and Michele Salanova1
(1)
Vegetative Anatomy, Charité Universitätsmedizin Berlin, Berlin, Germany
Abstract
Skeletal muscle adaptations in different environment conditions are particularly sensed at the neuromuscular junction (NMJ), the most distal connection of the neuromuscular system to peripheral target muscles. For example, in all models of muscle disuse atrophy (e.g., bed rest, denervation, limb immobilization, unloading), the NMJ experiences disruptive structural adaptations that are very similar to those observed during exposure to microgravity (μG) in Space. Thus, prolonged disuse and μG both exert adverse effects on the determination of skeletal muscle mass. However, studies on the molecular mechanisms of the normal neuromuscular system including the NMJ in disuse and in different adaptation conditions are scarce. The NMJ on its own is a highly specialized cell-cell communication contact between a nerve (axon terminal) and a muscle fiber (myofiber) providing the basis for the appropriate peripheral motor control of the neuromuscular system. Altered muscle contraction activity more extensively stimulate NMJ remodeling with synaptic microstructure and size changes. The most relevant changes include decreased synaptic vesicle density and neurotransmitter content, but also axon terminal de-/regeneration with regression and sprouting mechanisms. Previous studies in Space reported microgravity-induced cellular and molecular changes at spaceflown rodent NMJs. We found candidates of the Homer protein family (previously found at central synapses of brain) also expressed at the NMJ subsynaptic microdomain region of human skeletal muscle that were however lost in disuse and upregulated by exercise in bed rest, suggesting activity-driven Homer expression mechanisms and functions with unique roles in normal NMJ function and adaptation.
Keywords
Skeletal muscle innervationNeuromuscular junctionHomer proteinsDisuseExercise3.1 Introduction
Skeletal muscles, and in particular those of the lower limb or antigravity postural muscles (Fig. 2.1), undergo several adaptive processes during altered muscle activity or spaceflight missions which are mostly characterized by structural and metabolic changes that are consequently largely responsible for the altered skeletal muscle structure and function. The μG-induced muscle atrophy is well known during spaceflight programs (in a time-dependent manner), as well as the related reduction in motor performance control in most of the crew members which are responsible for the decrement in skeletal muscle strength and in resistance to fatigue that severely affected both “inflight” and “extravehicular” crew members’ activity. Moreover, morphological alterations of the neuromuscular junction (NMJ) in response to reduced neuromuscular activity and the role of exercise in maintaining the integrity have been extensively investigated since the beginning of the human space program (Wilson and Deschenes 2005; Nishimune et al. 2014).
By using animal experimental models exposed to μG, it was recently shown that different types of muscles experience a greater degree of atrophy than others. In particular, in some of the studies, the high degree of muscle atrophy well correlates with the presence of the slow-type myosin heavy chain suggesting the involvement of a greater proportion of slow-twitch fibers (see Sect. 2.1.5). By contrast, in other reports, atrophy is similarly described in both slow- and fast-twitch fibers, as previously reviewed by Wilson and Deschenes (2005).
One proposed explanation for the relative atrophic response elicited in different muscles is that μG induces a shift in “neuronal recruitment patterns” of motor units (Wilson and Deschenes 2005; Fitts et al. 2001). Thus, we may conclude that nerve endings’ remodeling may play an additional and synergistic role in response to altered skeletal muscle loading or altered contraction activity during spaceflight, and the altered neuron-derived specific signals (electrical and/or chemical transmitters) might be, at least in part, responsible for the microgravity-induced effects at the level of single skeletal muscle fiber.
In an attempt to further elucidate the molecular mechanisms which are involved during disuse-induced muscle atrophy, we investigated whether or not scaffold adapter proteins of the Homer family were involved in skeletal muscle plasticity changes. Thus, by confocal immunohistochemistry tools, we explored soleus and vastus lateralis NMJs of healthy subjects that underwent long-term bed rest muscle disuse without and with two different exercise countermeasures. The novel results of our experiments suggested that components of the Homer family are specifically expressed at the NMJ postsynaptic regions of postural skeletal muscles of healthy human subjects and, surprisingly, they were particularly differently regulated at the protein and mRNA level by muscle activity and inactivity (Salanova et al. 2011).
3.2 Where the Nerve Meets the Muscle: Neuromuscular Synapse
Skeletal muscle contraction is under the tight control of motor nerve activity by means of a unique muscle and nerve common structure named the “neuromuscular synapse” or “neuromuscular junction” (Fig. 3.1).
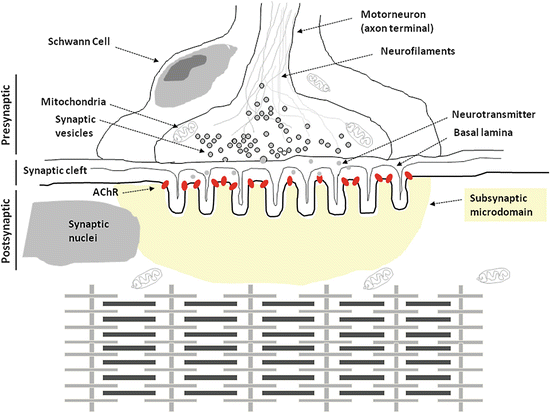
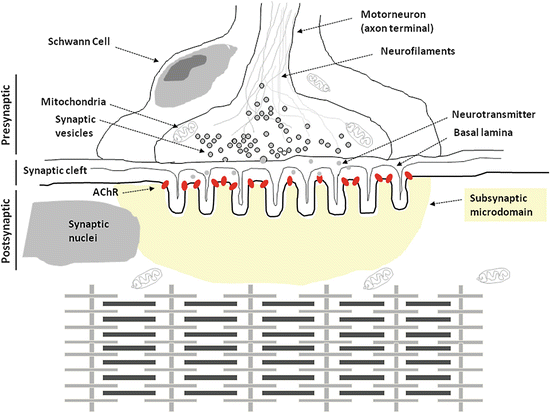
Fig. 3.1
Schematic diagram representing the different compartments of the skeletal muscle NMJ and the contractile apparatus (myofibrils with sarcomeres in series) of the myofiber sarcosol underneath
The NMJ or motor endplate could be functionally and anatomically divided into four distinct regions: a presynaptic, a synaptic (cleft), a postsynaptic region, and a subsynaptic microdomain.
3.2.1 Presynaptic Structure and Function
Every myelinated motor axon reaches its peripheral target muscle where it divides into several unmyelinated terminal fibers each of which directly innervates a single myofiber. Altogether, the terminal fibers of a given single motoneuron and all myofibers that are under its tight control are considered as a single motor unit (Sanes and Lichtman 1999). Basically, the terminal fibers contain both potassium and sodium channels which are important for the control of the duration and amplitude of the membrane action potential. The sodium channels however are absent in proximity of the nerve terminal. The nerve terminal contains synaptic vesicles each of which contain several thousand molecules of the typical neurotransmitter acetylcholine (ACh). The content of a single presynaptic vesicle is considered to reflect a “quantum” of the neurotransmitter being released upon motor nerve stimulation (Sanes and Lichtman 1999).
Once the action potential reaches the nerve terminal, the signal is further propagated in the cell by the opening of voltage-gated calcium channels which are responsible for a series of events at the nerve terminal which end up with the fusion of synaptic vesicles with the plasma membrane and the release of their quanta of ACh into the synaptic cleft (Fig. 3.1). These late events are particularly regulated by the presence of soluble N-ethylmaleimide sensitive factor attachment receptor or SNARE complex (Hong and Lev 2014). The duration of the nerve depolarization will determinate at the end the amount of calcium influx into the nerve terminal and the amount of neurotransmitter ACh will be released, and this appears to be specifically limited by the voltage-gated and calcium-dependent potassium channel activation. Thus, each action potential results in the exocytosis from several tens to few hundreds of synaptic vesicle which are largely in a supernumerary capacity needed to reach a sufficient number for the postsynaptic ACh receptor (AChR) threshold (Sanes and Lichtman 1999).
3.2.2 Synaptic Cleft Structure and Function
The space between the nerve terminal membrane and the myofiber plasma membrane or sarcolemma is called “synaptic cleft” which is a relatively wide (approx. 50 nm) intermembrane gap as compared to those found in typical central synapses (approx. 5–10 nm) of brain and spinal cord (Fig. 3.1). A peculiar microstructure typical for the NMJ is represented by a synaptic cleft basal lamina, a 3D micro network of loose extracellular matrix molecules and thin filaments that allow for transmitter diffusion but also provide mechanical link (via laminins and fibronectin anchoring proteins) between the pre- and postsynaptic membrane required to withstand mechanical stress in normal muscle tone/tension control, but also by contraction shortenings of the myofibers inside (Nishimune et al. 2004). The synaptic basal lamina is also the location of the acetylcholine esterase (AChE). Once ACh is released from the presynaptic vesicles, the neurotransmitter quickly spreads by diffusion in the synaptic cleft within a few microseconds in order to reach the specific postsynaptic membrane receptors (AChR). The transmitter signal is turned off by AChE enzyme activity present in the synaptic cleft that specifically hydrolyzes the ACh (Aldunate et al. 2004; Gaspersic et al. 1999) neurotransmitter (i.e., acetyl residue plus choline) for instant reuptake by the presynaptic nerve terminal for de novo transmitter synthesis.
3.2.3 Postsynaptic Membrane Structure and Function
The opposite part of the nerve terminal in the close vicinity of the synaptic cleft is called the postsynaptic membrane which actually is a specialized part of the outer muscle fiber membrane or sarcolemma. At this region, the sarcolemma forms several folds and troughs typical of the NMJ (Fig. 3.1). The “entrance” of the little troughs is occupied by the nicotinic acetylcholine receptors (nAchR) at a concentration range approx. of 10.000–20.000 receptors per mm2 of postsynaptic surface area, at least in a concentration range of about 1:1000 compared to extrasynaptic sarcolemmal regions of a normal myofiber (Fig. 3.1). The strong nAChR density at this region is to ensure for optimal postsynaptic neuromuscular transmission.
Normally, the nAChR consist of pentameric transmembrane subunits which cluster together forming a pore that in absence of ACh molecules is maintained in a closed state in order to ensure uncontrolled flow-through of cations. In the presence of ACh which selectively binds the N-terminal domain of the nicotinergic AChR a1 subunit, exactly two molecules of ACh are required to activate the receptor (Harpsoe et al. 2011; Gotti et al. 2009). Then, the channel pore opens, and sodium moves into the muscle cell and allows for an action potential to propagate forward on the sarcolemmal membrane. Once the action potential reaches the t-tubules (TT), it activates the voltage-gated ion channel dihydropyridine receptor 1 alpha (DHPR1α) by inducing conformational changes that, in turn, modify its physical interaction with the intracellular calcium release channel ryanodine receptor type 1 (RyR1) which is positioned at the sarcoplasmic reticulum terminal cisternae (TC) (Flucher and Franzini-Armstrong 1996) at the interface between the TT (Fig. 2.4). As RyR1 physically dissociates from DHPR1α, it becomes activated and opens providing most of the calcium influx required for muscle contraction (Fig. 2.19). RyR1 exist in the cell as a macromolecular complex whose regulation is under the tight control of different signaling pathways. Altered RyR1 regulation is considered to be a cause of several clinical skeletal muscle myopathies such as central core disease (CCD) or malignant hyperthermia (MH) (Brislin and Theroux 2013; Pietrini et al. 2004).
3.3 Molecular Mechanism of NMJ Adaptation to Disuse and Exercise
There is a large body of evidence suggesting that skeletal muscles, and in particular lower limb and antigravity postural muscles, undergo several adaptive processes during prolonged inactivity or unloading during exposure to microgravity (μG) mostly characterized by structural and metabolic changes, which thereafter might be largely responsible for the altered skeletal muscle structure and function. For instance, the μG-induced muscle atrophy is well known since the beginning of the human space program, as well as the related reduction in motor performance in astronauts, which is the likely one cause for the decrement in skeletal muscle mass and strength and in resistance to fatigue, severely affecting both “inflight” and “extravehicular” crew members’ mission duties.
Needless to say that the beneficial effects of exercise for the musculoskeletal quality and neuromuscular control are well accepted today. However, studies on mature NMJs of normal adult skeletal muscle showed continuous remodeling mechanisms even under physiological conditions suggesting a more or less continuum microstructural representation in normal healthy skeletal muscle depending on everyday moderate activities and fitness, throughout human life performances, and even chaperoning healthy aging on Earth that need to be considered for investigations. For instance, extended periods of increased or decreased neuromuscular activity more extensively stimulate remodeling, including changes in the overall NMJ microstructure and size. Recently, the role of exercise in maintaining the integrity of the NMJ at molecular level has been extensively reviewed (Nishimune et al. 2014). Overall, the results suggest that NMJ hypertrophy and the increase in active zone protein levels in exercised young, adult, and aged NMJs are all localized effects within the exercised muscles and motoneurons innervating exercised muscles (Nishimune et al. 2014).
Interestingly, in human skeletal muscle, resistive exercise training upregulates the expression of laminin β2 at mRNA and protein level (Gordon et al. 2012; Hunter et al. 1989) which, in turn, may play a role in the effect of exercise that increases the level of active zone protein Bassoon in aged NMJs (Nishimune et al. 2012). Laminin β2 is an extracellular matrix protein that is secreted by muscles and is concentrated specifically at the synaptic cleft basal lamina of NMJs (Nishimune et al. 2004; Hunter et al. 1989) which binds specifically P/Q- and N-type voltage-dependent calcium channels (VDCCs) (Nishimune et al. 2004). Several in vivo studies have demonstrated that the interaction between laminin β2 and VDCCs is responsible for organizing the NMJ active zones. The number of active zones, in fact, was decreased when the interaction between the VDCCs and laminin β2 was experimentally perturbed in wild-type mice (Nishimune et al. 2004). Consistent with the above reports, P/Q- and N-type VDCC double-knockout mice exhibited specific defects in the number of active zones and docked synaptic vesicles, which were twice as severe as the defects observed in the P/Q- and N-type VDCC single-knockout mice (Nishimune et al. 2004; Chen et al. 2011). Moreover, humans who carry laminin β2 mutations result in active zone loss and denervation in addition to the development of the “Pierson syndrome,” an autosomal recessive movement disorder associated with microcoria, and nephrotic syndrome (32, 49). These results suggest that laminin β2 binds to synaptic VDCCs to organize the active zones. Recently, studies showed that NMJ active zones are maintained at a constant density as the NMJ matures but are degraded in aged animals (Chen et al. 2012). On the same line of evidence, the expression level of Bassoon decreases in the NMJs of aged mice and rats (Nishimune et al. 2012; Chen et al. 2012). A lack of Bassoon is known to impair synaptic vesicle trafficking to presynaptic membranes in the central nervous system and sensory neurons (Hallermann et al. 2010; Mukherjee et al. 2010; Frank et al. 2010). Furthermore, a lack of Bassoon decreases VDCC Ca2+ influx and weakens synaptic transmission, because of the direct interaction between VDCCs and Bassoon that enhances the P/Q-type VDCC function (Nishimune et al. 2012). This modification to VDCCs by Bassoon is similar to the effect of another active zone protein such as RIM1 on VDCCs (Kiyonaka et al. 2007; Uriu et al. 2010). If confirmed, these findings suggest that active zone protein loss may be a part of the molecular mechanism that causes the deterioration of aged NMJs. Active zone deterioration in aged NMJs is ameliorated by muscle exercise. Two months of isometric force training rescued the loss of Bassoon in aged NMJs in the genioglossus muscle of 2-year-old rats (Nishimune et al. 2012). However, exercise training did not alter NMJ size, which suggests that the increase in the Bassoon immunohistochemistry signal in aged NMJs reflects an increase in the protein quantity in each nerve terminal (Nishimune et al. 2012). The mean intensity of the Bassoon immunohistochemistry signal in the NMJs of exercised aged rats is similar to the mean intensity observed in young adult rats. Importantly, the improvement in Bassoon protein level observed in exercised and aged NMJs is consistent with improvements observed using electrophysiology in NMJ function after endurance training in aged mice (Fahim 1997). In summary, exercise-induced upregulation of laminin β2 may play a role in preservation of active zones in aged NMJs, which more likely exerts a positive effect on NMJ synaptic transmission.
3.4 Neuromuscular System Adaptation to Spaceflight
Since the beginning of the “human space program,” most of the space research activity has dealt with the effect of μG on bone and skeletal muscle adaptation. For skeletal muscle in particular, most of the studies were made primarily at the physiological and behavioral levels, while only few studies so far investigated molecular and biochemical NMJ adaptation to μG exposure. Nevertheless, these studies however reported that μG during spaceflight induced disturbance in neuromuscular transmission together with a significant decrement in muscle strength (Deschenes et al. 2005).
Data recently available on the histologic and ultrastructural level generally reported that one of the most disruptive NMJ adaptations to μG is axonal disintegration and substantial decrease in synaptic vesicle density and neurotransmitter content, although small quantitative differences were often present between different types of muscle (Baranski and Marciniak 1979; Deschenes et al. 2005). Changes in NMJs of antigravity postural muscles such as soleus (SOL) or adductor longus, as reported, were always more pronounced than other muscles such as extensor digitorum longus (EDL) or gastrocnemius (GAS) (Riley et al. 1990). Interestingly, by using in vivo animal experiments, it was proposed that resistance training in rat SOL is able to remodel structure and size of the NMJ by increasing nerve terminal branching during muscle unloading (Deschenes et al. 2000, 2006). Moreover, neuromuscular adaptations in spaceflown rats were further investigated (Deschenes et al. 2005), which confirmed that these changes are present in particular in postural muscles or antigravity muscles such as the soleus (SOL) and were clearly manifested at the neuromuscular synapse. Thus, the general emerging picture is that under exposure to μG, NMJ experiences disruptive structural adaptations similar to those observed in several models of muscle disuse atrophy on ground such as bed rest inactivity, limb immobilization, denervation, or animal model of hind-limb unloading. Such muscle adaptations might be further accompanied at the level of NMJ by molecular modifications of specific cell signaling pathways and scaffold adapter proteins particularly involved in the synaptic transmission.
3.5 Role of Scaffold Adaptor Homer Proteins in Skeletal Muscle
So far there are several studies suggesting the importance of Homer protein family in skeletal muscle physiology (Stiber et al. 2005). For instance, specific isoforms of the Homer family have been proposed to directly modulate the intracellular calcium release channel ryanodine receptor 1 (RyR1) activity (Feng et al. 2008). Moreover, binding of Homer modulates the activity of various Ca2+ channels, whereas the formation of multimers allows cross-talk between different surface membrane receptors and Ca2+ channels in the membrane of intracellular compartments.
Primarily Homer proteins were originally isolated from central nervous system (CNS) neurons as one of the several proteins whose expression is upregulated upon synaptic activity induced by seizure or during induction of long-term potentiation (Shiraishi-Yamaguchi and Furuichi 2007). Homers can bind proline-rich regions present on target proteins through their EVH1 domains and cross-link them through their coiled-coil domains discussed in a recent review (Salanova et al. 2013). The Homer short forms, including Homer1a, the IEG, that directly modify synaptic plasticity (Shiraishi-Yamaguchi and Furuichi 2007) cannot form multimers, but they can still bind to target proteins through their EVH1 domain thereby modifying protein function. Thus, Homer1a disrupts signaling complexes, and therefore, this protein isoform has been proposed to function by acting as a negative regulator of the long forms of Homers (Xiao et al. 1998; Roche et al. 1999).
The EVH1 domain selects a specific consensus binding site within different target proteins that represents a short proline-rich amino acid sequence, PPXXΦ (where X is any amino acid and Φ is any aromatic amino acid), with charged residues on either side of the sequence (Salanova et al. 2013). Database screening analysis showed that there is a long list of potential candidates of Homers interacting proteins, which contained a poly-proline-rich consensus sequence. Some functional proteins were already shown to interact with Homers, e.g., the G-protein-coupled metabotropic glutamate receptor (mGluR) and several Ca2+-signaling proteins, including Shank, PLCb, IP3Rs, TRPC channels, RyR, several L-type Ca2+ channel isoforms and the nuclear factor of activated T-cells (NFAT) (Huang et al. 2008).
Homers regulate the myogenic program since the transient expression of specific Homer protein isoforms precedes myoblast fusion and thus myotube formation/skeletal muscle differentiation (Stiber et al. 2005). Further evidence supporting this hypothesis comes from findings indicating that Homer 2 mRNA and protein were found to be transiently expressed at high levels in whole embryo at the stage E14.5, particularly in the brain and hind limbs (Stiber et al. 2005). Further biochemical evidence for an effect of Homer 2b isoform on muscle differentiation was ascertained by examining the expression of RyR1 and of the myoglobin promoter, two well-established muscle-specific differentiation markers. Moreover, C2C12 cells (mouse cell line) expressing Homer 2b augmented basal NFAT transactivation and myoglobin promoter in the order of 2.5-fold greater than of empty vector transfected control cells. Interestingly, co-expression of both activated calcineurin and Homer proteins resulted in a synergistic activation (16-fold) of the myoglobin promoter (Stiber et al. 2005). A proposed mechanism was that Homer might play a role as a molecular switch mediating both IP3R and RyR signaling to promote myotube differentiation program through the NFAT signaling pathway. Thus, Homer proteins potentially represent the first example of a family of scaffolding proteins, which are involved in modulating Ca2+-dependent gene expression during muscle differentiation (Stiber et al. 2005).
An additional role of Homer protein family concerning the regulation of excitation-contraction (E-C) coupling was also proposed and suggested by the presence of a Homer binding motif in all RyRs and voltage-dependent L-type Ca2+ channels. This hypothesis was further confirmed by specific Ca2+ release measurement experiments in permeabilized isolated skeletal muscle fibers as well as by the analysis of the open probability (Po) of the RyRs channels inserted into lipid bilayers, which showed that Homers are able to activate both types of ryanodine receptor Ca2+ channels RyR1 and RyR2. Other studies reported that co-expression of Homer1a with RyR2 and Cav1,2 enhances, whereas co-expression of Homer1b with RyR2 and Cav1,2 reduces, the efficiency of Ca2+-induce Ca2+ release (CICR) in an in vitro cell model (Huang et al. 2007).
3.5.1 Mice with a Specific Deletion of Homer1 Develop Skeletal Muscle Myopathy
Homer1 interacts with several members of the transient receptor potential classic (TRPC) family acting as a scaffolding protein and modulating the formation of a large protein complex which involves TRPC1 and IP3R channels. Recently, Stiber et al. (2008) reported that Homer1−/− mice exhibit TRPC1 abnormalities such as altered ion channel activity, which leads to a pronounced skeletal muscle myopathy, as revealed by myofiber cross-sectional area analysis. Furthermore, Homer1−/− (KO) mice exhibited a decrease in skeletal muscle force contractility (Stiber et al. 2008). It was speculated that Homers might be localized at the NMJ and lack of Homer1 in KO mice appeared to mimic mechanisms of denervation (Stiber et al. 2008). However, the first evidence of Homers at NMJs in human and rodents was found and described more recently by our group (Salanova et al. 2011).
3.5.2 Skeletal Muscle Regeneration Characterized by Transition of Homer-specific Isoforms
Transition in Homer isoforms under resting conditions and under short- and long-term muscle adaptation has been previously investigated (Bortoloso et al. 2006). Interestingly, a switch in Homer-specific isoforms was proposed to be associated with muscle differentiation and regeneration mechanisms (Bortoloso et al. 2006). For instance, constitutively expressed Homer1a is transiently upregulated during myofiber regeneration and myotube formation. By contrast, Homer1b/c/d, −2b expression positively correlated with changes in muscle mass (Bortoloso et al. 2006). Therefore, a pivotal role of Homers in skeletal muscle plasticity and adaptation appears very likely.
3.5.3 Regulation of Homer Expression in Denervated Myofibers
By using a rat sciatic denervation model, the expression of Homer1b/c and Homer2a/b was investigated in detail (Bortoloso et al. 2013). Only Homer2 was shown to be downregulated at mRNA and protein level in atrophic muscle after 7 days of permanent denervation (in vivo unilateral sciatic nerve transection), suggesting a neuronal control of Homer expression in skeletal muscle. Interestingly, reconstitution of Homer2 by in vivo transfection experiments in denervated soleus partially rescued the atrophic phenotype (Bortoloso et al. 2013).
3.5.4 Hind-Limb Unloading (HU) Decrease Homer Expression
The expression of specific Homer isoforms has been investigated in rat soleus following hind-limb unloading (Bortoloso et al. 2013). Seven days of HU were sufficient to decrease Homer2 protein expression, which in turn affects the ubiquitination and thus, indirectly, the transcriptional activity of atrophy-related genes such as MuRF1, atrogin, and myogenin (Bortoloso et al. 2013). In fact, downregulation of Homer2 appears to be an early event in muscle atrophy.
Additional studies from our group using the HU model showed that loss of Homer proteins at the NMJ of rat soleus after 21 days of HU was clearly attenuated by an “active mode motion” (Salanova et al., manuscript in preparation); an exercise protocol which is characterized by spontaneous muscle contractions in HU rats entrained by electric impulse stimulation of the hind foot paw (Sun et al. 2013). Whereas, “passive mode motions” (an exercise protocol triggered by mechanically-induced hind limb lifting passively (Sun et al. 2013)) did not show similar effects (Salanova et al., manuscript in preparation).
3.5.5 Regulation of Myogenic Differentiation Program by Homer
Recently, it has been proposed that Homer-specific isoforms are regulators of myotube formation and, thus, inducers of the myogenic differentiation program. Additional evidence supporting this hypothesis stems from results of Homers that were found to be associated with several regulatory proteins, including Drebrin, a F-actin side-binding protein, and the small GTPase Cdc42 (Mancini et al. 2011). Moreover, Homer2b was found to be in the calcineurin/nuclear factor of activated T cells (NFAT) pathway and to enhance myoblast differentiation and myotube formation (Stiber et al. 2005).
3.5.6 Homer Localize in Skeletal Muscle-Specific Subcellular Compartments
Anti-pan Homer antibodies revealed two different immunofluorescence patterns. In longitudinal sections, a cross-striated sarcomeric labeling at the Z-line/costamere level was present, corresponding to the longitudinal sarcoplasmic reticulum cisternae (LSR) (Salanova et al. 2002). A second, moderate but discrete Homer immunolabeling punctuate pattern was observed at the NMJ corresponding to the NMJ subsynaptic microdomain. As shown in Fig. 3.2, α-bungarotoxin labeling of AChRs localized adjacent to the Homer antigens (Salanova et al. 2011).
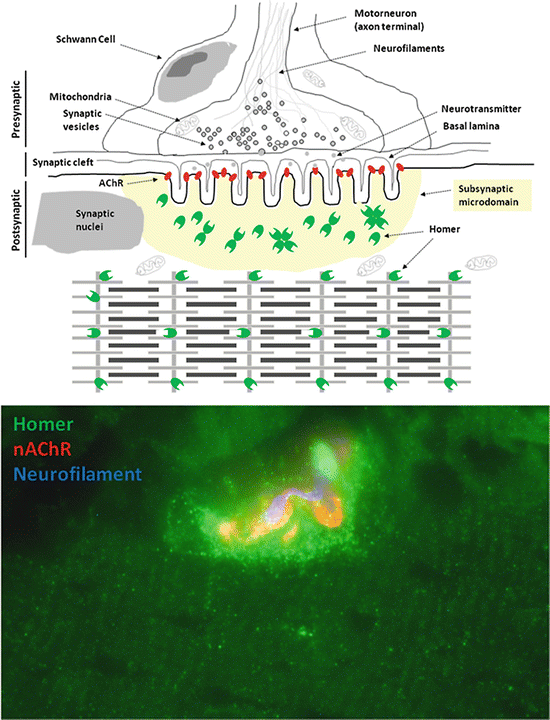
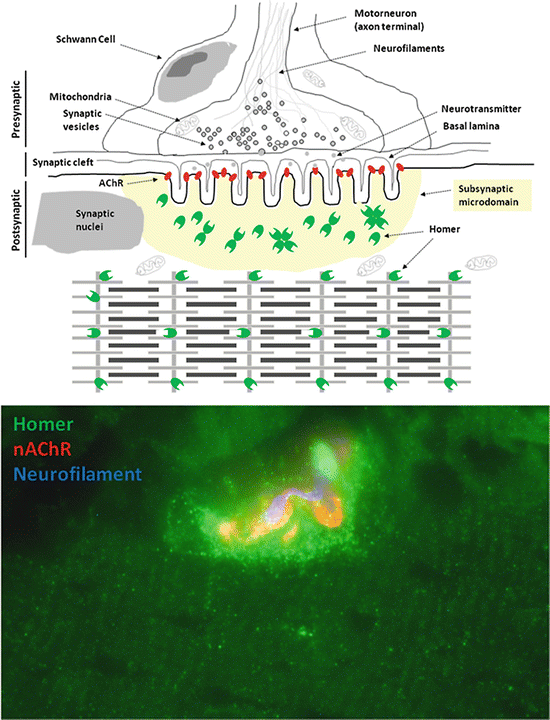
Fig. 3.2
Homer protein is concentrated at the NMJ. Upper panel, diagram representing Homer protein subcellular localization in human skeletal muscle. Homer proteins (green dots) are expressed both at the NMJ subsynaptic microdomain and at the Z-lines (costamere microdomain) of the contractile apparatus (Salanova et al. 2013). Lower panel, immunofluorescence image of human soleus myofiber with NMJ (red, nAChRs) immunostained with anti-Homer and anti-neurofilament (blue) antibodies
3.5.7 Role of Homers in Skeletal Muscle Atrophy
The signaling pathways responsible for muscle mass control/atrophy during prolonged disuse are not yet fully understood. Searching for new molecular players responsible for disuse-induced muscle atrophy, we recently proposed that proteins of the Homer family might play a pivotal role in neuromuscular synaptic signaling (Salanova et al. 2011). Based on our observation that Homers are particularly concentrated at the postsynaptic membrane of human and rodent neuromuscular junctions (NMJs), we suggested that they may play a role in the calcineurin-NFATc1 signaling pathway (Salanova et al. 2011).
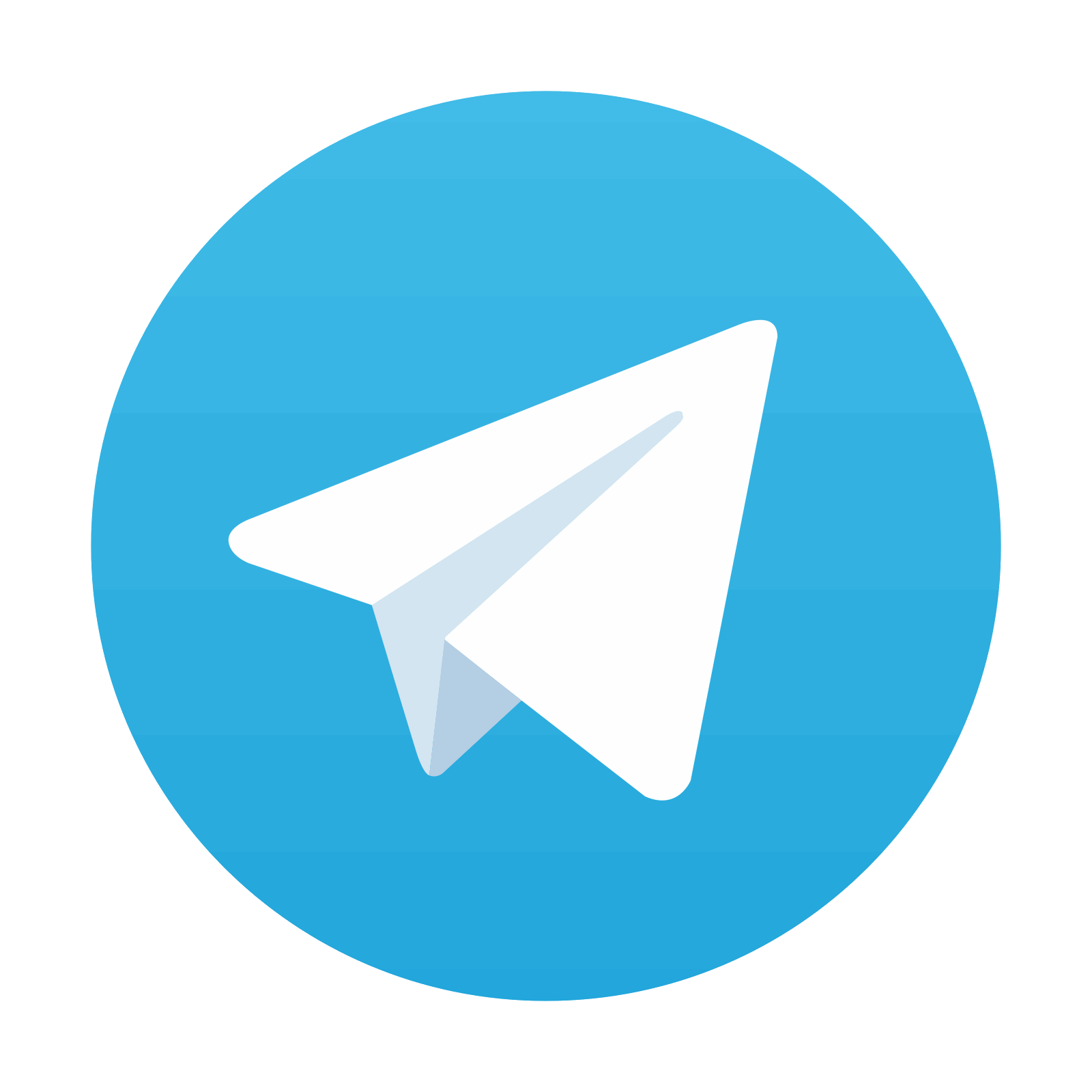
Stay updated, free articles. Join our Telegram channel

Full access? Get Clinical Tree
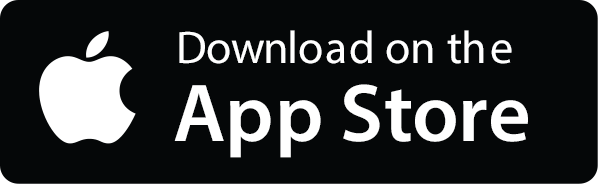
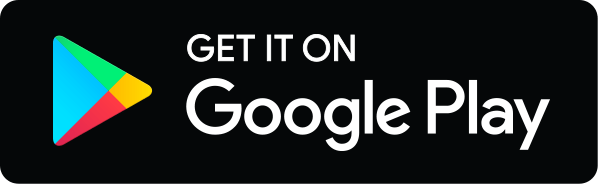