Fig. 2.1
Schematic representation of adult rodent neurogenesis. (a) Illustration of a sagittal section through the mouse brain showing location of neurogenic zones: the subgranular zone (SGZ) of the dentate gyrus (DG) in the hippocampus and the subventricular zone of the lateral ventricles (LV) where neurons are produced and migrate to the olfactory bulb (OB) via the rostral migratory stream (RMS). (b) Schematic summary of the neuronal differentiation cascade in the adult dentate gyrus. Each cell type is characterized by the expression of specific markers. (c) Representation of progenitor cell types and neurons in the SVZ/RMS/olfactory system based on the expression of localization and expression of specific markers. GFAP Glial Fibrillary acid protein, BLBP Brain lipid-binding protein, Tbr2 T-box brain protein 2, DCX Doublecortin, Prox1 prospero homeobox 1, NeuN Neuronal Nuclei, Dlx2 distal-less homeobox2, Mash1 mammalian achaete scute homolog 1, PSA-NCAM Poly-Sialated Neural Cell Adhesion Molecule, E ependymal cells
Type-2 Cells: Progenitors Cells
Type-1 cells divide asymmetrically and give rise to type-2 precursor cells, also known as transit amplifying precursors (TAP) or intermediate progenitor cells (IPC). Indeed, these cells possess an intense mitotic activity, thereby amplifying the pool of highly proliferating progenitors. Type-2 cells are morphologically distinct from type-1 cells: their processes are short and horizontally oriented. Type-2 cells are often subdivided in early progenitors, i.e. type-2a cells, and more mature type-2b cells. Type-2a cells still express markers of radial glia including nestin and Sox2 but no longer GFAP, a specific marker for type-1 cells. Type-2b cells show the first antigenic signs of neuronal differentiation and express several neuroblast markers such as doublecortin (DCX), a microtubule-associated protein involved in differentiation and neuronal migration, and the transcription factors NeuroD and Tbr2. The first synaptic inputs are GABAergic and recorded in type-2 cells, which suggest that these cells are wired to the pre-existing hippocampal network.
Type-3 Cells: Neuroblasts
Type-3 precursor cells correspond to a transition from a proliferative stage (type-2b cells) to postmitotic immature neurons. These cells are the most neuronally committed precursors and possess restricted proliferative capacity as compared to type-2b cells. Type-3 cells express markers of the neuronal lineage such as DCX, PSA-NCAM, NeuroD and Prox1 but lack the expression of glial markers. They exit the cell cycle and migrate on a short distance into the GCL, where they give rise to immature DCX neurons expressing calretinin, a calcium-binding protein, and neuronal nuclei (NeuN), a postmitotic neuronal marker. Most immature neurons produced will not survive and only few newborn granule cells will be stably integrated into the synaptic network of the DG.
Stem Cells or Not in the Dentate Gyrus?
Initially, hippocampal precursor cells were found to be multipotent in vitro and able to differentiate into electrophysiologically functional granule excitatory neurons. This persistent neuronal production in the adult DG arise from type-1 radial glial precursors (Doetsch et al. 1999), however whether these cells are able to self-renew by symmetric and/or asymmetric division remains under debate.
Indeed, it has been demonstrated, both in vitro and in vivo, that type-1 cells enter the cell cycle to generate, through asymmetric divisions, type-2 cells that in turn become neuroblasts, which then differentiate into mature neurons. Nevertheless, several teams were unsuccessful in identifying true stem cells in the SGZ. Seaberg and van der Kooy (2002) followed by Bull and Bartlett (2005) failed to isolate cells with self-renewal ability and multipotentiality in the adult DG (Bull and Bartlett 2005; Seaberg and Van Der Kooy 2002). More recently, Clarke et al. used in vitro clonal assay to challenge the stem cell properties (self-renewal and multipotentiality) of postnatal DG precursors. They found that type-1 cells are lineage-restricted progenitors with limited proliferative capacity and never detected colonies with abilities of self-renewing and multipotentiality (Clarke and Van Der Kooy 2011). Likewise, Encinas et al. showed in vivo that, after a limited number of asymmetric divisions, type-1 cells exit the cell cycle and start acquiring the astrocytic morphology, therefore leading to exhaustion of the “stem cell” pool (Encinas et al. 2011). However, among type-1 cells, some have a long-term ability to generate neurons and glia as well as to undergo self-renewing symmetric divisions, and therefore are true stem cells (Bonaguidi et al. 2011).
Adult Neurogenesis in the SVZ
The SVZ is composed of three main cell types: neuroblasts (Type-A cells), radial glia-like cells (Type-B cells), and TAPs (IPCs, Type-C cells) (Fig. 2.1). This heterogeneous population of cells exists in a dedicated environment, mainly composed of multiciliated ependymal cells (type-E cells) lining the ventricular surface as well as specialized vascular network (Ming and Song 2011). Arrangement of this “niche” is spatially defined, in that ependymal cells are organized in a pinwheel-like fashion surrounding monociliated type-B cells touching the ventricular surface (Mirzadeh et al. 2010). This «pinwheel structure» is only present in the ventricular wall of the neurogenic SVZ region suggesting an important role for intercellular interactions in neurogenesis (Mirzadeh et al. 2010). In addition, SVZ NSCs (i.e. type-B cells) extend basal processes that terminate on blood vessels that lie beneath the ependymal layer.
During lineage progression, astrocytic-like type-B stem cells produce TAPs that in turn give rise to type-A neuroblasts. These cells are immature proliferating neurons that migrate over long distances (several millimetres) from the SVZ bordering the lateral ventricles of the brain via the rostral migratory stream (RMS) toward the olfactory bulb (OB) through a tube formed by astrocytes. In the OB, type-A cells detach from the RMS and migrate radially toward glomeruli where they differentiate and becoming GABAergic granule or periglomerular interneurons.
Type-B Cells: Radial Glia-Like Stem Cells
Type-B cells extend an apical ending that contact the ventricle and a long basal process ending on blood vessels. The number of contacts in the ventricle appears to increase when SVZ proliferation is stimulated (Mirzadeh et al. 2010). Type-B cells are considered to be the NSCs with ultrastructural characteristics of astroglial cells such as irregular contours that profusely fill the spaces between neighbouring cells, a long basal process that terminates on blood vessels and a single primary cilium that reaches the ventricle lumen. The function of the single primary cilium remains to be understood. This cilium can have a mechanosensory function, suggesting that the force of CSF flow itself may exert an influence on the proliferative state of the stem cell (Singla and Reiter 2006). This organelle has also been implicated in Sonic Hedgehog (Shh), Wnt, and PDGF signaling pathways, which are important for the stemness maintenance (Ihrie and Alvarez-Buylla 2011).
Type-B cells express, like type-1 cells in the DG, GFAP, nestin and sox2 but, importantly, not S100B an astrocytic marker also expressed by ependymal cells (Ming and Song 2011). In the RMS, all type-B cells ensheath migrating neuroblasts. Type-B cells divide to generate by successive asymmetric or symmetric division TAPs (type-C cells).
Type-C Cells: Transient Amplifying Cells
Type-C cells represent the most important pool of proliferating progenitors within the SVZ, as type-2 cells in the DG. These cells are larger and more spherical than type-B cells and are intimately connected to blood vessels. Type-B cells form a tubular sheath surrounding type-C cells. Initial observations revealed that in vitro “neurospheres” were derived from type-B NSCs (Doetsch et al. 2002), however, a new study indicates that this neurosphere-forming ability is also an intrinsic property of type-C cells (Pastrana et al. 2009). In vivo, these cells still lack a specific clear-cut molecular marker that differentiate them from other cells (Doetsch et al. 1999). It was reported recently that type-C cells express specifically transcription factor Mash1 and a homeobox transcription factor, Distal less 2 (Dlx2), which is also express in type-A cells (Ming and Song 2011). Type-C cells will give rise to neuroblasts also named type-A cells.
Type-A Cells: Neuroblasts
The ultimate precursor type in the lineage progression of the adult SVZ is type-A cell. These cells express Dlx2 and are also characterized by the expression of proteins related to migration as DCX and PSA-NCAM (PolySialic Acid – Neuronal Cell Adhesion Molecule) – the embryonic form of NCAM. In the RMS, type-A cells form chains and migrate toward the OB through glial tube formed by astrocytes.
Six days after their birth in the SVZ, the first neuroblasts arrive in the OB and migrate radially into the layers where they differentiate into mature interneurons. A majority of neuroblasts differentiate into GABAergic granule neurons while a minority differentiate into GABAergic periglomerular neurons and a small portion into dopaminergic neurons. Finally, new granule cells with mature morphologies display spontaneous synaptic potentials (Carleton et al. 2003).
Cell Cycle
Introduction
The cell cycle consists in a series of sequential phases that takes place in a cell leading to its division and replication. The duration of the cell cycle varies from 2 to 3 h in unicellular organisms (such as Saccharomyces cerevisiae) to 24 h in eukaryotic cells. In prokaryotic cells, which lack a nucleus, the cell cycle occurs through binary fission: circular DNA is replicated, and then the cell splits into two identical cells which contain an exact copy of the original DNA. In eukaryotic cells, the cell cycle is an elaborate replication process divided in two periods: interphase and mitosis (M). Interphase leads to DNA duplication and comprises three distinct phases: G1 (Gap 1), S phase (DNA synthesis) and G2 (Gap 2). During G1, the expression of genes required for G1 progression and S phase entry results mostly from the initial Rb phosphorylation. After DNA replication that occurs in S phase, the cell enters in G2 where significant proteic biosynthesis occurs, mainly involving in the generation of microtubules necessary for mitosis. The process of mitosis comprises five phases: prophase, metaphase, anaphase, telophase and cytokinesis (a cytoplasmic division). Errors in mitosis often lead to cell cycle arrest and apoptotic cell death to avoid genomic instability and accumulation of mutations. Dependent of environmental and development signals, cells in G1 may stop their division and, temporarily or permanently, leave the cell cycle to enter in a quiescence or senescence state, i.e. G0 phase (Gap 0). During this stage, cells may remain quiescent for long periods of time (like skin fibroblasts or liver hepatic cells), possibly permanently (i.e. neurons or cardiac cells); or cells may enter in senescence in response to DNA damage or degradation.
The progress through the cell cycle requires the assembly of complexes of cyclin-dependent kinase (CDK, catalytic subunit) and a cyclin (regulator subunit) (Fig. 2.2). Functional heterodimer enters into the cell nucleus where it gets phosphorylated by a CDK-activating kinase (CAK) to activate or inhibit its target proteins and allows the entry into the next phase of the cell cycle. In mammals, they are at least 15 different cyclins known from Cyclin A to Cyclin Y, and 21 genes encoding CDKs among which a few (CDK1, CDK2, CDK4, CDK6) are exclusively associated with cell cycle regulation (Malumbres and Barbacid 2009). Each cyclin can bind one or two CDKs and each CDK can be associated with one or two cyclins. CDKs are constitutively expressed in cells whereas cyclins are synthesized in a cyclic manner in response to various molecular signals at specific cell cycle stages.
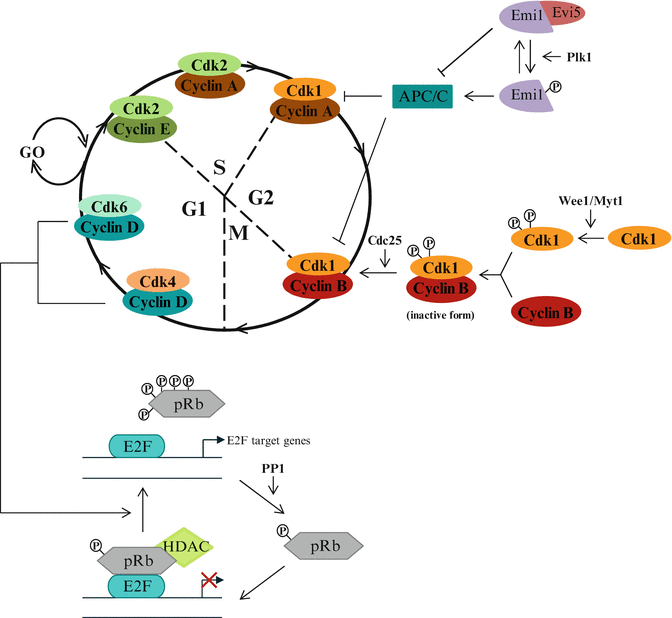
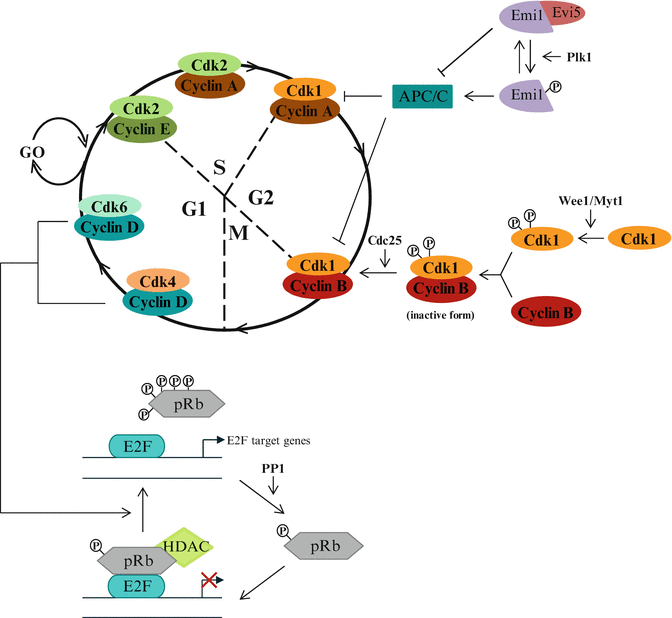
Fig. 2.2
Different aspects of cell cycle regulation. Modulation of cell cycle progression by CDK/cyclins, Rb/E2F pathway and M-phase promoting factor (MPF, i.e. CDK1/CyclinB) modulators
When quiescent cells (G0) enter into the cell cycle (in G1), the D-type cyclins (i.e. cyclin D1, D2 and D3) are expressed in response to mitogenic signals and bind to their catalytic partners, CDK4 or CDK6. The kinase activity of these complexes results in the expression of genes whose products are involved in G1/S transition and S phase progression. The G1/S transition requires the binding of CDK2 to cyclin E. CDK2 activity is also implicated in S phase progression through binding with cyclin A. Cyclin A is also important for G2/M transition through its association with CDK1. Finally, during M phase, cyclin B/CDK1 phosphorylates several important structural components of the cell that are responsible for nuclear envelope breakdown and reorganization of the microtubule and actin filaments.
Redundancy exists between cyclins: in the absence of cyclin E-Cdk2, cyclin A-Cdk2 can promote G1/S transition while cyclin D-CDK4/6 and cyclin E-CDK2 play redundant roles during G1/S progression. The degradation of cyclins A and B is regulated by the APC/C complex (Anaphase-Promoting Complex/Cyclosome) and the APC/C inhibitor Emi1 (Early Mitotic Inhibitor 1). The APC/C complex is an ubiquitin multisubunit E3 ligase, which contribute to the degradation of cyclins A and B by promoting poly-ubiquitinylation. Expression of these cyclins in S phase and G2 requires the inactivation of APC/C by Emi1 whose transcription is induced by E2F transcription factors. Emi1 is stabilized through binding to Evi5, while its degradation occurs after phosphorylation by Plk1 (Polo-like kinase 1).
CDKs Modulators
The activity of CDKs is regulated by both activating and inhibitory phosphorylations due to their association with cyclins, but also with “non-cyclin” CDK activators or CDK inhibitors.
CDK Inhibitors
The cell cycle is negatively regulated by two families of CDK inhibitors (CKIs): INK4 and CIP/KIP (Sherr and Roberts 1999). CKIs inhibit CAK phosphorylation of CDK by conformational changes, but without binding to CAK.
The INK4/ARF Family
The INK4/ARF (Inhibitor of Kinase 4/Alternative Reading Frame) family exclusively binds and inhibits CDK4 and CDK6 by blocking their binding to D-type cyclins during G1 phase progression. This family contains four members: INK4A (also named p16), INK4B (p15 or CDKN2B), INK4C (p18 or CDKN2C) and INK4D (p19 or CDKN2D). A unique gene encodes all INK4 proteins, which are 15- to 19-kDa polypeptides containing several ankyrin repeats implicated in their interaction with CDK4 and CDK6. These proteins show poor sequence homology (approximately 40 %), however they are highly conserved among species (for example, each of the INK4 proteins in the mouse shares 90 % identity with the corresponding proteins in human). INK4 proteins are equally potent as inhibitors, but are differentially expressed during mouse development and show diversity in their pattern of expression suggesting cell-lineage specificity or tissue-specific functions.
The CIP/KIP Family
Members of CIP/KIP (CDK Interacting Protein/Kinase Inhibitory Protein) family are negative regulators of the cell cycle progression. The CIP/KIP family is composed of three members: p21Cip1 (CDKN1A), p27Kip1 (p27, CDKN1B) and p57Kip2 (CDKN1C), which are negative regulators of cyclin E/CDK2, cyclin A/CDK2 and cyclin B/CDK1, and negative or positive regulators of cyclin D/CDK4-6 (Besson et al. 2008). Contrary to the INK4/ARF family, the action of Cip/Kip members depends on their binding to both cyclins and Cdks subunits, that occurs on a specific N-terminal domain conserved in all CIP/KIP members, the “LFG” domain. The remainder of their sequence is poorly conserved, suggesting that each of these proteins present specific functions in the regulation of the cell cycle. Under physiological conditions, p27 is highly expressed during G0 and early G1 phase, and then rapidly decrease in late G1 and S phase. p27 blocks S phase progression through inhibition of cyclin E/CDK2 and cyclin A/CDK2. p21 is less specific, it inhibits the activity of cyclin E-A/CDK2 and cyclin B/CDK1 complexes, and also cyclin D/CDK4 that controls G1 checkpoint. Moreover, p21 can bind PCNA (Proliferating Cell Nuclear Antigen) subunit of δ DNA polymerase and inhibits this enzyme activity to block DNA replication. p57 seems more implicated in the regulation of the cell cycle during the embryonic development, and it present a tissue-specificity expression.
Cip/Kip proteins could also act as non-inhibitory or positive modulators of cell cycle progression by facilitating the transition between the G1 and the S phase. Their progressive binding to increasing amount of cyclin D/CDK4 dimers releases their inhibitory activity on cyclin E/CDK2 and thus favors cell cycle progression. The balance between inhibitory and non-inhibitory functions of CIP/KIP could be linked to their phosphorylation state within specific domains. For example, p27 is an inhibitor of cyclin D/Cdk4 complexes in cell cycle-arrested cells, while it is associated with active cyclin D/Cdk4 complexes in proliferating cells. Indeed, p27 is preferentially tyrosine phosphorylated (Tyr88 and 89) in proliferating cells, causing it to bind cyclin D/Cdk4 in a non-inhibitory mode. In quiescent cells, p27 is dephosphorylated while bound to cyclin D/Cdk4 and therefore inhibits complexes activity (James et al. 2008). It is also possible that CIP/KIP role as inhibitor or non-inhibitor could depend directly on their expression level (Labaer et al. 1997).
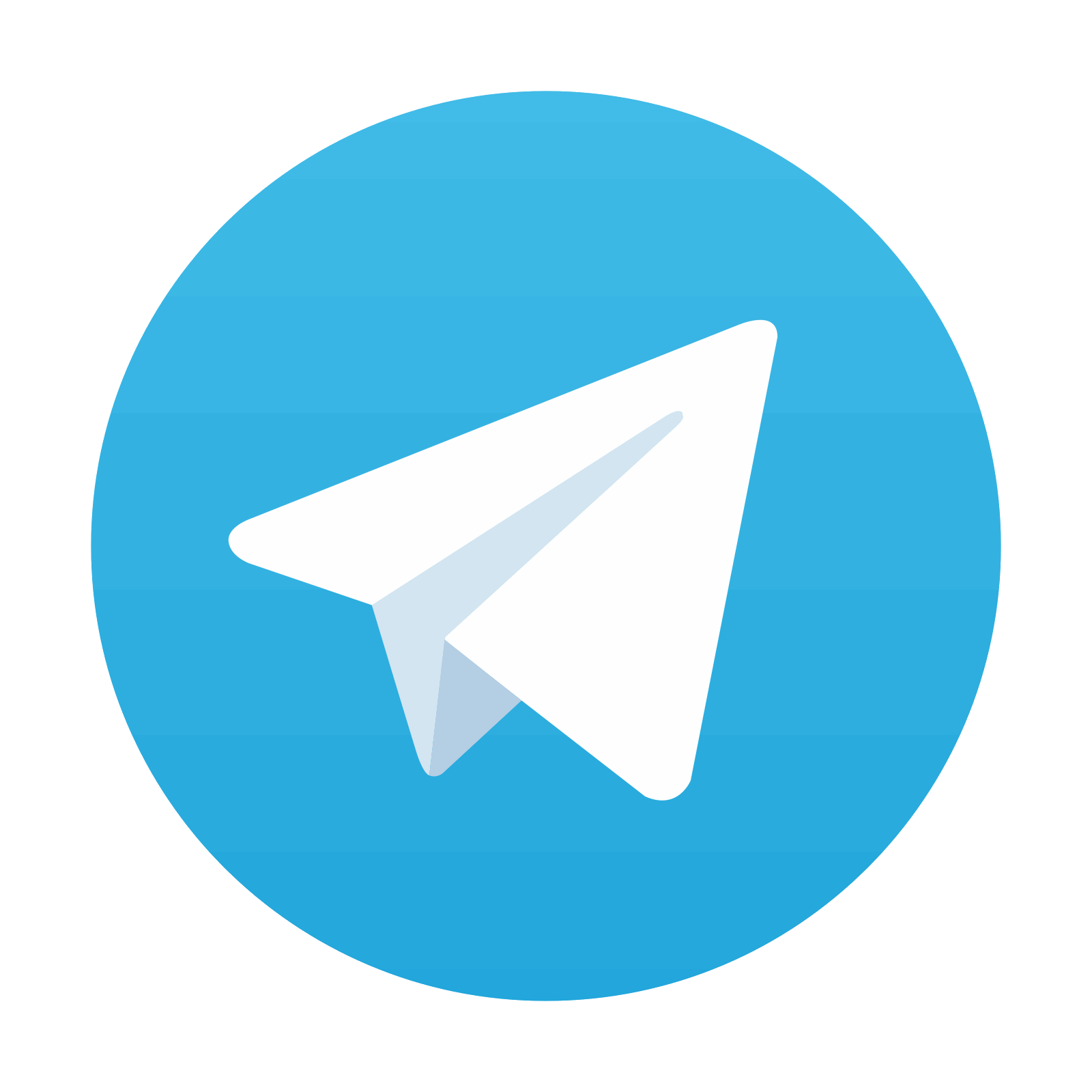
Stay updated, free articles. Join our Telegram channel

Full access? Get Clinical Tree
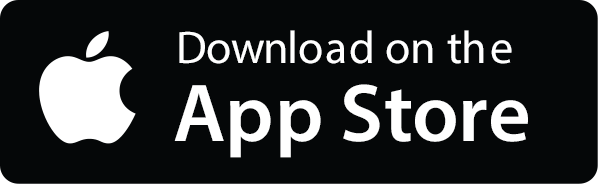
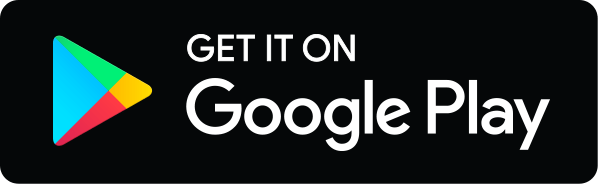