INTRODUCTION
The few individuals who reach very old age are called ‘longevity outliers’. This particular group represents the fastest growing age group worldwide. For instance, the number of centenarians increases steadily in the US population and their numbers are expected to grow to more than 400 000 in the next 30 years1.
Awareness of age-related diseases such as dementia has prompted considerable interest in the study of the ageing human brain to trace a clear boundary between physiological and pathological ageing, to carry out primary prevention on populations at high risk of developing dementia, to achieve secondary prevention and to apply eventual disease-modifying therapy to at-risk subjects. Results from these types of studies could be used to plan political and public health interventions. Recent investigations have prompted advancement in biochemical, structural, functional and neurophysiological fields.
Ageing is a physiological dynamic process due to a specific property of the central nervous system (CNS) known as neuronal plasticity. The concept of ‘plasticity’ applied to neurobiology typically denotes the potential for change in addition to the mechanisms of self-repair and/or of reorganization of neural connections. Neural plasticity underlies learning as well as endogenous brain function repair2, including synaptic efficacy and synaptic redundancy; on this basis the functional status and distribution of a network depends on the balance between neural excitation and inhibition, with some areas appearing silent or ‘masked’ by a mechanism of active tonic inhibition, the latter being prone to alteration or removal, causing a rapid change of the functional network, a process termed ‘unmasking’3. Additional mechanisms include long-term potentiation (LTP) and long-term depression (LTD), which contribute to the functional strengthening or weakening of existing synapses by which information is stored in the CNS. LTP has been regarded as the prototypic mechanism of modified synaptic efficacy, and it can differently occur via modification of the input to a postsynaptic cell, the concomitant synchronous input to the synapse from another cell, or postsynaptic depolarization. LTD stems from Na+ or Ca2+ channels at least for short-term changes, while LTP-like mechanisms include glutamate-dependent NMDA receptor activation for long-term changes, a process that can be antagonized by GABA inhibition4. Another plasticity-related process is neuronal sprouting and formation of new synapses influenced by local neurotransmitter, neurotrophic factors and synaptic protein synthesis5. In particular, dendrites and related spines with synaptic connections undergo continuous remodelling most likely also modulated by interaction with neighbouring astrocytes4.
Postmortem examinations show that brain ageing is characterized by the formation of neurofibrillary tangles and senile plaques in both cognitively intact individuals and patients with dementia, namely Alzheimer’s disease1 . The ubiquitous presence of these lesions and the steady increase of the prevalence of dementia up to 85 years have strongly supported a continuum between normal and pathological brain ageing.
Ageing is a physiological process that occurs asynchronously in different areas of the brain, its rate being also dependent on the style of life. The differential age-related changes produced in several regions of the brain in the anatomy of neurons, volume tissue, density and dynamics of several neurotransmitters give support to this suggestion6.
From a macroscopic point of view, brain ageing becomes evident by atrophy. In 1955 Brody estimated that the neuronal loss was about 40%. In 1986 Braak and Braak specified that during the ageing, especially pyramidal cells of frontal lobes die. Some studies suggest that the primum movens is a functional and then a structural modification of synapses7. It is followed by a modification of the dendritic tree and then by the neuronal death, today known as aposklesis. Particularly, the hippocampus seems to be one of the most vulnerable structures in brain ageing.
Functional modifications precede the structural ones, so it is hoped that by integrating structural techniques with the functional ones it will be possible to better investigate brain ageing. Moreover, modern neurophysiological techniques (transcranial magnetic stimulation, TMS; electroencephalography, EEG; magnetoencephalography, MEG; event-related potentials, EPRs) could be helpful for this purpose, being of low cost and non-invasive8. Modern techniques of functional neurophysiological imaging allow investigation with high accuracy of the cortico-cortical ‘connectivity’ that is the base for synchronization and functional binding. These phenomena allow neuronal populations, even at a distance, to contribute to a specific task or to process a specific input working together only for a few milliseconds. Analysis of EEG and MEG signals and TMS techniques gives us worthwhile information about that.
TRANSCRANIAL MAGNETIC STIMULATION
TMS is a brief and intense magnetic field, created by a strong electric current circulating within a coil resting on the scalp; it penetrates human tissue painlessly and, if the current amplitude, duration and direction are appropriate, it induces in the brain (or in spinal roots, or in nerves) electric currents that can depolarize neurons or their axons. TMS can demonstrate causal relationships between the stimulated area and behaviour. It can be used to interfere with the neuronal activity underlying sensory processing, motor planning or execution, attention and visual processing or awareness, different forms of declarative and non-declarative memory, reasoning, mathematics, language and consciousness. This temporary disruptive effect of TMS on cortical function is often referred to as a ‘virtual lesion’ and is likely to occur because the stimulus transiently synchronizes the activity of a large proportion of neurons under the coil, thus inducing a long-lasting, generalized inhibitory postsynaptic potential that reduces cortical activity for the next 50 to 200 milliseconds, depending on stimulus intensity and duration. Moreover, repetitive TMS (rTMS) can produce changes – outlasting the period of stimulation – in cortical excitability9.
rTMS studies are increasing knowledge about correlation between anatomic structures and their functions. For example, they showed that dorsolateral prefrontal cortex (DLPCF) is implicated in working memory and in verbal episodic memory tasks.
Lesions of frontal lobes cause a reduction of learning and memory abilities but they do not cause the same memory loss as bilateral hippocampal lesions do. So, it has been demonstrated that prefrontal cortex (PFC) is involved in episodic memory tasks and that during encoding or retrieval, there are different areas of PFC that are activated with different patterns. It has been demonstrated that left PFC is involved in encoding processes; instead right PFC is involved in retrieval processes. This theory is known as hemispherical encoding retrieval asymmetry (HERA)9-11. Nowadays, it is known that the HERA model is not so absolute but that there could be variations due to the nature of the presented stimuli, the strategies of memorization used and the grade of difficulty of the task. Recently, positron emission tomography (PET) and functional magnetic resonance imaging (fMRI) studies have demonstrated that PFC activity tends to be less asymmetric in older than in younger adults (hemispheric asymmetry reduction in old adults, HAROLD). This change may help counteract age-related neurocognitive decline (compensation hypothesis) or it may reflect an age-related difficulty in recruiting specialized neural mechanisms (dedifferentiation hypothesis)12,13. Functional neuroimaging techniques could not be used to study whether the loss of asymmetry during ageing reflects compensatory mechanisms or dedifferentiation processes; in fact, they show only the activation of a specific area during a task, but this activation could be related to the performance of the task without being the cause. Instead, this issue was addressed by using rTMS to assess causal relationships between performance and stimulated brain regions. Rossi et al. studied the effects of rTMS applied to the left or right DLPFC simultaneously with the presentation of memoranda on visuo-spatial recognition memory task, in a population of healthy subjects divided into two classes by age (<45 and >50 years). In the younger subjects, rTMS of the right DLPFC interfered with retrieval more than left DLPFC stimulation. The asymmetry of the effect progressively vanished with ageing, as indicated by bilateral interference effects on recognition performance. Conversely, the predominance of left DLPFC effect during encoding was not abolished in the older subjects, thereby indicating its causal role for encoding along the life span. Findings with rTMS suggest that the bilateral engagement of the DLPFC has a compensatory role on episodic memory performance across the age span13.
Physiological ageing is associated with more complex activations of the motor system to compensate for the reduced skill in motor performance14,15. Therefore, TMS variables can provide useful clues to investigate causally subclinical changes of cortical excitability at the motor cortex level. Unfortunately, studies do not show converging findings, probably as a result of technical and experimental differences among them13,16-18. Apart from assessing cortical excitability, TMS can be used to measure intracortical facilitatory and inhibitory mechanisms resulting from the balance among neurotransmitters, and dynamic synaptic adaptations to progressive neuronal loss. Motor threshold is generally reduced in Alzheimer’s disease compared to healthy age-matched controls and is similarly reduced in subcortical ischaemic vascular dementia16,19,20, even if several reports have not found reduction of motor thresholds21,22, with one study noting increased rather than decreased motor thresholds in Alzheimer’s disease23. Global cortical hyperexcitability while the brain is ‘at rest’ in Alzheimer’s disease is a consistent result22, which seems to occur independently of GABAergic and cholinergic cortico-cortical mechanism dysfunction as reduced motor thresholds and intracortical inhibition (ICI) and short-latency afferent inhibition (SAI) are uncorrelated. Since TMS does not provide specific pathophysiological information but is sensitive to the ‘global weight’ of several neurotransmitters as well as subcortical and cortical motor inputs, interpretation of cortical hyperexcitability in Alzheimer’s disease is not yet possible.
The majority of these TMS results indicate that the cortex of Alzheimer’s disease patients is hyperexcitable, and that such hyperexcitability may offer clues for the differential diagnosis from other dementias in which the cholinergic deficit is not predominant. An intriguing issue that can be addressed by TMS is the study of cortical reorganization of the motor output in Alzheimer’s disease. Indeed, motor cortex physiological organization is early involved in Alzheimer’s disease, despite the lack of clinically evident motor deficits, which only appear later24. This observation is not surprising considering that the motor cortex, through muscarinic receptors and cholinergic terminals, receives a major input from the nucleus basalis of Meynert25. Mapping of motor output in mild Alzheimer’s disease patients asymptomatic for motor deficits has demonstrated frontal and medial shift of the cortical motor maps for hand muscles. In healthy controls the centre of gravity of motor cortical output is coincident with the site of maximal excitability (hot spot26), whereas in Alzheimer’s disease patients it shifts towards frontal and medial regions in the absence of changes in the hot spot22. An altered synaptic plasticity in Alzheimer’s disease also has been demonstrated by applying brief trains of high-frequency rTMS to motor cortex and recording motor-evoked potentials (MEPs) from the contralateral hand muscles. This procedure can induce a progressive increase in MEP size in normal age-matched controls, but it produces opposite changes in Alzheimer’s disease. This finding was interpreted as an altered short-term synaptic enhancement in excitatory circuits of the motor cortex. Additional assessment of these findings will be required by evaluating Alzheimer’s disease patients after different pharmacological treatments (e.g. cholinergic versus drugs acting on NMDA glutamate receptors).
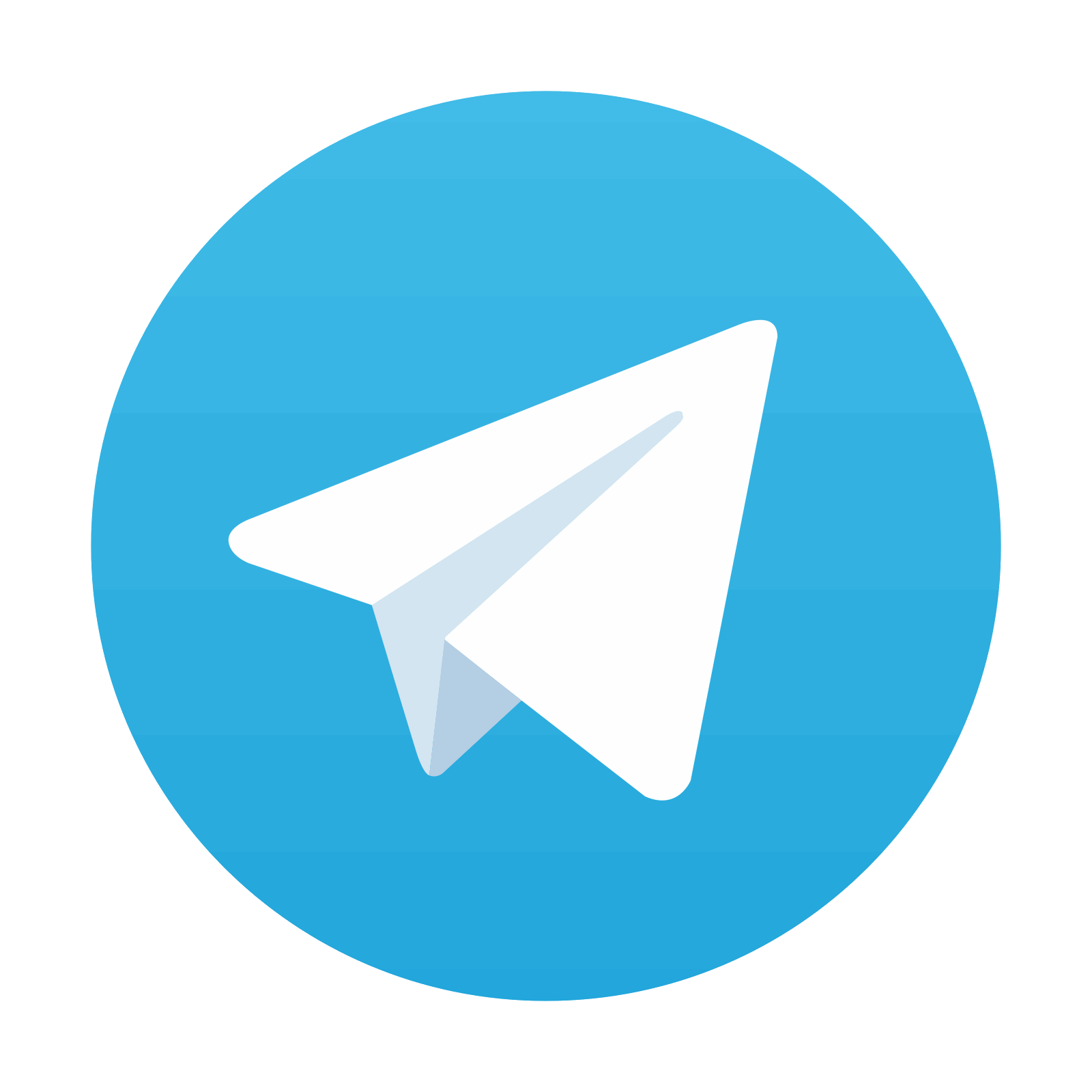
Stay updated, free articles. Join our Telegram channel

Full access? Get Clinical Tree
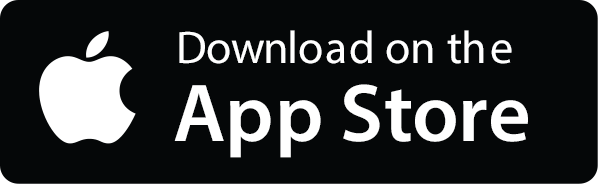
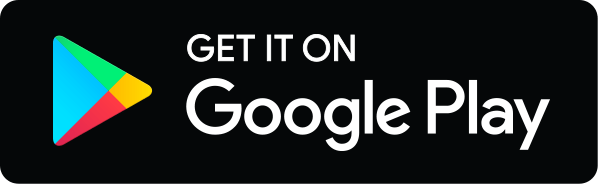