Fig. 17.1
Ipilimumab-related hypophysitis. MRI of the brain in a 77-year-old woman with metastatic melanoma. After the fourth cycle of ipilimumab, she developed profound weakness, fatigue, and confusion, with significant endocrine and electrolyte abnormalities relating to pituitary hypophysitis attributed to ipilimumab treatment. On T1-weighted, contrast-enhanced imaging, her MRI a shows an enhancing pituitary and stalk. The lesion abuts the optic chiasm. b shows the region prior to ipilimumab treatment, where no enhancement or enlargement is noted (Image courtesy of Sashank Prasad, M.D., Brigham and Women’s Hospital)
Pembrolizumab and Nivolumab
PD1 monoclonal antibodies, pembrolizumab (Keytruda®, Merck, Kenilworth, NJ, USA), and nivolumab (Opdivo®, Bristol-Myers Squibb, New York, NY, USA) are now approved for metastatic melanoma, with superior response rates and lower adverse event rates compared to ipilimumab [73, 74]. As a consequence, PD1 inhibitors may supplant CTLA-4 inhibitors as monotherapy for first-line treatment for metastatic melanoma. Indeed, responses rates (30–35% vs. 12%), progression-free survival, and overall survival favored pembrolizumab over ipilimumab, when compared head-to-head in a randomized phase III trial, using two different schedules of pembrolizumab compared to ipilimumab (given at standard dose and schedule of 3 mg/kg every 3 weeks for 4 doses) (Keynote-006) [74]. The toxicity profile of pembrolizumab was also favorable compared to ipilimumab with nearly half the number of grade 3–5 adverse events (10–13% vs. 19.2%). In a phase 3 study comparing nivolumab with dacarbazine in previously untreated melanoma, for instance, rare neurologic complications included headache (4.4%), paresthesias (1%), vertigo (1%), and hypophysitis (0.5%) [74]. Case reports highlight rare irNAEs including vasculitis [100], and encephalitis [101].
Notwithstanding the somewhat more favorable irAE profile, response rates to monotherapy with either PD-1 or CTLA-4 inhibitors remain <50%, prompting evaluation of dual immune checkpoint blockade and several combined approaches with targeted therapies, radiation, and chemotherapy. Two recent studies evaluating concurrent immunotherapy with CTLA-4 and PD1 inhibition reported improved response rates compared to monotherapy with either ipilimumab or nivolumab [73, 102]. While overall survival data are not yet available, the rate of severe (grade 3 or 4) adverse events was >50% leading to a 30–40% discontinuation rate in the combination setting, although irNAEs were not addressed. Future studies will address the issue of whether sequential immune checkpoint inhibition is comparable to concurrent therapy, but these studies underscore the importance of evaluation of adverse event monitoring with single-agent immunotherapies, combinations of immune checkpoint inhibitors, and the emerging combinations of immune checkpoint inhibitors with targeted agents or traditional chemotherapy. The adverse event rate in patients treated with nivolumab following prior treatment with ipilimumab was 18–33%, which is lower than seen with combined therapy but higher than reported rates of irAEs with nivolumab in the first line [103, 104]. There is a paucity of data regarding the side effect profile of patients receiving ipilimumab following progression on nivolumab or pembrolizumab, although there are some recent reports of early and severe toxicities following sequential immune checkpoint inhibition [105].
Immune Therapy Under Development
Chimeric Antigen Receptor (CAR) T Cells
An innovative approach to potent and specific cancer immunotherapy is the generation of engineered chimeric antigen receptor (CAR) autologous T cells [106]. Based on recent impressive results, these agents are likely to be FDA approved in the near future for acute B lineage leukemias. CARs are cytotoxic T cells that have the MHC-restricted T cell receptor replaced with an engineered chimeric receptor targeting the desired tumor cell-specific surface antigen. CARs improve on bispecific engagers by delivering tumor-specific cytotoxic T cells, activated through engineered chimeric receptors, which are able to bypass certain aspects of tumor-elaborated immune inhibition. Bispecific engagers, in contrast, rely on the recruitment of patient’s endogenous polyclonal T cells, and can be suppressed by tumor-elaborated factors, leading to immune evasion of tumors. The first CAR T cell in clinical trials targets CD19 (CD19 CAR) and has been tested in patients with relapsed ALL and refractory diffuse large B cell lymphoma (DLBCL) [1, 107]. Five adult patients with relapsed B cell acute lymphoblastic leukemia (B-ALL) were treated with CD19 CAR transfer. Amazingly, all patients had a complete response; there were no comments on neurological toxicity [108]. Among 15 patients with advanced B cell malignancies including DLBCL receiving with CD19 CAR in a phase 1/2a clinical trial, the majority showed complete remission with a 92% objective response rate [106]. Interestingly, in this study (much like in studies with bispecific engagers), neurological toxicity was prominent with common confusion and obtundation. Three of 15 patients also developed aphasia with various associated neurological symptoms ranging from myoclonus, to confusion, hemifacial spasm, facial hemiparesis, apraxia, and gait disturbance [106]. All patients improved between 11 and 20 days after administration, and one patient had lymphocytosis in the CSF that consisted 97% of T cells (32.9% CD19 CAR), despite lack of CD19 expression in the CNS (confirmed with serial brain biopsies and reported by multiple investigators) [106].
Summary
Advances in our understanding of cancer immunology are leading to rapid FDA approval of novel cancer treatments, harnessing the power of the immune system to fight cancer. While most of these immune therapies are well tolerated due to the exquisite self-regulation of the immune system, immune-related adverse events, including neurological side effects, can occur. Of the agents discussed here, vaccines cause the least neurologic complications, since they are both the most specific and allow for immune self-regulation. With global activation of the immune system, such as using IL-2 or checkpoint inhibition, rare autoimmune neurological complications can be seen such as myasthenia gravis, neuropathies, plexopathies, cranial nerve involvement, to name but a few. While irNAEs are rare with checkpoint inhibitors, they can cause neuroendocrine complications in the form of autoimmune hypophysitis, and rare but dangerous conditions such as Guillain–Barre syndrome (GBS). Cytokines such as IL-2 and especially IFN cross the blood–brain barrier and have CNS effects including neuropsychiatric changes ranging from depression to confusion. Some immunotherapies such as the anti-angiogenic and immune-active bevacizumab (Avastin) have neurotoxicities directly related to their mechanism of action, such that bevacizumab presents with increased risk of bleeding (intracranial hemorrhage), clotting (stroke), as well as complications related to microvascular dysfunction (PRES). CD19 targeting methods such as BiTEs or CD19 CAR T cells are the most neurologically active and can cause confusion, ataxia, encephalitis, aphasia, and neuropsychiatric complications; some neurotoxicities can be severe enough to force medication discontinuation. Many in the field are looking toward combination therapies either combining multiple immunotherapies, or combining immunotherapy with targeted, biological, or cytotoxic treatments with the hope of increased responses and durability. These trials have just begun, and it will be important to see how this changes the landscape of neurotoxicity in cancer treatment.
References
1.
Grupp SA, et al. Chimeric antigen receptor-modified T cells for acute lymphoid leukemia. N Engl J Med. 2013;368(16):1509–18.CrossrefPubMedPubMedCentral
2.
Hodi FS, et al. Improved survival with ipilimumab in patients with metastatic melanoma. N Engl J Med. 2010;363(8):711–23.CrossrefPubMedPubMedCentral
3.
4.
5.
Administration, U.S.F.A.D. Provenge [Internet]. 2010. Available from: http://www.fda.gov/downloads/BiologicsBloodVaccines/CellularGeneTherapyProducts/ApprovedProducts/UCM210031.pdf.
6.
Administration, U.S.F.A.D. Imlygic [Internet]. 2015. Available from: http://www.fda.gov/BiologicsBloodVaccines/CellularGeneTherapyProducts/ApprovedProducts/ucm469411.htm.
7.
8.
Agency EM. Talimogene laherparepvec [Internet]. 2015. Available from: http://www.ema.europa.eu/docs/en_GB/document_library/EPAR_-_Product_Information/human/002771/WC500201079.pdf.
9.
10.
Administration, U.S.F.A.D. TheraCys [Internet]. 1990. Available from:http://www.fda.gov/downloads/BiologicsBloodVaccines/CellularGeneTherapyProducts/ApprovedProducts/UCM310364.pdf.
11.
12.
13.
14.
Brausi M, et al. Side effects of bacillus Calmette-Guerin (BCG) in the treatment of intermediate- and high-risk Ta, T1 papillary carcinoma of the bladder: results of the EORTC genito-urinary cancers group randomised phase 3 study comparing one-third dose with full dose and 1 year with 3 years of maintenance BCG. Eur Urol. 2014;65(1):69–76.CrossrefPubMed
15.
Administration, U.S.F.A.D. Gardasil [Internet]. 2006. Available fhttp://www.fda.gov/downloads/BiologicsBloodVaccines/Vaccines/ApprovedProducts/UCM111263.pdf.
16.
17.
18.
19.
20.
21.
Patel MA, et al. The future of glioblastoma therapy: synergism of standard of care and immunotherapy. Cancers (Basel). 2014;6(4):1953–85.Crossref
22.
Liao W, Lin JX, Leonard WJ. Interleukin-2 at the crossroads of effector responses, tolerance, and immunotherapy. Immunity. 2013;38(1):13–25.CrossrefPubMedPubMedCentral
23.
24.
25.
Atkins MB, et al. High-dose recombinant interleukin 2 therapy for patients with metastatic melanoma: analysis of 270 patients treated between 1985 and 1993. J Clin Oncol. 1999;17(7):2105–16.CrossrefPubMed
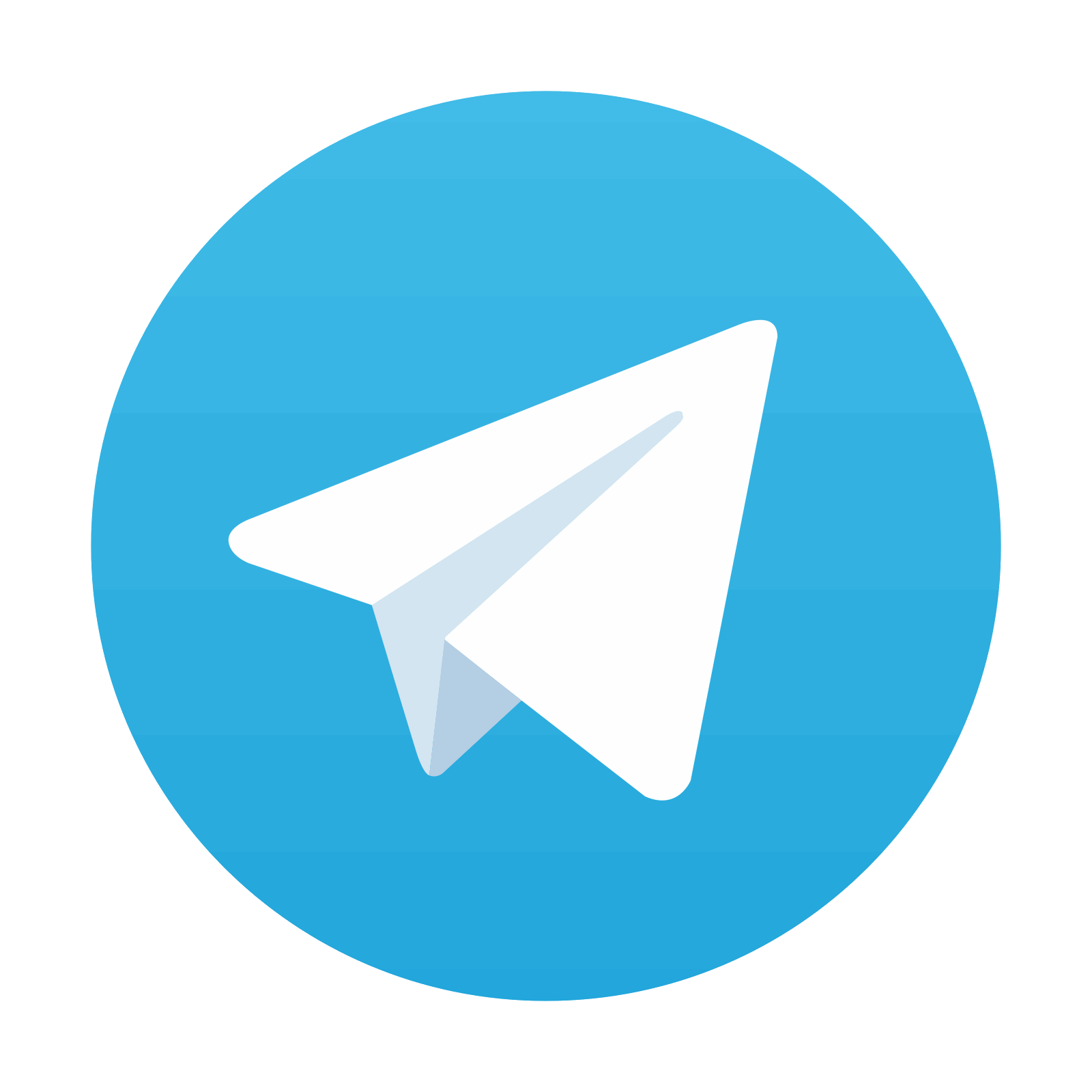
Stay updated, free articles. Join our Telegram channel

Full access? Get Clinical Tree
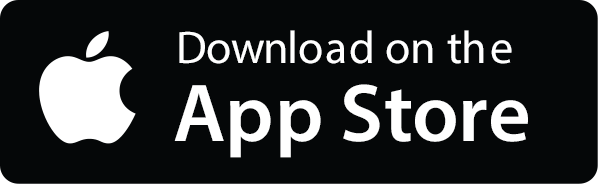
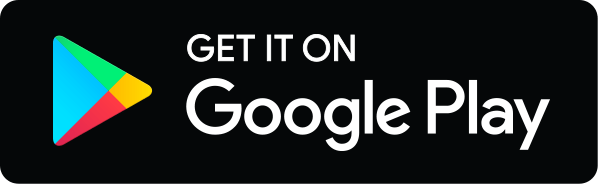