Electroencephalography (EEG) records the electrical activity generated by the brain. It is a safe, readily available, and inexpensive tool to provide real-time information on brain functioning. It can provide valuable clinical information on patients with focal brain lesions, coma, and encephalopathy. It is an essential test for the evaluation and management of patients with epilepsy. It may provide definitive diagnostic information on patients with seizure-like episodes or alteration of the level of consciousness that is psychogenic in nature.
Prolonged EEG monitoring is a valuable tool in the hospital setting. Video-EEG monitoring is used for the characterization of spells of indeterminate mechanism as to whether epileptic or nonepileptic, for seizure-type classification, for the localization of a seizure focus in the evaluation of candidates for epilepsy surgery, and for characterization of the interictal epileptiform discharges. Prolonged EEG monitoring in the ICU setting is able to recognize subtle or electrographic seizures and is an essential tool in the management of status epilepticus.
Electroencephalography EEG records the electrical activity of the brain, measuring the spatial distribution of voltage fields on the scalp and their variation over time. The activity detected is considered the result of the sum of excitatory and inhibitory postsynaptic potentials generated primarily in the apical dendrites of the pyramidal cells in the outer layers of the cerebral cortex (mainly layers III, IV, and VI). The cortical activity is modulated by subcortical structures such as the thalamus and other brainstem centers, mainly through the ascending projections of the reticular activating system. The cortical activity is synchronized by these subcortical pacemakers in either physiologic (ie, sleep) or pathologic (ie, generalized spike-wave discharges) states. EEG, by providing continuous, real-time information on the fluctuations of brain electrical activity, is the only readily available, practical, and inexpensive ancillary test to assess brain function in the hospital setting.
The EEG activity is recorded by a set of 21 electrodes placed on the scalp in accordance to the International 10–20 system. These electrodes are distributed strategically to cover all areas of the cortex accessible from the scalp. The EEG hardware consists of multiple differential amplifiers (channels) that record differences in potential between two electrodes. The information from these multiple channels is arranged in a specific order called a montage, providing information on the topography of voltage differences. Multiple montages allow for a systematic visualization of the field of electrical activity of the brain. The EEG is graphically displayed in multiple channels (typically 21–24), each carrying information from different locations of the scalp overlying the cerebral cortex. This activity is analyzed in terms of its frequency, voltage, morphology, and topography.
It is important to recognize that the scalp EEG has significant limitations. Large areas of the cortex, such as the interhemispheric or basal regions, are located far from the surface and beyond the detection of scalp electrodes. Subcortical lesions, unless very large or diffuse, may go undetected on EEG. Furthermore, because of the high impedance of the skull, the minimal volume of brain required to generate a signal change on the scalp has been estimated at 6–10 cm2. Very restricted cortical lesions, either lesional or irritative, and a high proportion of simple partial seizures may have no EEG expression during an ictal scalp recording.
The EEG is sensitive to detect and localize brain dysfunction. The electrophysiological changes observed on EEG are usually nonspecific and do not allow to differentiate between a variety of pathological processes. In pathologies resulting in loss of brain function, the EEG usually demonstrates slowing of the brain waves, either focal or diffuse. For example, in a patient with an acute ischemic middle cerebral artery infarct the EEG typically would show a focal area of slowing in the central region of that hemisphere. This focal slowing is evident immediately after the onset of the ischemia and may precede the visualization of the structural changes on a brain CT by several hours. EEG can also detect focal functional deficits, like the transient slowing observed in the region of a seizure focus following a prolonged seizure. Neuroimaging studies are usually negative in this situation. In toxic or metabolic encephalopathies the EEG shows diffuse slowing of the background, proportional to the severity of the condition. In disorders resulting from a paroxysmal, excessive, disorganized discharging of neuronal networks, such as the epilepsies, the EEG is the test of choice in demonstrating the underlying state of neuronal hyperexcitability, in both the ictal and the interictal state.
Focal slowing on an EEG usually points to the presence of an underlying structural or functional lesion. Structural lesions that affect the subcortical white matter usually result in a polymorphic irregular slowing that can be continuous or intermittent. The EEG, however, is unable to provide specific information about the nature of the lesion. Lesions such as a cerebral infarct, a neoplasm, or an abscess have a similar appearance on EEG. Neuroimaging procedures are much more accurate to localize and provide information about the nature of the lesion.
Lateralized periodic discharges (LPDs), previously known as periodic lateralized epileptiform discharges (PLEDs), are often seen with acute or subacute focal brain insults (Figure 9-1).1 An acute large hemispheric stroke is the most common cause. LPDs are also commonly associated with herpes simplex encephalitis and may provide an early clue to the diagnosis. LPDs usually resolve in a matter of a few days and they are not necessarily associated with seizures. However, patients with LPDs, especially if the pattern known as “LPDs plus” is present, are at a higher risk of acute seizures and focal epilepsy. LPDs can also be seen in focal status epilepticus. Therefore, the significance of LPDs on an EEG needs to be carefully analyzed in the clinical context.
Figure 9-1
EEG in a 63-year-old man showing lateralized periodic discharges (LPDs) following an acute cerebral infarct in the right hemisphere. These high-voltage sharp waves occur with a periodicity of about 1 second (arrows) throughout the entire EEG tracing. They usually indicate an acute underlying focal brain insult and tend to resolve over a few days.

The characteristic finding on EEG in an acute encephalopathy is diffuse slowing. This finding is nonspecific, and different causes of encephalopathy result in very similar changes. The EEG can also assess the severity of the encephalopathy. A mild encephalopathy results in a slowing of the dominant occipital rhythm (alpha rhythm) and an increase in the amount of slower frequencies (theta activity) diffusely. In a moderate encephalopathy, the alpha rhythm disappears, the diffuse slowing is more severe (delta activity), and the typical sleep transients (K complexes and sleep spindles) are less well defined. Runs of frontal intermittent rhythmic delta activity (FIRDA) can be seen as well as brief periods of diffuse attenuation during sleep. As the encephalopathy worsens, there is loss of the sleep/wake differentiation, loss of reactivity to noxious stimulation, a burst-suppression pattern, and finally a complete cessation of electrocerebral activity. When assessing EEG findings in patients with encephalopathy, it should be remembered that barbiturates, benzodiazepines, and general anesthetics at high doses can induce all these changes in a normal brain.
In coma, the EEG may quantitate the degree of electrophysiological dysfunction, provide localizing information, and assist in the clinical evolution or response to therapy.2 Certain EEG patterns in coma have prognostic significance. A burst-suppression pattern following cardiac arrest is usually associated with a poor outcome (Figure 9-2). The patterns known as “alpha coma” and “spindle coma” are also most commonly associated with hypoxic brain injury and carry a poor prognosis. However, if these patterns are induced by medication overdose or trauma, the outcome tends to be more favorable.
Figure 9-2
Burst-suppression pattern on EEG hours after cardiac arrest. EEG shows brief bursts of diffuse, rhythmic, high-voltage slow waves with intermixed spikes alternating with periods of profound suppression of the EEG activity. This periodic pattern was persistent throughout the entire recording. The patient was profoundly comatose and experienced generalized myoclonic jerks coinciding with the bursts of EEG activity. These findings are consistent with postanoxic myoclonic status and carry a very grave prognosis.

EEG can be used as ancillary procedure in the determination of brain death. Absence of electrical activity can be established as long as a detailed protocol is carefully followed.3 It should be remembered that absence of EEG activity may be seen in patients with some preservation of brainstem function. Therefore, the diagnosis of brain death is always a clinical one and should not be based solely on EEG information.
Periodic patterns consist of EEG transients that recur with a certain periodicity, and they can be generalized (GPDs) or lateralized (LPDs). Triphasic waves, a subtype of GPDs, are characteristic of toxic metabolic encephalopathies, especially hepatic encephalopathy, but can also be associated with seizures and nonconvulsive status epilepticus. GPDs with a periodicity of about 1 Hz are characteristic of Creutzfeldt-Jacob disease, albeit a late finding in the course of the disease. GPDs can also be seen in hypoxic–ischemic encephalopathy, toxic encephalopathies (ie, lithium, cefepime, baclofen), or status epilepticus.
EEG is an essential test in the diagnosis and management of patients with epilepsy. Interictal epileptiform discharges, consisting of spikes, sharp waves, often followed by slow waves, provide evidence of abnormal cortical excitability when the patient is in the asymptomatic interictal state.4 Detecting an interictal spike provides important information as to the diagnosis of the type of epilepsy (focal versus generalized) and the possible location of the seizure focus, and guides therapy (Figures 9-3 and 9-4). The recording of a seizure during EEG is usually not necessary in the management of the vast majority of patients with epilepsy. The ictal EEG provides even stronger evidence for the diagnosis of epilepsy and aids in classifying the seizure type and localization of the seizure source.5
Figure 9-3
EEG shows a burst of generalized spike-wave activity at 3.5 Hz with a duration of 6 seconds in a 9-year-old boy with childhood absence epilepsy. The discharge occurred after about 60 seconds of forced hyperventilation. Forced hyperventilation is very effective in triggering absence seizures and can also be used as a bed-side maneuver.

Figure 9-4
EEG during sleep demonstrating a left temporal sharp wave (arrow) in a 32-year-old woman with medically intractable complex partial seizures. The sharp wave is noted in the left temporal chain (channels 9–12). Note the absence of the discharge in the homologous channels (13–16) in the right temporal region. MRI demonstrated left hippocampal atrophy (mesial temporal sclerosis). The patient became seizure free following a selective left amygdalo-hippocampectomy.

The routine EEG has some limitations in the evaluation of seizures and epilepsy. Interictal epileptiform discharges are present on a small percentage of the general population without clinical seizures. Many of these abnormalities are considered genetic traits, without the phenotypic expression of seizures. Patients with epilepsy, not uncommonly, may have a normal interictal EEG. Repeated recordings or prolonged EEG monitoring may be required in some cases to confirm the diagnosis. It is important to recognize that the diagnosis of epilepsy is a clinical one and never based solely on EEG findings.
In a patient admitted to the hospital after a single new-onset seizure, who has regained baseline neurological status, a STAT EEG is rarely necessary. In the majority of these cases the EEG can be done electively in the outpatient setting. Reasons to obtain a STAT EEG in the acute setting include a pattern of recurrent seizures consistent with status epilepticus, a failure of the patient to recover consciousness after a seizure, or if an induced coma to control seizures is being implemented. Prolonged EEG monitoring is recommended in these situations.
Occasionally patients may present in the ED with a prolonged confusional or twilight state, unusual behavior, or even psychosis due to nonconvulsive status epilepticus. The spectrum of clinical presentation ranges from subtle changes in behavior to stupor or coma. NCSE may present de novo, more commonly in the elderly, or occur in patients with absence epilepsy or focal epilepsy, especially temporal lobe epilepsy. A STAT EEG is the best way to confirm the diagnosis, establish the underlying seizure type, and select the proper therapy.6
Seizures are very common in the ICU setting. Patients with a variety of encephalopathies can present acute reactive seizures. Patients with acute or chronic brain insults are at an even higher risk of seizures. Overt convulsive seizures are rarely missed by the ICU staff. Seizures, however, can be very subtle or even subclinical in patients with altered level of consciousness. Subtle clinical manifestations of seizures include tonic eye deviation, nystagmus, clonic twitching of an extremity, or autonomic changes. With the more widespread use of prolonged EEG monitoring, it has become evident that the majority of seizures in the ICU setting are nonconvulsive in nature and, therefore, likely to be missed without an EEG (Figure 9-5). In patients with traumatic brain injury, intracerebral hemorrhage (ICH) and subarachnoid hemorrhage (SAH), EEG monitoring has found a prevalence of seizures of 15–40%.
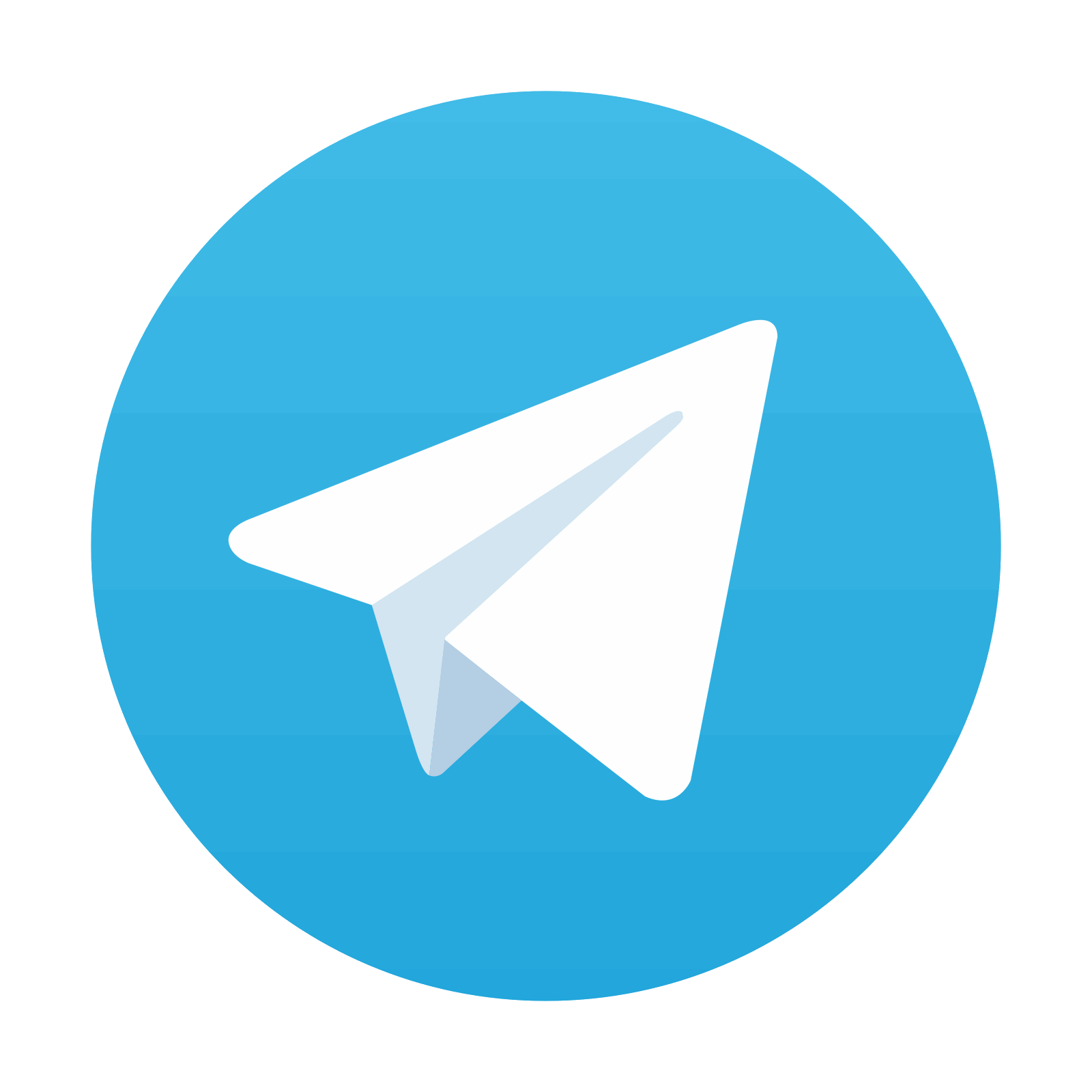
Stay updated, free articles. Join our Telegram channel

Full access? Get Clinical Tree
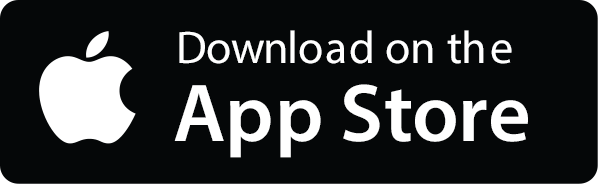
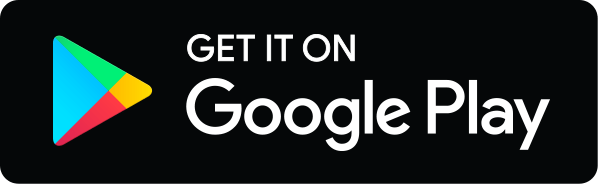