Several palliative neuromodulation treatment modalities are currently available for adjunctive use in the treatment of medically intractable epilepsy. Over the past decades, a variety of different central and peripheral nervous system sites have been identified, clinically and experimentally, as potential targets for chronic, nonresponsive therapeutic neurostimulation. Currently, the main modalities in clinical use, from most invasive to least invasive, are anterior thalamus deep brain stimulation, vagus nerve stimulation, and trigeminal nerve stimulation. Significant reductions in seizure frequency have been demonstrated in clinical trials using each of these neuromodulation therapies.
Key points
- •
Several neuromodulation options are available as palliative treatments for patients with medically refractory epilepsy.
- •
Vagus nerve stimulation, thalamic deep brain stimulation, and trigeminal nerve stimulation are currently the dominant nonresponsive treatment modalities in use.
- •
These different neurostimulation modalities all have a good safety profile and satisfactory rates of seizure reduction.
Introduction
A significant proportion of epilepsy patients is medically refractory, and apart from those who are candidates for resective surgery, most will continue to have disabling seizures for rest of their lives. Neuromodulation, or neurostimulation, is a palliative treatment option for many of these patients who are not eligible for resective surgery or who have persistent medically intractable refractory seizures despite previous epilepsy surgery. Patients typically have partial onset seizures, with or without secondary generalization, or less frequently, generalized epilepsies. Candidates for neuromodulation usually have a long-standing history of intractable epilepsy and have undergone extensive investigations, including video-electroencephalography (EEG), advanced neuroimaging, and even intracranial subdural or depth electrode implantations for seizure localization in many cases. Most of these patients have been found to have no single discrete seizure onset localization; a minority has seizure onsets localized to nonresectable areas of eloquent cortex, for example, within the boundaries of language centers in the dominant temporal or frontal lobes.
Neuromodulation as an alternate form of treatment for intractable epilepsy was first considered based on historical observations that electrical stimulation of subcortical structures could modify the cortical EEG: high-frequency stimulation “desynchronized” the EEG and low-frequency stimulation “synchronized” the EEG. Increased cortical synchrony mediated by low-frequency stimulation was demonstrated to be proepileptic, while cortical desynchronization mediated by high-frequency stimulation was shown to be antiepileptic. Experimental or clinical antiepileptic properties were subsequently reported with chronic electrical stimulation of several different central and peripheral nervous system sites, including the cerebellum, hypothalamus (mamillary nuclei), vagus nerve, trigeminal nerve, caudate nucleus, substantia nigra, centromedian thalamus, anterior thalamus, subthalamic nucleus, and the amygdalohippocampal region. In 1997, peripheral vagus nerve stimulation (VNS) was the first neurostimulation modality to be licensed for the treatment of patients with refractory epilepsy: controlled trials and subsequent widespread clinical usage have demonstrated significant, albeit modest, reduction in seizure frequencies.
Following the successes of VNS, and with the hope that direct stimulation of central nervous system structures might provide additional, more robust, benefit in terms of seizure control, interest was renewed in performing clinical trials of deep brain stimulation (DBS) for epilepsy. The initial clinical trials of DBS in epilepsy were performed in the 1970s and 1980s, mainly using cerebellar and, to a lesser extent, thalamic or caudate stimulation. DBS of the centromedian thalamic nucleus (CM) for intractable epilepsy was reported in several articles, with benefits in seizure control described in a most patients.
The mechanisms by which DBS may control seizures are largely hypothetical and unproven. CM stimulation, acting via the widely projecting nonspecific thalamic system, is hypothesized to act through induction of cortical desynchronization, preventing seizure propagation and generalization. Based on experimental data describing a “nigral control of epilepsy” system in rodents, controlled in large part by activity in the substantia nigra pars reticulata (SNpr), subthalamic nucleus stimulation has been proposed to act through disfacilitation of SNpr neurons, although there is no direct evidence for such a control system in primates. The anterior thalamus has been demonstrated to be involved in seizure propagation, both experimentally and clinically, and stimulation or lesioning of the anterior nucleus (AN) or its afferent pathways have been shown experimentally to have antiepileptic properties. The dorsomedial nucleus of the thalamus, situated posterior and inferior to AN, has also been shown to be involved in the maintenance and propagation of seizures, specifically those involving limbic brain structures. Anterior thalamus stimulation, aimed especially at AN, is thus hypothesized to act through blockade of corticothalamic synchrony, similar to CM. All of these proposed mechanisms are strictly hypothetical and, in fact, even the local effects of DBS are poorly understood. In a broad sense, most of the clinical effects of DBS can be considered to result from local inhibition of function, in that the effects are typically mimicked by lesions or application of inhibitory neurochemicals. Nevertheless, the mechanisms of local inhibition are unresolved, and it is possible that some effects of DBS could result from local neuronal or axonal excitation.
The exact parameters necessary to optimally alter the relevant corticothalamic networks with electrical stimulation are unknown, apart from the need for high-frequency stimulation (eg, ≥100 Hz). In the experimental models cited above, it is only high-frequency stimulation that shows antiepileptic properties, usually attributed to a cortical desynchronizing effect. In contrast, low-frequency stimulation tends to be proepileptic in experimental models, an observation conceptually linked to the increased synchronization in cortex that can be demonstrated through induction of the so-called recruiting rhythm with low-frequency thalamic stimulation.
In general, neuromodulation therapies have excellent safety profiles, and stimulation can, at least theoretically, be titrated to optimize seizure control. However, neuromodulation is not expected to provide freedom from seizures or antiepileptic medications. The aim of these palliative treatment modalities is to either reduce seizure frequency or prevent secondary generalization in order to minimize the many risks associated with intractable epilepsy. At present, the primary neuromodulation modalities in use for the treatment of patients with medically refractory epilepsy are AN DBS, VNS, trigeminal nerve stimulation (TNS), and responsive neurostimulation (RNS) ( Fig. 1 ). The authors have not included RNS in this review because it is discussed elsewhere in this issue. In reviewing each of the main nonresponsive treatment modalities, a further brief historical perspective is offered, followed by the rationale of therapy and the clinical results to date.

Introduction
A significant proportion of epilepsy patients is medically refractory, and apart from those who are candidates for resective surgery, most will continue to have disabling seizures for rest of their lives. Neuromodulation, or neurostimulation, is a palliative treatment option for many of these patients who are not eligible for resective surgery or who have persistent medically intractable refractory seizures despite previous epilepsy surgery. Patients typically have partial onset seizures, with or without secondary generalization, or less frequently, generalized epilepsies. Candidates for neuromodulation usually have a long-standing history of intractable epilepsy and have undergone extensive investigations, including video-electroencephalography (EEG), advanced neuroimaging, and even intracranial subdural or depth electrode implantations for seizure localization in many cases. Most of these patients have been found to have no single discrete seizure onset localization; a minority has seizure onsets localized to nonresectable areas of eloquent cortex, for example, within the boundaries of language centers in the dominant temporal or frontal lobes.
Neuromodulation as an alternate form of treatment for intractable epilepsy was first considered based on historical observations that electrical stimulation of subcortical structures could modify the cortical EEG: high-frequency stimulation “desynchronized” the EEG and low-frequency stimulation “synchronized” the EEG. Increased cortical synchrony mediated by low-frequency stimulation was demonstrated to be proepileptic, while cortical desynchronization mediated by high-frequency stimulation was shown to be antiepileptic. Experimental or clinical antiepileptic properties were subsequently reported with chronic electrical stimulation of several different central and peripheral nervous system sites, including the cerebellum, hypothalamus (mamillary nuclei), vagus nerve, trigeminal nerve, caudate nucleus, substantia nigra, centromedian thalamus, anterior thalamus, subthalamic nucleus, and the amygdalohippocampal region. In 1997, peripheral vagus nerve stimulation (VNS) was the first neurostimulation modality to be licensed for the treatment of patients with refractory epilepsy: controlled trials and subsequent widespread clinical usage have demonstrated significant, albeit modest, reduction in seizure frequencies.
Following the successes of VNS, and with the hope that direct stimulation of central nervous system structures might provide additional, more robust, benefit in terms of seizure control, interest was renewed in performing clinical trials of deep brain stimulation (DBS) for epilepsy. The initial clinical trials of DBS in epilepsy were performed in the 1970s and 1980s, mainly using cerebellar and, to a lesser extent, thalamic or caudate stimulation. DBS of the centromedian thalamic nucleus (CM) for intractable epilepsy was reported in several articles, with benefits in seizure control described in a most patients.
The mechanisms by which DBS may control seizures are largely hypothetical and unproven. CM stimulation, acting via the widely projecting nonspecific thalamic system, is hypothesized to act through induction of cortical desynchronization, preventing seizure propagation and generalization. Based on experimental data describing a “nigral control of epilepsy” system in rodents, controlled in large part by activity in the substantia nigra pars reticulata (SNpr), subthalamic nucleus stimulation has been proposed to act through disfacilitation of SNpr neurons, although there is no direct evidence for such a control system in primates. The anterior thalamus has been demonstrated to be involved in seizure propagation, both experimentally and clinically, and stimulation or lesioning of the anterior nucleus (AN) or its afferent pathways have been shown experimentally to have antiepileptic properties. The dorsomedial nucleus of the thalamus, situated posterior and inferior to AN, has also been shown to be involved in the maintenance and propagation of seizures, specifically those involving limbic brain structures. Anterior thalamus stimulation, aimed especially at AN, is thus hypothesized to act through blockade of corticothalamic synchrony, similar to CM. All of these proposed mechanisms are strictly hypothetical and, in fact, even the local effects of DBS are poorly understood. In a broad sense, most of the clinical effects of DBS can be considered to result from local inhibition of function, in that the effects are typically mimicked by lesions or application of inhibitory neurochemicals. Nevertheless, the mechanisms of local inhibition are unresolved, and it is possible that some effects of DBS could result from local neuronal or axonal excitation.
The exact parameters necessary to optimally alter the relevant corticothalamic networks with electrical stimulation are unknown, apart from the need for high-frequency stimulation (eg, ≥100 Hz). In the experimental models cited above, it is only high-frequency stimulation that shows antiepileptic properties, usually attributed to a cortical desynchronizing effect. In contrast, low-frequency stimulation tends to be proepileptic in experimental models, an observation conceptually linked to the increased synchronization in cortex that can be demonstrated through induction of the so-called recruiting rhythm with low-frequency thalamic stimulation.
In general, neuromodulation therapies have excellent safety profiles, and stimulation can, at least theoretically, be titrated to optimize seizure control. However, neuromodulation is not expected to provide freedom from seizures or antiepileptic medications. The aim of these palliative treatment modalities is to either reduce seizure frequency or prevent secondary generalization in order to minimize the many risks associated with intractable epilepsy. At present, the primary neuromodulation modalities in use for the treatment of patients with medically refractory epilepsy are AN DBS, VNS, trigeminal nerve stimulation (TNS), and responsive neurostimulation (RNS) ( Fig. 1 ). The authors have not included RNS in this review because it is discussed elsewhere in this issue. In reviewing each of the main nonresponsive treatment modalities, a further brief historical perspective is offered, followed by the rationale of therapy and the clinical results to date.
Anterior nucleus deep brain stimulation
Historically, Cooper and Upton first reported on AN DBS for refractory complex partial seizures. In the subsequent decades, a small number of case series reported an acceptable efficacy and safety profile : among the 19 total patients described in these pilot studies, there was a mean reduction in seizure frequency of 35% at 1 year (n = 19), 61% at 2 years (n = 13), and 65% in long-term follow-up (3–7 years; n = 14). This mean reduction in seizure frequency paved the way for a large multicenter randomized trial: Stimulation of Anterior Nucleus of Thalamus for Epilepsy (SANTE), which showed nearly identical results to those described in the collected pilot studies ( Table 1 ). Currently, this procedure is approved for the treatment of refractory epilepsy in Europe and Canada but not in the United States.
Modality | Patients (Refs.) | Responder Rate (>50% Seizure Reduction) 1 y, % | Responder Rate (>50% Seizure Reduction) 2 y, % | Responder Rate (>50% Seizure Reduction) Last Visit (y), % | Average Reduction in Seizure Frequency 1 y, % | Average Reduction in Seizure Frequency 2 y, % | Average Reduction in Seizure Frequency Last Visit (y), % |
---|---|---|---|---|---|---|---|
DBS (AN) | 110 | 43 | 54 | 68 (5) | 41 | 56 | 69 (5) |
VNS | 65, 90 | 44 | 59 | 64 (5) | 36 | 52 | 56–66 (5) 76 (10) |
TNS | 35 | 37 | — | — | 37 | — | — |
RNS | 191, 230 | 44 | 53 | 56–58 (3–6) | 44 | 53 | 48–66(3–6) |
Rationale
The AN forms part of the limbic circuit and is connected to the hippocampus via the mammillothalamic tract and the fornix. This nucleus has abundant thalamocortical projections (to the cingulate gyrus, posterior parietal cortex, insular region, and lateral temporal cortex), making it an attractive target for modulation of seizure activity. Combined EEG-functional MRI studies have shown involvement of the AN in the initiation and propagation of seizures in human idiopathic generalized epilepsy. Mirski and colleagues investigated seizure control after lesioning or electrical stimulation of the mammillothalamic tract or AN: in the pentylenetetrazol (PTZ) rat model, seizure threshold increased with high-frequency (but not low-frequency) stimulation of AN. Similarly, in a sheep model of penicillin-induced epilepsy, only high-frequency stimulation reduced spontaneous and induced epileptic activity. The mechanism underlying this efficacy of AN DBS could involve activation of the inhibitory corticothalamic projections.
The implantation of electrodes for AN DBS is performed using frame-based stereotaxy. In a parasagittal projection (4–5 mm from midline), the AN is located dorsal and anterior to the mammillothalamic tract. Intraoperative microelectrode recordings may aid electrode implantation by confirming the exit from the ventricle and entry into the thalamus.
Clinical Results
The early case series described acceptable safety profiles and benefits in seizure control in patients with various types of medically refractory epilepsy. The reported mean seizure reduction rates varied between 50% and 90%. In the SANTE trial, 110 patients underwent implantation of DBS electrodes in the AN and were randomly assigned to stimulation ON (5 V, pulse width 90 μs, frequency 130 Hz, duty cycle 1 minute ON/5 minute OFF) or stimulation OFF for a 3-month blinded phase, after which all patients received AN stimulation (open-label phase). In the blinded phase, patients with active AN stimulation reported a 40% decrease in seizure frequency, versus a 15% decrease in the stimulation OFF group, a significant difference ( P = .002). In long-term (5 year) follow-up, the mean reduction in seizure frequency was 69%, and 68% of patients reported 50% or better reduction in seizure frequency. In the SANTE trial, AN DBS was well tolerated in most patients and unassociated with somatomotor side effects. Memory disturbance was the most commonly reported side effect, likely related to stimulation-induced alterations in the circuit of Papez.
Vagus nerve stimulation
Historically, the inhibition of motor activity by stimulation of vagal afferents was first reported in 1937 by Schweitzer and Wright. Left VNS was approved by the US Food and Drug Administration (FDA) in 1997 for the treatment of medically refractory partial onset seizures.
Rationale
The vagus nerve is generally considered a parasympathetic nerve, but it also delivers sensations from the viscera. These visceral afferents project diffusely to cortex and subcortical regions, particularly the thalamus, via the medial reticular formation. In patients with efficacious stimulation, decreased metabolic activity (assessed with technetium- hexamethylpropyleneamine oxime single-photon emission computed tomography) was observed bilaterally in the medial thalamus during the ON stimulation state. VNS at clinically relevant parameters may also result in stimulation of unmyelinated C fibers (nociceptive fibers) leading to an arousal-like effect. Zabara studied VNS in canine models of strychnine -or PTZ-induced seizures. The stimulation (40 V, frequency 60 Hz, pulse width 0.6 ms) was applied 1 to 3 minutes after the onset of motor seizure activity. The investigator reported a stimulation-induced interruption of seizure activity in a timely fashion (the longest interval observed was 14 seconds from the start of stimulation). The most effective parameters were intensity greater than 20 V, frequency higher than 20 Hz, and an approximate stimulus duration of 0.2 ms. McLachlan, using the penicillin seizure model in rats, reported a significant decrease in interictal spike activity, mean spike frequency, and amplitude with stimulation of the left vagus nerve. This effect persisted a few seconds after stimulation. Interestingly, a similar effect on spike frequency was seen in response to heating the rats’ tails, suggesting that the antiepileptic effects of stimulation were not necessarily specific to the vagus nerve. Chronic stimulation of the right vagal branch in a monkey model was also shown to reduce seizure frequency. Clinically, however, the left vagus nerve is the preferred site of stimulation because it preferentially innervates the cardiac ventricles and is associated with a lesser risk of cardiac side effects.
Clinical Results
In a double-blind randomized controlled trial of VNS in a pediatric population (41 children with both localization-related and generalized epilepsy), the comparison groups were allocated to 2 different stimulation parameters: a treatment group: 0.25 mA, 30 Hz, 30 seconds ON/5 minutes OFF, and a control” group: 0.25 mA, 1 Hz, 14 seconds ON/60 minutes OFF. After the initial phase, the first group received stimulation at the maximum tolerated current (maximum 1.75 mA), whereas in the second, control group, the output current was initially increased during the clinic visit but was then switched back to 0.25 mA at discharge. After the 20-week observation period, an overall reduction in seizure frequency and severity was observed as compared with baseline, without a statistically significant difference between the treatment and control groups. In a slightly different strategy, a large multicenter randomized trial (E03; 114 adult patients enrolled from 17 institutions) evaluated the effects of chronic stimulation and also the on-demand magnet activation strategy of the VNS device by instructing patients to activate the device during an aura. The participants received 2 different stimulation parameters: high stimulation group: up to 3 mA, 20–50 Hz, 500 μs, ON 30–90 s/OFF 5–10 minutes, and low stimulation group: up to 2.75 mA, 1 Hz, 130 μs, ON 30 s/OFF 60–180 minutes. After 14 weeks of stimulation, a significantly higher reduction in mean seizure frequency was observed in the high-stimulation group (24% vs 6%, P = .01). Another large randomized trial of chronic stimulation involving 196 patients (E05) with similar study parameters was associated with a greater reduction in seizure frequency in the high-stimulation group (28% vs 15%, P <.05), especially among patients with complex partial seizures. Regarding the effectiveness of the magnet intervention (ie, patient-controlled activation of the device near seizure onset), most patients thought the on-demand intervention to be effective, although there was no difference in reported efficacy between patient groups assigned to true activation versus sham activation (device programmed to 0 mA for magnet activation in the latter group).
The VNS surgical procedure carries a relatively low risk. Common stimulation-related side effects (eg, cough, voice alteration, throat paresthesias) are typically mild and can be reversed by adjusting the stimulation parameters. The long-term follow-up data reveal a persistent benefit in seizure control that, similar to AN DBS, has been reported to gradually improve over time (see Table 1 ).
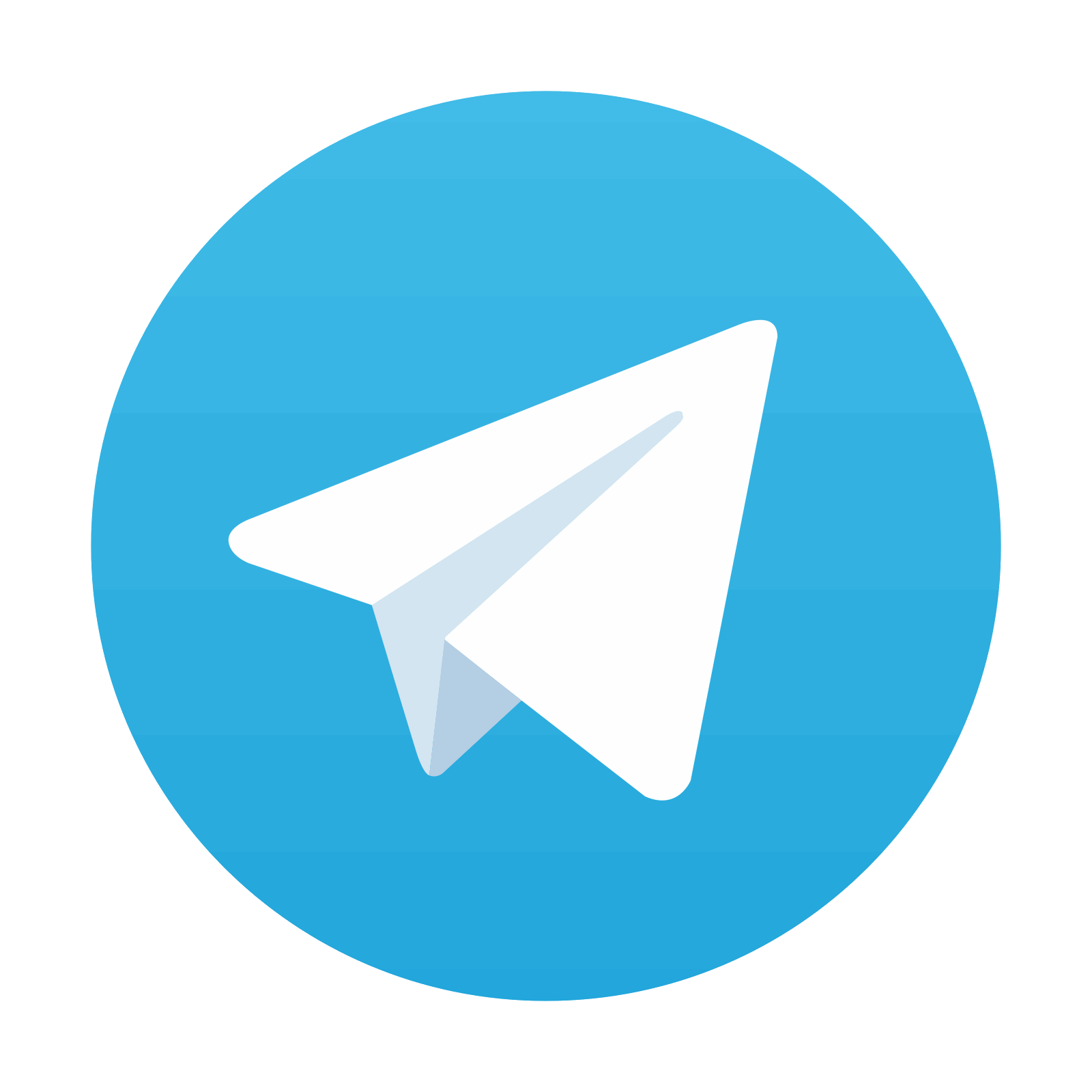
Stay updated, free articles. Join our Telegram channel

Full access? Get Clinical Tree
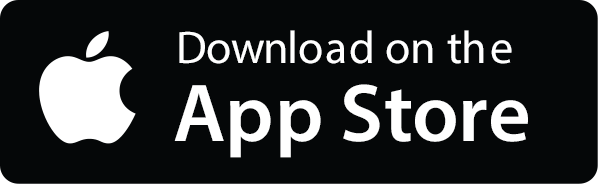
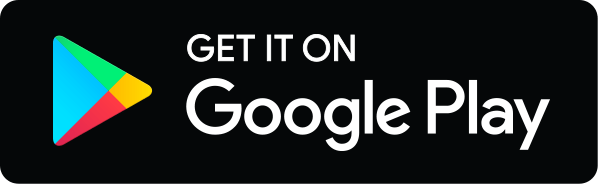