction in the amplitude of the P-300 event-related brain potential and a reduction in the P-300 latency, corresponding to the timing and duration of chemotherapy. This pattern was consistent with changes in information processing capacity [241, 258].
Chemobrain is a diagnosis of exclusion wherein PNS, CNS metastases, medication toxicity (corticosteroids, antiepileptics), fatigue, sleep disorders, pain, and preexistent neurological disease need to be excluded [261, 263]. The effectiveness of donepezil, an acetylcholinesterase inhibitor, for the treatment of chemotherapy cognitive deficits is uncertain [261]. No significant positive impact of psychostimulants such as methylphenidate, dexmethylphenidate, and modafinil, has been shown for the treatment of cognitive impairment in non-CNS cancer patients [239, 261]. Gingko biloba administered concomitantly with chemotherapy had no effect on cognitive function [274]. New approaches are needed, and promising results in animal studies suggest agents that prevent oxidative stress (2-mercaptoethane sulfonate, N-acetylcysteine, or melatonin), stimulate neurogenesis (insulin-like growth factor-1, fluoxetine, or glucose), or improve chemotherapy-induced cognitive effects (dextromethorphan or memantine) may be useful [261]. Learning compensatory strategies can help to minimize the impact of chemotherapy-related cognitive deficits [265]. Cognitive rehabilitation aims to improve cognitive abilities, functional capacity, and real-world skills [261]. Several studies show a significant improvement in both subjective and objective cognitive deficits with these interventions [244, 261]. Sleep disturbances, psychological distress, and fatigue, if present, should be addressed as treating these elements secondarily improves cognition [261]. Other medications that may affect cognition should be reduced or discontinued when possible. Physical activity has been associated with improved cognition, mostly executive function, in human and animal studies [275]. Neurofeedback and transcranial magnetic stimulation have been suggested as possibly useful interventions [261, 276].
Several studies have suggested a negative impact on cognition in BC patients treated with endocrine therapies, affecting mostly verbal memory and executive functions [277–286]. Deficits in processing speed and verbal memory were observed in postmenopausal patients compared to controls [279–281, 287]. A negative impact of tamoxifen or anastrozole on speed measures of letter fluency, complex visuomotor attention, and manual dexterity has also been reported [288]. In a separate trial, postmenopausal women with early-stage BC were treated with anastrozole or tamoxifen and the cognitive impact of each treatment was compared. A more severe impact on verbal and visual learning as well as on memory tests was observed after anastrozole [288]. Notably, no cognitive decline was reported at 6 and 24 months in the randomized IBIS II trial conducted in high-risk postmenopausal women without BC receiving anastrozole or placebo [282]. In the TEAM (tamoxifen and exemestane adjuvant multinational) study, no cognitive impact was found after exemestane treatment, but poorer performances on verbal memory and executive function were observed after tamoxifen compared to healthy controls [277]. In a phase III randomized trial, aromatase inhibitors did not appear to negatively affect verbal episodic memory during a 1-year follow-up [289]. The discordant findings in the above-mentioned studies could be explained by methodological inconsistencies such as differences in the characteristics of population studied (prior chemotherapy or not, inclusion of pre- or postmenopausal women), differences in the neurocognitive testing batteries, trial design (prospective vs. cross-sectional), timing and the duration of follow-up, and whether the evaluation of cognitive function was a primary study objective [277–281, 286, 287, 289]. Importantly, the impact of mood or fatigue on cognition is often not reported [268, 279, 290–294].
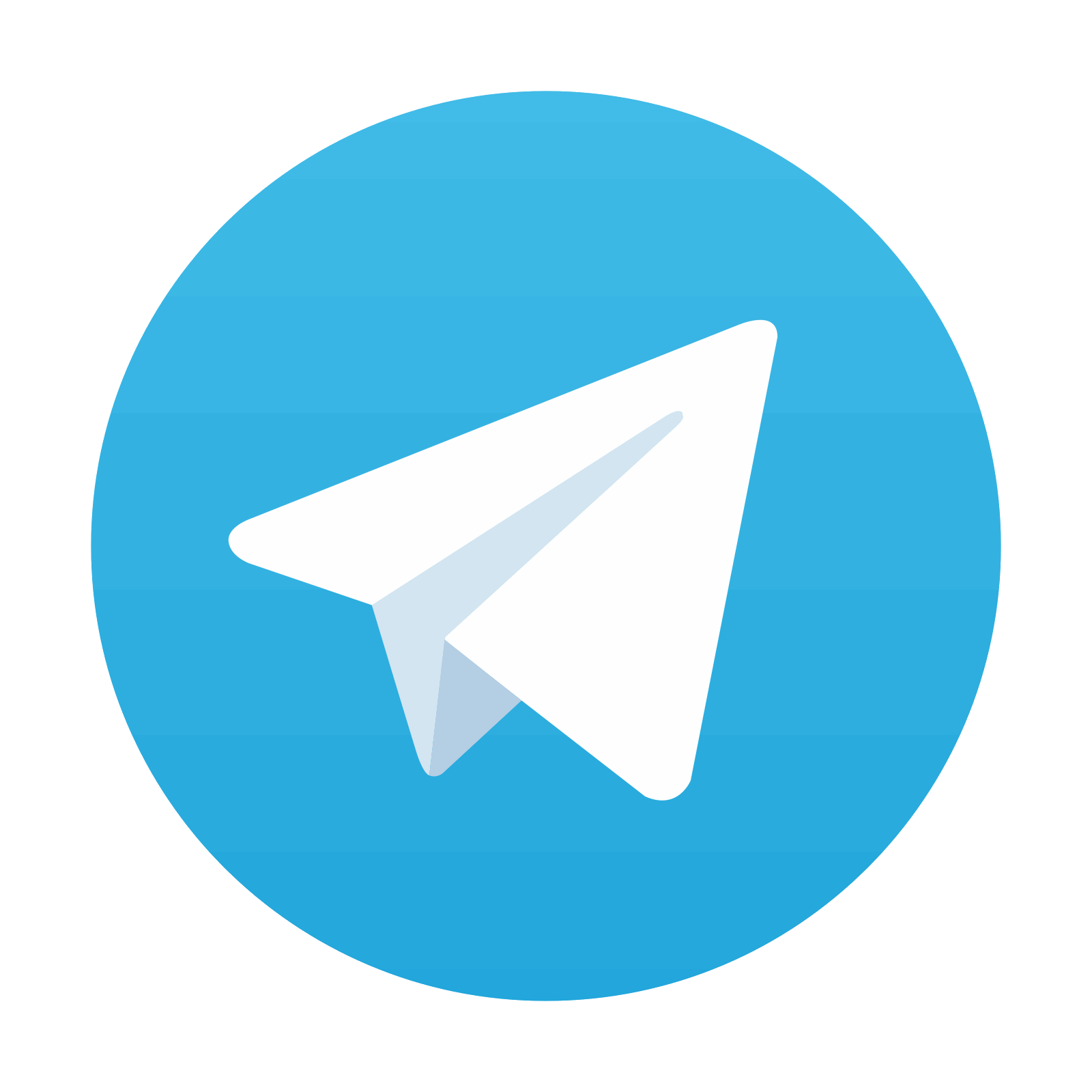
Stay updated, free articles. Join our Telegram channel

Full access? Get Clinical Tree
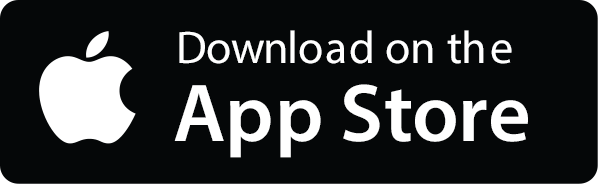
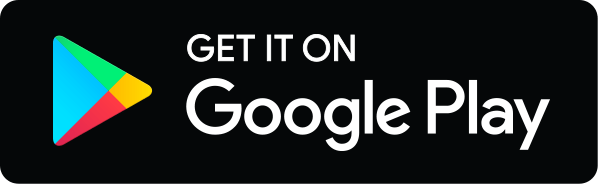