Neuroradiological Imaging in Children
Ken Allison
Todd Miller
Jacqueline Bello
Neuroradiological imaging plays a fundamental role in the diagnosis and management of neurological disease in children and adolescents. This chapter addresses the commonly available neuroimaging modalities and provides recommendations on how best to employ them in the evaluation of children, with particular attention to clinical conditions that the child and adolescent psychiatrist might encounter. In addition, it presents the neuroimaging characteristics of these conditions.
Neuroimaging Techniques
COMPUTED TOMOGRAPHY
Head computed tomography
The most readily available test for brain imaging is often computed tomography (CT). This modality is a quick and efficient screening tool for demonstrating intracranial structures and mass effect. Noncontrast head CT without sedation is usually tolerated by children as well as adolescents. It provides sufficient tissue contrast to demonstrate hydrocephalus, most tumors, infarcts, hemorrhage, and many infections. Compared to magnetic resonance imaging (MRI), CT provides better bony detail and spatial resolution. These attributes, most useful in an emergent situation, come at a cost. CT scan images are generated by passing x-ray beams through the patient. Although recent data suggest the radiation dose associated with head CT is not insignificant, this radiation exposure may be more significant in children than in adults. In addition, modern scanner designs, for the same test, deliver higher doses to a smaller patient than to a larger one (1).
Computed tomography angiography
Computed tomography angiography (CTA) is a head CT acquired rapidly (2 seconds) during an intravenous bolus injection of iodinated contrast timed to optimize arterial opacification. In order to maximize evaluation of the vascular structures, which are measured in millimeters, these scans are acquired with the thinnest slice width provided by scanner technology (as little as 0.625 mm). These factors combine to create one of the highest dose CT scans performed. While the radiation exposure is of some concern, CTA provides an unparalleled noninvasive three-dimensional demonstration of the intracranial vascular tree and
its relationship to the surrounding anatomy (Fig. 2.1). Children usually require conscious sedation because the contrast injection consists of a rapid bolus that is difficult to tolerate.
its relationship to the surrounding anatomy (Fig. 2.1). Children usually require conscious sedation because the contrast injection consists of a rapid bolus that is difficult to tolerate.
MAGNETIC RESONANCE IMAGING
Magnetic resonance imaging is the current gold standard for demonstrating neural tissue in vivo. The superior soft tissue contrast resolution of MRI allows for detailed characterization of the gray and white matter, which is the most necessary component in detecting brain abnormalities. Spatial resolution of MRI is slightly less than that which is available with CT scan. The magnetic field required to produce MR images precludes the use of MRI for patients with certain metallic surgical implants as well as implanted electronic devices (e.g., cochlear implants, pacemakers, and defibrillators). Imaging takes place inside a cylinder 3 feet in diameter and several feet in length in configured magnets. More “open” magnet designs may reduce some of the claustrophobic problems associated with “closed” bore magnets. Patient motion significantly degrades image quality. Excellent tissue visualization and no ionizing radiation make MRI the imaging test of choice in the pediatric population. Because MRI is useful in staging hemorrhage of different ages, it is often applicable to investigation of suspected nonaccidental trauma. There are only scarce nonemergent clinical scenarios where CT represents the neuroimaging modality of choice.
Generic MRI has several adjuncts, including magnetic resonance angiography (MRA), cerebrospinal fluid (CSF) flow studies, diffusion weighted imaging (DWI), diffusion tensor imaging (DTI), perfusion, functional MRI, and spectroscopy.
Magnetic resonance angiography is a specific imaging sequence designed to emphasize fluid flow within vessels. Thin-section axial or coronal images are acquired (without contrast) and are then reconstructed in three dimensions. These images provide near millimeter resolution and demonstrate most vascular abnormalities.
Cerebrospinal flow studies are one of the few MRI examinations tailored to maximize temporal resolution over spatial resolution or tissue contrast. In general, several sagittal images are rapidly acquired over the same midline space to produce a cine effect (i.e., a “mini-movie”). The flow of CSF through the ventricles and cisterns is emphasized by employing a phase-contrast pulse sequence. This problem-solving tool is frequently used to demonstrate the physiologic significance of low cerebellar tonsils in symptomatic patients or confirm flow diversion in patients with third ventriculostomies for hydrocephalus.
Diffusion weighted imaging measures molecular motion of extracellular water molecules and is particularly useful in the setting of acute ischemia. Cytotoxic edema is reflected as restricted diffusion. DWI is also useful in defining the diffusion characteristics of fluid collections (e.g., arachnoid cysts and epidermoid inclusion cysts) (Fig. 2.2).
SPECIALIZED TECHNIQUES
Perfusion imaging
Perfusion imaging involves dynamic imaging of the brain with CT or MRI after the administration of an intravenous contrast agent. This allows evaluation of blood perfusion to an area of interest, and assessment of other features such as capillary permeability and angiogenesis. Perfusion imaging plays a role in, among other things, the investigation of central nervous system (CNS) tumors, white matter diseases, and acute infarcts in defining penumbra.
Diffusion tensor imaging
Diffusion tensor imaging is an MRI technique that measures the degree of anisotropy (asymmetrical diffusion, also called fractional anisotropy) of water molecule motion in a region of
interest. Capitalizing on its ability to detect the preferential diffusion of water molecules in white matter tracts, DTI is often used to study brain maturation, abnormalities underlying neurological deficits, and white matter injury following traumatic or toxic brain insults.
interest. Capitalizing on its ability to detect the preferential diffusion of water molecules in white matter tracts, DTI is often used to study brain maturation, abnormalities underlying neurological deficits, and white matter injury following traumatic or toxic brain insults.
![]() ▪ FIGURE 2.2 Polymicrogyria. A: Sagittal T1 magnetic resonance imaging (MRI) demonstrates numerous small gyri in a patient with seizures. B: Axial noncontrast CT in the same patient shows apparent pachygyria, failing to define the gray-white detail. |
Magnetic resonance spectroscopy
Magnetic resonance spectroscopy (MRS) uses an MRI scanner to evaluate the chemical composition of a selected volume of tissue. This approach does not yield an image, but rather a spectrum, which is displayed as a series of peaks, whose positions correspond to different metabolites. MRS can be used to study biochemical alterations in neoplasms, infections, neurodegenerative diseases, infarcts, and metabolic disorders.
Functional magnetic resonance imaging
Functional imaging refers to the technique of using an image to display information about the biological activity of an organ or tissue, rather than its anatomical structure alone. Functional MRI (fMRI) is a type of functional imaging that uses the different magnetic properties of oxygenated and deoxygenated hemoglobin to detect and display the pattern of blood flow within the brain that varies according to its activity. Anatomical images obtained during the same examination allow correlation of structure with function. In pediatric patients, fMRI shows promise for the evaluation language disorders and language mapping prior to epilepsy surgery.
Positron emission tomography
Positron emission tomography (PET) is a nuclear medicine imaging technique that employs radioactively labeled, biologically active molecules to obtain functional information. The most commonly used agent is a glucose analogue, fluorine-18 fluorodeoxyglucose (F-18 FDG). A PET scanner detects F-18 FDG taken up by metabolically active cells. PET can be used to study neurological disorders, such as tumors, strokes, seizures, and dementia.
Molecular imaging
In contrast to most types of radiological imaging, which provide anatomical information, molecular imaging refers to the in vivo measurement, analysis, and depiction of biological processes at the cellular and molecular levels. This is a rapidly developing field that holds great promise for the characterization, monitoring, and treatment of disease and for pharmaceutical development. Because it facilitates the detection and characterization of gene and protein abnormalities, as well as the defining of cellular processes, such as signal transduction, molecular imaging will be increasingly central to the translation of basic science into clinical applications within many spheres of medicine (2).
CONSIDERATIONS WHEN IMAGING CHILDREN
Radiological imaging of children involves practical and ethical concerns that should be borne in mind when deciding whether imaging is indicated and, if so, which modality should be employed. Among these concerns are a child’s ability to tolerate the examination, the clinical stability, a need for sedation, and exposure to ionizing radiation. Each of these factors may be more problematical when imaging children than adults.
A typical brain MRI scan with contrast takes approximately 40 minutes to complete, requires complete immobility, and is performed in a noisy, potentially claustrophobic machine. Many children, unable to tolerate this situation, require varying levels of sedation or anesthesia. In addition to their inherent risk, sedation or anesthesia increases the complexity,
expense, and duration of the study. (To some extent, fast, motion-compensated, T2-weighted MRI sequences ameliorate these problems.) Alternatively, the difficulties associated with MRI scanning can be reduced or eliminated by the faster and less threatening environment of CT scanning.
expense, and duration of the study. (To some extent, fast, motion-compensated, T2-weighted MRI sequences ameliorate these problems.) Alternatively, the difficulties associated with MRI scanning can be reduced or eliminated by the faster and less threatening environment of CT scanning.
Perhaps the greatest concern when imaging children is exposure to ionizing radiation and its potential for inducing malignancy. The chief source of radiation exposure in medical imaging is from CT, which accounts for nearly 70% of all medical radiation (3). The use of CT among pediatric patients has increased over the past decades in both absolute and relative numbers, with pediatric studies constituting up to 11% of all CT examinations by 1999 (3,4). Radiation exposure is of relatively greater concern in children compared with adults due to inherently greater radiosensitivity, larger organ doses for a given set of machine parameters, and their longer lifetime during which cancer may develop (4). Of particular note is the radiation dose to the ocular lenses because they are included in most head CT and all paranasal sinus CT examinations. The lenses may begin to develop cataracts after exposure to 0.5 to 2.0 Gy of radiation. However, the typical sinus scan using modern multislice CT scanners imparts a radiation dose of approximately 24.5 mGy to the lens, substantially below the threshold dose. This exposure can be further decreased by using low-dose scanning techniques. In addition, multislice CT scanners have the ability to reformat axial images into additional planes, eliminating the need for direct coronal scanning (5).
Brenner et al. (6) estimate that the lifetime cancer mortality risk attributable to the radiation from a single CT scan in a 1-year-old patient is 0.18% for an abdominal CT and 0.07% for a head CT. Although this radiation dose is not insignificant, it is important to note that these numbers represent a small (approximately 0.35%) increase over the background rate of lifetime cancer mortality (6). In addition, for any given properly selected patient, the benefits of a CT study likely far outweigh the potential risks (7), making it difficult to translate the previous data into appropriate recommendations. Therefore, probably the best advice to physicians who are ordering neuroimaging studies on pediatric patients is to adhere to the as low as reasonably achievable (ALARA) principle of radiation exposure. Indeed, there is anecdotal evidence that a significant proportion of CT scans are not clearly justified by medical necessity (1). Pediatric physicians and radiologists should work closely together to determine whether a CT scan is indicated for a given clinical question or whether another imaging modality, such ultrasound or MRI, could provide an acceptable alternative, thereby eliminating exposure to ionizing radiation. For example, fast MRI techniques, previously discussed, are increasingly used for monitoring pediatric patients with chronic neurological problems, such as hydrocephalus, that require repeated imaging. Even patients with programmable cerebrospinal fluid shunt valves, with the valve “reset” after the procedure, can be imaged this way.
Neuroimaging in Common Clinical Situations
HEADACHES
Headaches are extremely common in children and adolescents, with a prevalence of 37% to 51% in 7 year olds, increasing to 57% to 82% by age 15 (8). The prevalence of migraine headaches in adolescent boys relative to girls is approximately 1:1.5 (3.8% and 6.6%, respectively) (9). Headache symptoms are the presenting complaint in approximately 1.3% of pediatric emergency department visits (10). Although the possible causes of headaches in children are myriad and most often benign, many children with headaches undergo imaging examinations to exclude neurological diseases that require
immediate medical or surgical intervention. However, the role and value of neuroimaging in the evaluation of headache in children remains controversial, in part, because of a paucity of well-designed, sufficiently sized studies (8).
immediate medical or surgical intervention. However, the role and value of neuroimaging in the evaluation of headache in children remains controversial, in part, because of a paucity of well-designed, sufficiently sized studies (8).
A recent practice parameter published by the American Academy of Neurology (AAN) (8) reviewed the available evidence regarding the value of neuroimaging in children with recurrent headaches. Among children imaged with CT or MRI for recurrent headache, 16% had imaging abnormalities, but most of these were incidental or not necessarily surgical (including Chiari malformations, arachnoid cysts, and paranasal sinus disease) (Fig. 2.3). Only 2.3% of patients had lesions that required surgical treatment, such as tumors or vascular malformations. Notably, all patients with lesions requiring surgery had abnormal neurological examinations and no patient with a normal examination had a lesion requiring surgery. This practice parameter therefore concluded that routine neuroimaging is not indicated in children with recurrent headaches and normal neurological examinations.
Neuroimaging should be considered if patients have abnormal neurological findings, especially papilledema, nystagmus, and gait or motor abnormalities (9). Other symptoms or signs that may warrant imaging include the following: recent onset of severe, persistent headache; change in the type of headache; headaches that awaken a child or occur immediately upon wakening; absence of a family history of migraines; associated significant confusion, disorientation, or emesis; and the coexistence of seizures (8,9). In most cases, the imaging modality of choice is MRI.
It is worth mentioning that intracranial aneurysms are rare in children. Their incidence is one per million per year, which is one hundredth of the incidence in adults (11). In a large series, only 0.6% of ruptured aneurysms were found in patients younger than 19 years (12). If a patient presents with the “worst headache of life” and subarachnoid hemorrhage (SAH) is suspected, initial imaging should be with noncontrast CT. However, because CT can miss subacute or small amounts of hemorrhage, a negative CT scan in this clinical scenario should be followed by lumbar puncture. Subarachnoid hemorrhage may also be seen with trauma. When SAH is due to ruptured aneurysm, the hemorrhage is usually extensive and present in the sulci and basal cisterns. By comparison, traumatic SAH is most often seen in the posterior interhemispheric fissure, along the tentorium cerebelli, or within the sulci adjacent to the site of injury. A small amount of SAH in the setting of trauma should not necessarily prompt investigation for intracranial aneurysm.
SEIZURES
Febrile seizures—seizures in infants and children from 6 months to 5 years of age, with associated fever and no evidence of CNS infection or other neurological disease—occur in approximately 2% to 4% of children (13). Other than a slightly increased risk relative to the general population of subsequent epilepsy, simple febrile seizures are considered benign. A practice parameter released by the American Academy of Pediatrics recommends that neuroimaging not be performed in the evaluation of first simple febrile seizures (14).
The role of neuroimaging in the evaluation of children with first nonfebrile seizures without an apparent provocative cause is less well defined. A review and practice parameter published in 2000 by the AAN (15) found that CT and MR scans in these patients showed abnormalities in up to 33%, although significant abnormalities that influenced management were seen in only 0% to 7% of cases, with an average of 2%. Significant imaging findings were more often seen in patients with focal seizures or other specific focal clinical findings, and included lesions, such as tumors, hydrocephalus, subarachnoid or porencephalic cysts, and cysticercosis. MRI abnormalities, such as atrophy, structural brain malformations, and mesial temporal sclerosis, were relatively common but usually did not influence management. Therefore, this AAN practice parameter concluded that
there is insufficient evidence to recommend routine neuroimaging for first nonfebrile seizures in children. However, another review published by the AAN in 2007 found that in children presenting with first seizures, abnormal head CT findings are present in 0% to 21% of patients, and these findings influenced management in 3% to 8% of patients. This review concluded that emergent CT examination is “possibly useful for acute management” of such patients (16).
there is insufficient evidence to recommend routine neuroimaging for first nonfebrile seizures in children. However, another review published by the AAN in 2007 found that in children presenting with first seizures, abnormal head CT findings are present in 0% to 21% of patients, and these findings influenced management in 3% to 8% of patients. This review concluded that emergent CT examination is “possibly useful for acute management” of such patients (16).
![]() ▪ FIGURE 2.3 Chiari I malformation. Sagittal T1 MRI in an adolescent with headache demonstrates tonsillar herniation at the foramen magnum. |
Most sources support the conclusion that emergency imaging is indicated for children who show prolonged focal postictal deficits or who do not return to baseline within several hours, infants younger than 6 months, and for patients with acquired immunodeficiency syndrome (AIDS) (15,16). Nonurgent imaging should be considered for children with seizures who are younger than 1 year or who have abnormal neurological examinations; for those with unexplained, significant cognitive or motor deficits; and for those with partial seizures or electroencephalographic (EEG) findings not consistent with benign partial epilepsy of childhood or primary generalized epilepsy. Although MRI is the modality of choice in most cases, CT may further characterize any lesions (15).
In the case of status epilepticus, imaging plays a role when the cause is unknown. It may identify structural abnormalities or exclude the need for neurosurgical treatment (17). Imaging should be performed only after the child is clinically stabilized and the seizures controlled. Again, MRI is more sensitive and specific than CT, although CT is often more readily available and may require less sedation (Fig. 2.4).
MINOR HEAD INJURY
Head trauma in children accounts annually for more than 500,000 visits to emergency departments and more than $1 billion in health care costs (18). Because most injuries are minor, the optimal neuroimaging is a matter of significant clinical concern. However, this
topic is controversial because of the differing definitions of minor head trauma, difficulty of clinical evaluation, and lack of definitive studies comparing clinical outcomes between different management strategies, such as routine imaging and observation.
topic is controversial because of the differing definitions of minor head trauma, difficulty of clinical evaluation, and lack of definitive studies comparing clinical outcomes between different management strategies, such as routine imaging and observation.
![]() ▪ FIGURE 2.4 Dysembryoplastic neuroectodermal tumor (DNET). Sagittal T2 (A) and contrast-enhanced (B) T1 scans in a 6-year-old with seizures demonstrate a nonenhancing parietal DNET. |
Some authors define minor head trauma as a closed head injury without altered mental status, abnormal or focal neurological examination findings, or evidence of skull fracture at the time of initial evaluation (19). Others employ the Glasgow Coma Scale (GCS), defining minor head trauma by ranges of scores from 13 to 15 (18). Many people use the term “concussion” synonymously for minor head trauma. The AAN defines concussion as “a trauma induced alteration in mental status that may or may not involve loss of consciousness” (20). Regardless of the definition used, the detection of intracranial injury on the basis of physical examination findings is notoriously difficult, and no clinical finding or combination of findings reliably identifies all significant intracranial injuries (21). This further underscores the important role of research in developing guidelines relating to the utility of imaging in the management of minor head trauma. Conversely, in the setting of imaging findings, there is a need to consider the fact that not every abnormality detected with neuroimaging may turn out to be clinically significant. The proportion of children with minor head trauma who will eventually need neurosurgical intervention is small; the exact number is a matter of some debate, although a recent article argued that the number is definitely less than 1% and likely less than 0.5% (22).
There is broad agreement that any patient with evidence of skull fracture should undergo neuroimaging. Signs of skull fracture include hemotympanum, the Battle sign (postauricular ecchymosis), “raccoon eyes” (periorbital hematoma), palpable depressed fracture, serosanguineous leakage from the nose or ear, and cranial nerve palsies. Imaging should be considered for patients with pre-existing disorders that may mask new processes or consequences of trauma, or that may be exacerbated by trauma, such as bleeding diatheses, arteriovenous malformations, and CSF shunts. Imaging is also indicated for patients with altered mental status, GCS of less than 15, a deteriorating clinical course, or focal neurological abnormalities (18,19,21,22). Some authors recommend that imaging be
considered when trauma is associated with loss of consciousness or persistent vomiting (22), or with cases of posttraumatic amnesia, seizures, persistent or worsening headaches, or behavioral changes (18,21). Imaging is not recommended if a child is asymptomatic at the time of evaluation, with a GCS of 15, and if none of the previously mentioned clinical indicators is apparent.
considered when trauma is associated with loss of consciousness or persistent vomiting (22), or with cases of posttraumatic amnesia, seizures, persistent or worsening headaches, or behavioral changes (18,21). Imaging is not recommended if a child is asymptomatic at the time of evaluation, with a GCS of 15, and if none of the previously mentioned clinical indicators is apparent.
If neuroimaging is performed, most sources recommend CT scanning, in large part because of its wide availability, speed, relatively low cost, and superior bony detail. Skull radiographs are generally of limited utility. CT is also the modality of choice for craniofacial fractures, hyperacute or acute intracranial hemorrhage, and especially subarachnoid hemorrhage. In general, CT imaging is sensitive for intracranial pathology that requires medical or surgical treatment, and patients with a normal head CT have a very low risk of later neurological deterioration (18). However, MRI is very sensitive for intracranial pathology and more sensitive than CT for injuries, such as small intracranial hematomas or contusions, diffuse axonal injury, and brainstem injuries (23, 24, 25). MRI should also be considered in cases in which an abnormal clinical examination exceeds the CT findings in severity (Fig. 2.5).
CHILD ABUSE
It has been estimated that up to 30% of children in the United States experience some form of child abuse prior to the age of 18 (26). Physical abuse is the leading cause of death in children. Although abuse accounts for only 10% of injury under the age of 2, up to 50% of such injury includes head injury. Eighty percent of fatal abusive injury is related to head injury. Nonaccidental head injury is responsible for a disproportionate percentage of serious traumatic brain injury, resulting in mortality in 30%, severe neurological deficit in 30% to 50%, and recovery in only 30% of cases (27, 28, 29).
![]() ▪ FIGURE 2.5 Skull fracture, CT. A: Bone window demonstrates right temporal fracture. B: Brain window shows associated extra-axial hemorrhage. |
Shaking and impact mechanisms both result in similar brain injury. Rotational forces generated by angular acceleration and deceleration of the head result in brain movement around its center of gravity, as well as shearing of vascular and axonal structures. These forces are accentuated in the infant, in whom the neck muscles are relatively weak and the head is relatively large, with a head to body weight ratio five times greater than that of the adult. In this same population, the immaturity of the myelin and fragile axonal structure within the brain predisposes its vulnerability to shear injury (30, 31, 32).
The spectrum of findings in nonaccidental head injury includes subdural hematoma (SDH), shear injuries, diffuse axonal injury (DAI), white matter contusions, SAH, and retinal hemorrhage (RH) (33). Several of these were included in Caffey’s initial description of the “whiplash/shaking injury syndrome,” in which SDH, SAH, and RH were coupled with metaphyseal extremity fractures as indicators of non-accidental trauma (34,35). At autopsy, SDH is found in 90% of cases of nonaccidental head injury, in which it is typically bilateral, along the posterior interhemispheric fissure and falx, due to shear injury. This same shear mechanism may result in SAH due to venous tears (36). The incidence of SDH in cases of accidental trauma is only 28%, compared with 81% in cases of nonaccidental trauma (37). In a reported series of 150 infants younger than 24 months hospitalized for head injury, roughly half of the patients (48.7%) had SDH; however, almost two thirds (64.4%) of the SDH in these patients was due to nonaccidental injury. Accidental versus nonaccidental SDH may be differentiated by its imaging appearance. On CT, focal, homogeneous, hyperdense SDH is more common with accidental injury, whereas multifocal, mixed-density SDH is more typical of nonaccidental injury (33,38). SDH is the most common finding in cases of nonaccidental head injury (48.7%); other imaging findings, in order of decreasing frequency, include skull fracture (13.3%), SAH (12%), depressed fracture (10.7%), epidural hematoma (7.3%) contusion (6%), and DAI (2%) (37). In general, skull fracture is uncommon with falls from less than 3 feet. When skull fracture is associated with SDH, or is multiple, complex, depressed, or diastatic, the possibility of nonaccidental trauma should be considered (38).
In the evaluation of nonaccidental trauma, various imaging modalities play a role, including skeletal survey, CT, MRI, and nuclear medicine scans. For head injury, CT best demonstrates fractures, and MRI is more sensitive in detecting and characterizing SDH and shear injury. No single finding is independently diagnostic of abusive head injury. Rather, a constellation of findings, including injuries of different chronicity, may suggest the diagnosis (Fig. 2.6).
Intracranial Neoplasms
GENERAL CONSIDERATIONS
All ages considered, pediatric brain tumors occur in roughly equal frequency within the posterior fossa and supratentorial compartment, although supratentorial tumors predominate in the first 2 years of life (39). Although CT is commonly used in the evaluation of pediatric brain tumors, MRI is the imaging modality of choice, because of its multiplanar capability and its ability to image the posterior fossa without the artifact (related to surrounding dense bone) that hinders CT evaluation of this region. Moreover, MRI is superior to CT for the assessment of subarachnoid metastatic disease (40,41). The intravenous administration of contrast with both CT and MRI is often useful, if not necessary, to further characterize lesions. MRS is a specialized MRI technique that may provide helpful information, by assessing tumor grade and differentiating tumor from granulation tissue
or necrosis. Magnetic resonance perfusion imaging may also contribute to evaluating tumor grade and distinguishing neoplasm from various other brain lesions.
or necrosis. Magnetic resonance perfusion imaging may also contribute to evaluating tumor grade and distinguishing neoplasm from various other brain lesions.
POSTERIOR FOSSA NEOPLASMS
Clinical presentation
Posterior fossa tumors most often present clinically with symptoms related to hydrocephalus and increased intracranial pressure, such as headaches, nausea, vomiting, and increasing head circumference. Patients may also exhibit symptoms referable to cerebellar dysfunction, such as ataxia, nystagmus, and dysdiadochokinesia. Depending on the exact tumor location, cranial nerve deficits and long tract signs may be present, especially with brainstem tumors.
Medulloblastoma
Medulloblastoma is a highly malignant tumor of the posterior fossa and is the most common posterior fossa neoplasm in children. It affects males two to four times as commonly as females and is primarily seen in children (median age at diagnosis is 9 years), although it can occur infrequently in adults. This is an aggressive tumor, with invasion of the brainstem and CSF dissemination commonly seen (42,43).
Medulloblastomas are typically located within the midline cerebellar vermis, behind the fourth ventricle. On CT studies, the lesion classically appears hyperdense, surrounded by hypodense vasogenic edema, and demonstrates homogeneous contrast enhancement. Hydrocephalus is extremely common, due to mass effect on the fourth ventricle, and atypical features such as calcification, cysts, and necrosis may be present (43). The MRI
appearance of medulloblastoma is more variable, with isointense to hypointense signal on T1-weighted images, variable signal intensity on T2-weighted images, and heterogeneous contrast enhancement on post-gadolinium T1-weighted images (42). Contrast-enhanced MR examination of the entire brain and spine is obligatory in cases of medulloblastoma, as CSF dissemination is common and indicates a poorer prognosis (42) (Fig. 2.7).
appearance of medulloblastoma is more variable, with isointense to hypointense signal on T1-weighted images, variable signal intensity on T2-weighted images, and heterogeneous contrast enhancement on post-gadolinium T1-weighted images (42). Contrast-enhanced MR examination of the entire brain and spine is obligatory in cases of medulloblastoma, as CSF dissemination is common and indicates a poorer prognosis (42) (Fig. 2.7).
Atypical teratoid/rhabdoid tumors
Atypical teratoid/rhabdoid tumor is a rare malignant neoplasm that predominantly affects children younger than 2 years. It resembles medulloblastoma histologically and radiologically but is now classified separately because of the tumor’s resistance to standard therapies for medulloblastoma. The prognosis is dismal, with usual survival of less than 1 year after diagnosis (44). The tumor most often arises in the cerebellum, although it may occur supratentorially as well. It may spread through the subarachnoid spaces. Imaging characteristics are similar to those of medulloblastomas and ependymomas, but atypical teratoid/rhabdoid tumors are often more heterogeneous in appearance than typical medulloblastomas (43).
Cerebellar astrocytomas
Astrocytomas are the most common pediatric brain neoplasm overall, and approximately 40% of them occur in the cerebellum (43). Most cerebellar astrocytomas are of the low-grade pilocytic type and typically affect children and young adults. Pilocytic astrocytomas are usually treated with surgical resection, with an excellent prognosis (45).
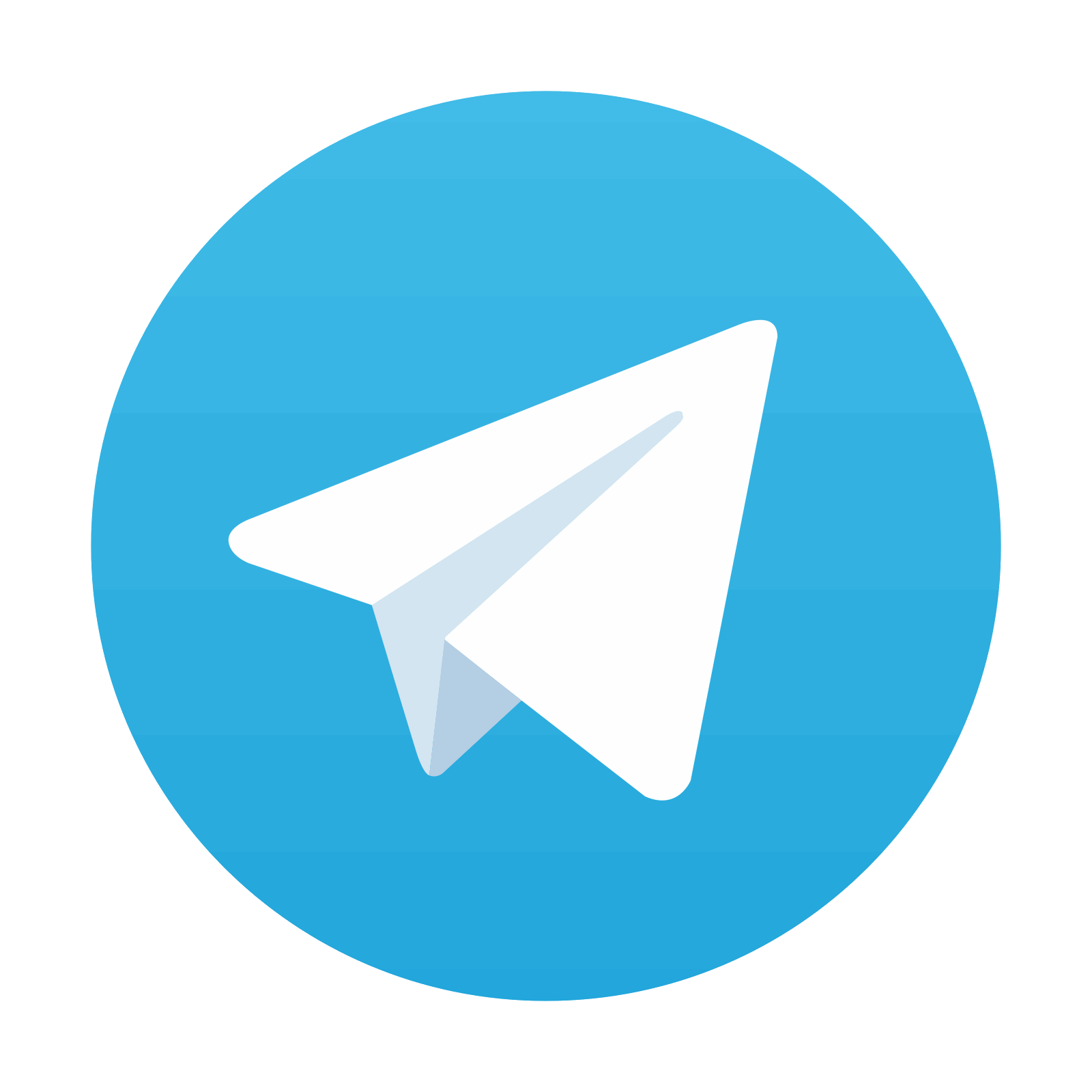
Stay updated, free articles. Join our Telegram channel

Full access? Get Clinical Tree
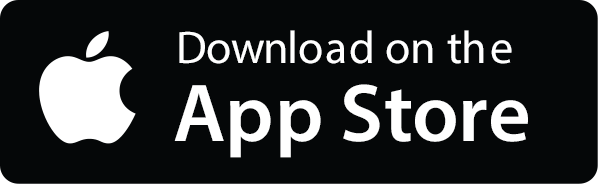
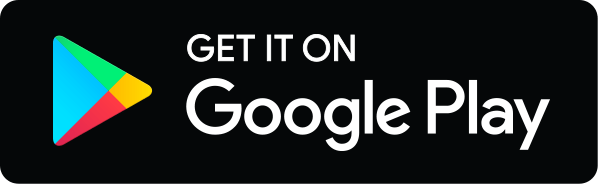
