8 Lawrence C. Wang, Michael T. Zagardo, Kenneth Fraser, and Giuseppe Lanzino Cavernous malformations (CMs) have alternatively been referred to by several terms in the neuroradiologic literature, including cavernous hemangiomas, cavernous angiomas, and cavernomas. CMs account for a significant if not overwhelming percentage of (angiographically) occult cerebral vascular malformations. Computed tomography (CT) and angiography have largely been supplanted by magnetic resonance imaging (MRI) for the diagnosis of CMs, being both insensitive and nonspecific. CMs were known for 50 years prior to the development of MRI, but they were not visualized on a consistent basis. When identified by CT, CMs may show a rounded and well-circumscribed high-density mass with variable focal calcification (Fig. 8-1A, B). There is generally minimal or no mass effect and typically no significant surrounding edema, with limited (Fig. 8-1B) or absent enhancement. Dynamic CT with bolus contrast administration may show slow progressive enhancement on delayed images. More cystic internal regions manifest as low-density foci. Smaller CMs are not consistently seen by CT. As the CT findings are nonspecific, differential diagnostic possibilities include other masses such as hematoma, vascular malformation, and calcified neoplasm. CMs are generally angiographically occult (Fig. 8-2A). Conventional angiography including current digital subtraction techniques typically demonstrates an avascular mass without feeding arteries or draining veins. It is generally considered unnecessary if MRI is characteristic, with no suggestion of abnormal vascularity. In the pre-MRI era, Numaguchi et al.1 described prolonged injection selective cerebral angiography (ICA injection at 3.75 mL/s for a total of 15 mL) for angiographic determination of small venous pools relating to CMs, not visible by less selective angiography with standard length injections. Angiography retains potential utility for differentiating parasellar extra-axial CMs from parasellar meningiomas, as discussed later in this chapter. Angiography will also define associated developmental venous anomalies. Radionuclide imaging can similarly be helpful in the differentiation of parasellar extra-axial CMs from parasellar meningiomas, also discussed later in this chapter. The prototypical appearance of CMs by modern cross-sectional MRI correlates well with their typical pathology, representing benign hamartomas consisting of masses of sinusoidal vascular spaces that are endothelium-lined. These spaces are filled with blood and blood products in various stages of thrombosis and organization. These vascular spaces may be separated by collagenous, fibrous adventitial, or degenerative hyalin material, with no smooth muscle or elastic fibers. Their lack of intervening neural tissue and lack of prominent supplying or draining vessels serve not only to pathologically distinguish CMs from developmental venous anomalies, capillary telangiectasias, and micro-arteriovenous malformations, but also to account for their neuroimaging characteristics. The pathologic correlates to modern neuroradiologic findings in CMs are presciently summarized by Simard et al.2 in their timely 1986 survey of the literature (138 cases). This review was presented at the advent of common clinical usage of high-field MRI, before which CMs were considered rare lesions. They noted the well-established propensity of CMs to bleed, with internal hemorrhagic cystic cavities of varying chronology as a frequent and prominent finding. Microscopic evidence of prior hemorrhage in the form of hemosiderin-laden macrophages, cholesterol crystals, and hemosiderin deposition in surrounding tissues was common as well. Calcification was also common (though not dense and confluent), particularly involving vascular walls thickened with collagen and involving areas of thrombosis with subsequent organization. Various stages of recent and chronic thrombosis were noted within the vascular channels of CMs. Surrounding marginal gliosis was common. In his comment immediately after the Simard review, J.B. Kirkpatrick offered his suspicion that local perilesional edema might contribute to mass effect by CMs. Figure 8-1 Multiple cavernous malformations in a 67-year-old woman presenting with left-sided weakness. (A) Axial noncontrast CT demonstrates a lesion in the inferior aspect of the right frontal operculum. There is also a lesion with punctate calcification involving the head of the caudate nucleus. (B) Axial postcontrast CT shows faint enhancement of both lesions. (C) In axial postcontrast CT, more superior slice shows the complex hemorrhage of the lesion centered at the right frontal operculum. There was no definite enhancement at this level. Parts (D) to (F) are MR images obtained 3 hours after the CT images. (D) Axial post-contrast T1-weighted image shows a faint curvilinear enhancement at the posterior aspect of the more nodular focus within the right frontal opercular/subinsular lesion. A thin hyperintense rim at the periphery of this lesion was present on the noncontrast axial T1-weighted images (not shown), thus representing early methemoglobin formation at periphery of clot. Linear enhancement extends from the anteroinferior aspect of the lesion at the caudate nucleus, corresponding to probable associated developmental venous anomaly. (E) Axial T2-weighted fast spin-echo (FSE) image of type II CM at caudate nucleus, with multinodular hyperintense core with complete hemosiderin rim, is apparent. In correlation with (D), the larger frontal opercular/subinsular lesion is likely for the most part composed of acute and early subacute hemorrhage, a variant type I CM. Edema surrounds the hemorrhage. (F) Axial gradientecho image in which internal architecture of caudate nucleus lesion is not apparent, in contradistinction to internal morphology delineated in (E). Incomplete susceptibility rim is shown about the larger frontal opercular/subinsular CM. More recently, Wong et al.3 presented a transmission electron microscopic examination of CMs. The authors provided further insight into their propensity for recurrent hemorrhage and their imaging characteristics. They described caverns of varying size filled with resorbing intracavernous blood, with compromised interendothelial tight junctions suggesting deficiencies in the integrity of the blood-brain barrier, as well as attenuated cavern walls lacking subendothelial support. With the widespread availability of MRI and its ability to detect differences in tissue contrast not visualized by angiography and CT, direct neuroradiologic-pathologic correlation is possible. The often heterogeneous internal signal intensities of CMs on MRI thus represent acute to subacute blood products surrounded by hemosiderin, in conjunction with variable calcification and variable thickening of the walls of the sinusoids. Figure 8-2 Cavernous malformation masquerading as aneurysm in a 41-year-old woman initially presenting with sudden onset of headache and right retro-orbital pain for 3 days, referred from outside the medical center with diagnosis of partially thrombosed aneurysm, after having had CT and MRI there. (A) Right external carotid angiogram has nonspecific finding of slight meningeal blush from a deep meningeal branch of the middle meningeal artery in the region of the previously identified lesion. Differential possibilities include a dural-based parasellar mass and dural recruitment from the vasa vasorum in relation to a thrombosed aneurysm. No identifiable aneurysm on internal carotid angiography (not shown). (B) Subsequent noncontrast CT shows heterogeneous densities within this ovoid parasellar lesion. No calcification is demonstrated. (C) Magnetic resonance angiography (3D TOF [time of flight] MIP [maximum intensity projection]) in which prolonged T2 relaxation of lesion is apparent. (D to F) MRI (T1-weighted, T2-weighted, and gradient echo) from an examination 1 month after presentation. Note lack of lamellated architecture more commonly seen with thrombosed aneurysms. Surrounding edema (E) and incomplete hemosiderin ring (F) associated with recent hemorrhage. By conventional spin-echo imaging, both acute and chronic hemorrhage will manifest as hypointensity on T2-weighted images. This represents water proton dephasing due to the heterogeneous magnetic susceptibility of such hemorrhages. As this effect is proportional to the square of the magnetic field strength, T2-weighted images on low-field MRI units are not sensitive to such deoxyhemoglobin/hemosiderin effects. Furthermore, the widespread adoption of fast spin-echo T2-weighted sequences on high-field systems has resulted in a decreased sensitivity to hemorrhage. The aforementioned proton dephasing is in large part prevented by the physics of fast spin-echo imaging, wherein the interecho times are shortened by a train of 180-degree refocusing pulses. Figure 8-3 Pontomedullary cavernous malformation with associated transpontine developmental venous anomaly in a 31-year-old woman with severe nausea and vomiting. (A) Axial T1-weighted image shows intra-axial lesion with heterogeneous hyperintensity. (B) Axial fluid attenuated inversion recovery (FLAIR) image. Perilesional edema is well demonstrated. (C) Axial T2-weighted image. Extent of peripheral hemosiderin of CM approximates pial surface at, and anterior to, the porus acusticus. (D) Coronal T2-weighted image shows acute hemorrhage with perilesional edema and incomplete hemosiderin rim along inferior aspect of CM. (E) Sagittal postcontrast T1-weighted image shows transpontine developmental venous anomaly (DVA) along superior aspect of CM. (F) Coronal postcontrast T1-weighted image shows medullary veins of DVA converging radially. Gradient-recalled echo sequences omit a 180-degree refocusing pulse and thereby provide for increased sensitivity to magnetic susceptibility effects. The blood products in CMs will have accentuated signal loss, particularly when longer echo times (TE) and lower flip angle protocols are employed. However, internal signal characteristics of CMs can be obscured by this signal loss (Fig. 8-1F). Alternatively, Kim et al.4 demonstrated artifactual increased signal intensity on gradient-echo imaging within lesions in a patient with multiple CMs. His group verified experimentally that hemosiderin deposits within CMs can act as point magnetic dipoles, with intravoxel dephasing resulting in a high-intensity ring artifact within the expected region of signal void on axial sections. Parallel to the main magnetic field (i.e., on coronal and sagittal sections on the typical MRI unit), trilobed and cloverleaf (four-lobed) hypointense artifacts on gradient-echo imaging were also observed. These susceptibility-induced intravoxel signal interference patterns could easily be respectively misinterpreted as a ring-like deposition of material within a CM or multiple contiguous lesions. Another caveat associated with gradient-echo imaging is the lack of specificity of the aforementioned susceptibility effects and artifacts, which are not exclusive to hemosiderin. These findings can be seen with chronic hemorrhage breakdown products in the setting of parenchymal hematoma, as well as hemorrhagic or melanotic metastases, brain iron deposits, calcification, other paramagnetic ions, and susceptibility artifact near brain-air interfaces. The gradient-echo images should thus be correlated with the remainder of the MR examination and with the clinical context. Postcontrast imaging of CMs is helpful for exclusion of coexistent developmental venous anomalies (DVAs), seen in approximately one quarter of patients with CMs in a recent large series5 (Figs. 8-1D, 8-3E, and 8-3F). This is prudent in patients for whom surgical resection of a symptomatic CM is contemplated, given the risks associated with inadvertent coexistent DVA resection. Lesions of the spinal cord share common histologic features with those in the intracranial compartment. Intramedullary lesions exhibit a discrete central core, often of mixed signal intensity as with intracranial lesions, with variable surrounding low signal intensity hemosiderin on T2-weighted images. There can be CM perilesional cord edema manifested as increased signal intensity on T2-weighted images and short tau inversion recovery (STIR) images (Fig. 8-4). Imaging of the entire neuraxis will often reveal the presence of multiple lesions.6 CM localization by MRI does not only guide the specific surgical approach; indications of surgical accessibility based on MRI also help to determine whether to operate on, or to expectantly manage, patients who are asymptomatic or mildly symptomatic.
Neuroradiology and Classification of Cavernous Malformations
Computed Tomography, Angiography, and Radionuclide Imaging of Cavernous Malformations
Magnetic Resonance Imaging of Cavernous Malformations
Correlation of Findings with Pathology
Magnetic Resonance Imaging Of Intramedullary Spinal Cord Cavernous Malformations
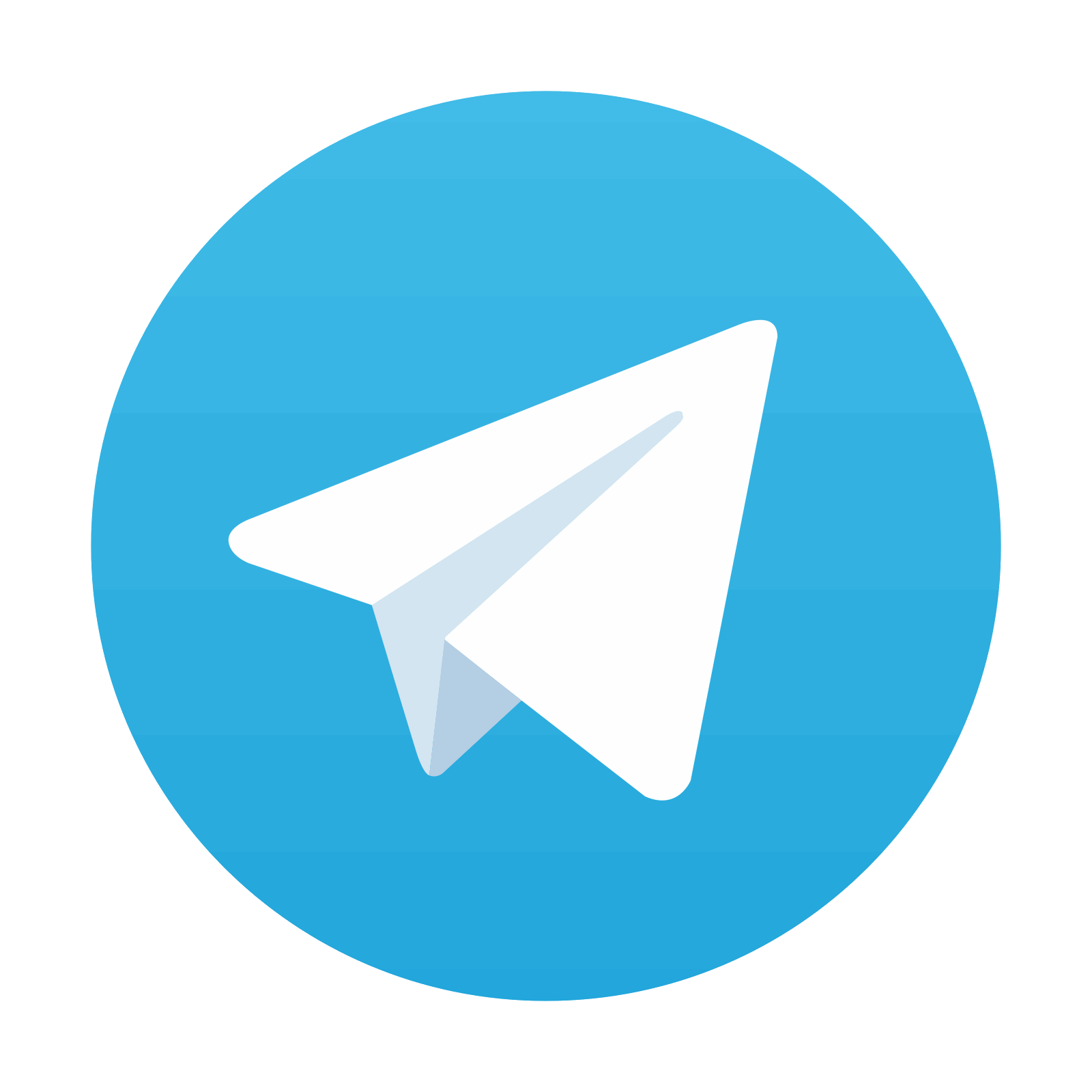