Fig. 2.1
The lunate sulcus forming the boundary between the parietal and occipital lobes on the left hemisphere of a chimpanzee brain. The location of the lunate sulcus in hominin fossil endocasts has been highly debated
Much of the debate regarding the position of the lunate sulcus has focused on australopithecines, from approximately 4 to 2.5 million years ago, particularly the Taung endocast of Australopithecus africanus. Whereas Falk (1980, 1983, 1985a, b, 1989) identifies the australopithecine lunate sulcus more rostrally in an ape-like position, Holloway (1981a, 1983, 1984, 1988, 1992), Holloway and Shapiro (1992), and Holloway et al. (2004) assert that the position of the lunate sulcus is more caudal, similar to modern humans. Because australopithecines have relatively small cranial capacities in the range of great apes, a rostrally positioned lunate sulcus would suggest that reorganization had not occurred in these species, and did not occur in the hominin lineage prior to brain enlargement. A caudally positioned lunate sulcus, however, would suggest that reorganization in the parietal and occipital lobes had occurred in these relatively small-brained species prior to brain enlargement in the hominin lineage (Holloway et al. 2004).
Recent fossil discoveries have provided further evidence for the possibility of reorganization in other regions of the cerebral cortex in hominins without an increase in brain size. The discovery of A. sediba from about 2 million years ago suggests the possibility that cortical reorganization had occurred prior to brain size enlargement in hominins. The endocast of A. sediba has a relatively small endocranial volume comparable in size to earlier Australopithecus species. However, the morphology of its orbitofrontal region is claimed to be more similar to that seen in later Homo (Carlson et al. 2011). Further complicating the debate is the endocranial morphology of the H. floresiensis specimen LB1. The position of the lunate sulcus in the LB1 endocast of H. floresiensis from 94,000 to 13,000 years ago has been identified in a more posterior modern human-like position and it displays other derived morphology of the frontal and temporal lobe similar to that of Homo erectus, even though it has an incredibly small cranial capacity of 417 cc (Falk et al. 2009).
Relative sizes of the various lobes of the cerebrum have also been estimated to differ among hominin species with similar brain sizes, suggesting that changes can occur in a mosaic fashion without a change in overall brain size. Balzeau et al. (2012) examined the surface areas of the frontal, parieto-temporal, and occipital lobes relative to endocranial volume for Homo endocasts. Humans and Neandertals exhibited marked differences in relative lobe size despite similar endocranial capacities; Neandertals had relatively larger frontal and occipital lobes and a relatively smaller parieto-temporal lobe than humans. Evidence for parietal and cerebellar expansion in humans has also been demonstrated using geometric morphometrics techniques (GM). GM is based on the use of landmarks to define the shape of an object. Landmarks are biologically homologous loci across all specimens, and contain spatial data that can be separated according to size and shape information (Zelditch et al. 2004). The use of GM methods has transformed the field of paleoneurology, as it allows for a quantitative, rather than descriptive, study of shape changes among different specimens. Using GM, Neubauer et al. (2009) investigated the growth pattern of the endocranium throughout human development. The parietal and cerebellar regions of the cranial vault undergo pronounced expansion during early postnatal development. This pattern of globularization during early development is not seen in chimpanzees, as humans and chimpanzees differ for their entire cranial growth trajectory (Neubauer et al. 2010). Although modern human and Neandertal crania both have relatively large endocranial capacities, they differ in both adult and developmental endocranial shape; Neandertals lack the early postnatal globularization phase seen in the human cranium, and develop along a trajectory that results in a more elongated cranial shape (Gunz et al. 2010, 2012). These results show that cranial growth trajectories have influenced the shape of the modern human brain, independently of changes in overall brain size (Bruner 2004).
Thus, there is considerable evidence to indicate that reorganization has occurred in parallel with brain expansion in hominin evolution. However, it is impossible to know the full extent of reorganizational changes in human brain evolution based solely on the fossil record. Although endocasts provide the only means of studying neural morphology of extinct hominins, the information they offer is limited to overall size differences, imprints of cerebral vasculature, the external convolutional morphology of well-preserved gyri and sulci, and changes in shape (Falk 2012). Although important information can be gained from such studies, they do not provide insight concerning changes that occurred in the microstructure, connectivity or molecular biology of the brain. Furthermore, modifications of internal structures, such as the basal ganglia, thalamus, and other deep nuclei cannot be examined from endocasts. Comparative neuroanatomical and genetic data across primates, therefore, is needed to reveal possible changes in microstructure, connectivity, and function that cannot be detected in endocasts (Fig. 2.2). The knowledge gained from such research has the potential to inform our interpretations of how the earliest hominins may have differed from the living great apes, and how later Homo may have evolved modern behaviors such as language.


Fig. 2.2
Different levels of examining brain reorganization, with a description of methods discussed in this chapter. Macrostructure includes large, visible structures of the brain. Macrostructure is the only level that can be investigated in endocasts. Microstructure is the next level, and allows for the analysis of cytoarchitecture and connectivity between different brain regions. Molecular biology refers to neurotransmitters, receptors, genes, and other molecular components of the brain
Reorganization in Structure
Recent findings have provided a better understanding of how regions of the human cerebral cortex have changed compared to other primates in terms of size and position. The frontal lobe has been identified as a region that is noticeably enlarged and widened in Neandertals and modern humans compared to other hominins (Bruner and Holloway 2010). It is notable that the primate frontal cortex (including primary motor cortex, premotor cortex, supplementary motor area, and prefrontal cortical areas combined) hyperscales relative to the rest of the neocortex and brain, with a steeper scaling exponent than what is observed in carnivores (Bush and Allman 2004). The human brain does not differ from other primates in this respect (Bush and Allman 2004), and the total frontal lobe is as large as expected for an ape of human size (Semendeferi and Damasio 2000; Semendeferi et al. 2002; Barton and Venditti 2013). Furthermore, the human frontal cortex, though absolutely larger in humans, occupies a similar proportion of the cortex as in nonhuman primates (Semendeferi et al. 2002). Thus, humans appear to follow an expected pattern of total frontal cortex enlargement for a primate of our brain size. More uncertainty surrounds the question of prefrontal cortex enlargement in human evolution. The granular prefrontal cortex of humans contains regions that are important for language, social cognition, abstract thinking, long-term planning, and other executive functions. The frontal pole region (area 10) has been measured based on cytoarchitecture across hominoid species and has been shown to be both absolutely and relatively larger compared to the rest of the brain in humans than in other apes (Semendeferi et al. 2001), though human area 10 is only approximately 6 % larger than expected for an ape of human size (Holloway 2002). Other studies of the whole prefrontal cortex have concluded that it is larger than expected by allometry in humans for both grey and white matter (Schoenemann et al. 2005a; Smaers et al. 2011; Sherwood and Smaers 2013).
Less is known about how reorganization may have affected other brain regions. The temporal lobe in humans is larger than expected for an ape brain of human size (Semendeferi and Damasio 2000; Rilling and Seligman 2002). Humans also have larger than expected temporal lobe surface area and white matter (Rilling and Seligman 2002). Enlargement of the temporal lobe may be due to adaptations for language, as it contains the auditory cortex and Wernicke’s area, two regions important for language processing, along with other regions such as the middle and inferior temporal gyri that are active in phonological and lexical-semantic functions (Binder et al. 1997). The parietal lobe has been difficult to differentiate from other regions, as its boundaries are not easily identified. Semendeferi and Damasio (2000) showed that the occipito-parietal sector, which is the combined volume of the parietal and occipital lobe, is as large as expected for an ape brain of human size. Although the total volume of the occipito-parietal sector is not larger than expected in humans, more fine-grained reorganization in this region is evident in modern humans; the size of the parietal lobe appears to have become more rounded in modern humans compared to Neandertals (Bruner 2004, 2008; Bruner et al. 2011), while a comparative study including humans and apes showed that the size of the adjacent primary visual cortex in the occipital lobe is reduced in modern humans compared to hominoids (de Sousa et al. 2010).
In sum, reorganization of neocortical regions of the human brain beyond what is expected by allometry appears to be relatively subtle for most regions. Many additional significant reorganizational differences are seen in the microstructure and molecular biology of the human brain. Microstructure consists of smaller components that form the larger, visible structures of the brain, and includes cytoarchitecture and connectivity. Cytoarchitecture refers to the organization of neurons and glia within neural tissue. Connectivity involves the structure and pathways of white matter tracts that traverse the brain and connect different regions. These can be large pathways, such as the corpus callosum, or smaller ones that connect neighboring regions of tissue, such as the “U-fibers” that connect adjacent gyri in the neocortex. Brain microstructure is an important component of brain function and studies of comparative neuroanatomy have revealed key differences at this microscopic level between humans and nonhuman primates that may have resulted in unique aspects of human cognition.
One major question concerning brain reorganization is whether one unit of neocortex has a uniform structure and functional processing capacity among species (Holloway 1968). Many models assume this is true, and propose that processing power of cortical tissue does not vary significantly along with brain size changes. The assumption that brains of different species are generally comparable on the basis of simple measures of size was bolstered by the influential publications of Jerison (1973) and Rockel et al. (1980). More recent evidence from comparative studies of the cytoarchitecture of the neocortex, however, indicates that the contents of 1 mm2 of neural tissue may not be uniform across species. Cortical uniformity assumes that 1 mm2 of cortex contains a constant number and density of neurons across species. In primate species, however, the average number of neurons under 1 mm2 of cortex varies tremendously, with total surface area of the cortex increasing more slowly than neuron number (Herculano-Houzel et al. 2008).
Analyses of minicolumns throughout the neocortex also bring the hypothesis of cortical uniformity into question. Minicolumns are single-neuron wide vertical arrays that traverse the cortical layers, and are considered to be an important functional unit of the cerebral cortex (Buxhoeveden and Casanova 2002). Two measurements of cytoarchitecture, gray-level index (GLI) and horizontal spacing distance (HSD), have been used to quantify neuron organization in minicolumns of the primate neocortex. GLI is a measure of the percent-area of perikarya, or neuronal cell bodies, compared to neuropil (Schleicher and Zilles 1990; Semendeferi et al. 1998), while HSD indicates the amount of neuropil space separating minicolumns from each other horizontally (Semendeferi et al. 2011). Neuropil consists of dendrites, axons, synpases, glial cell processes, and vasculature (Spocter et al. 2012) and the amount of neuropil can be used to estimate interconnectivity of surrounding neurons (Wree et al. 1982).
Comparisons among humans, apes, and macaque monkey minicolumns throughout the neocortex show variability in neuron density, horizontal spacing, and amount of surrounding neuropil (Fig. 2.3). In the frontal lobe, there is proportionally more neuropil in humans than in great apes for Broca’s area (Schenker et al. 2008) and the frontal pole (Semendeferi et al. 2001). The GLI value (i.e., space occupied by cell somata) is lowest in the human frontal pole despite being both absolutely and relatively larger than that of great apes, reflecting increased connectivity in the larger human brain (Semendeferi et al. 2001). HSD was also largest in the human frontal pole and was 30 % larger than in great ape species, likely related to more corticocortical integration and processing power (Semendeferi et al. 2011). The frontal pole region may have become more specialized in hominin evolution, with increasing neuropil space providing more processing capacity in this and other prefrontal regions, including the anterior region of the insular cortex (Semendeferi et al. 2001; Spocter et al. 2012).


Fig. 2.3
Minicolumns in layer III of left area Tpt (Wernicke’s area homologue) of a macaque (a), chimpanzee (b), and human (c). Magnification = 10x and scale bar = 10 mm for all photos
Studies of microstructure in the temporal lobe have focused on the cortex of the planum temporale (PT). The PT is a region that is part of Wernicke’s area and is associated with speech comprehension in humans (Hickok 2009). Cytoarchitectonic area Tpt is located in the posterior portion of PT, sometimes extending into adjacent regions of the parietal cortex (Galaburda and Sanides 1980; Galaburda and Pandya 1983). Humans are reported to have the lowest neuron density in this cortical region as compared to chimpanzees and macaques, with the least linear and widest minicolumns (Buxhoeveden et al. 1996). Despite lower neuron density, the core of human minicolumns is more compact than in chimpanzees and macaques. Lower neuron density indicates increased neuropil space and connectivity in humans. Despite a large size difference between chimpanzee and macaque brains, both have similar minicolumn neuron density and neuropil space (Buxhoeveden et al. 1996, 2001). This evidence suggests that the human neocortex may have increased minicolumn size associated with more input and output pathways for language processing (Buxhoeveden et al. 2001). Although one study concluded that humans show a leftward predominant asymmetry of minicolumn spacing and neuropil in the PT which was absent in chimpanzees (Buxhoeveden et al. 2001), a more recent study with a larger sample size failed to replicate this earlier finding and showed that neither humans nor chimpanzees display neuropil asymmetry (Spocter et al. 2012). Humans also show differences from chimpanzees in minicolumn morphology in the fusiform gyrus of the temporal lobe. In both species, the fusiform gyrus is involved in facial recognition. Humans have larger neurons and increased neuropil space in the fusiform gyrus than in chimpanzees, particularly in the left hemisphere. The asymmetry may be associated with a functional difference for the left FFA in humans (Chance et al. 2013).
In the occipital lobe, minicolumn width in the primary visual cortex is larger and more variable in humans than in chimpanzees and macaques, with a corresponding increase in neuropil. As in the cortex of the PT, chimpanzees and macaques are similar in minicolumn width and variability despite a difference in size, suggesting increased processing power in the human primary visual cortex (Casanova et al. 2009). It is notable that such microstructural reorganization is evident in the human primary visual cortex, since in terms of volume, humans have a particularly reduced V1 as compared to what would be expected based on scaling for brain size (de Sousa et al. 2010). Additional histological evidence suggests that layer IVA of V1, which is involved in motion processing, has also been altered in recent human evolution (Preuss and Coleman 2002). Humans differ from anthropoid primates in the structure of the magnocellular pathway in the primary visual cortex, and differ from great apes in the organization of V1 (Preuss et al. 1999).
Humans also have astroglial specializations associated with minicolumn anatomy that are unique among primates. The primate neocortex contains astroglial cells with long processes that extend vertically across the cortical layers (Colombo et al. 1997, 2000; Oberheim et al. 2006; Sherwood et al. 2009). These astroglial processes are closely associated with pyramidal cell columns and are concentrated in layers II and III in most primates, suggesting a pattern of organization similar and complementary to cortical minicolumns (Colombo et al. 1999). Anthropoids, especially catarrhines, have a vertical, radial distribution of long interlaminar astroglial processes that form a palisade in the superficial cortical layers that may increase the functional capability of the cortical minicolumns by facilitating communication among them (Colombo and Reisin 2004). Compared with other cararrhines, humans have increased branching complexity of astroglial cells, which may contribute to synthesis of processing in cortical minicolumns (Oberheim et al. 2009).
Studies of minicolumns and corresponding astroglial organization demonstrates that the anatomical structures underneath 1 mm2 of neocortex is not constant across species, or even across the neocortex of a single individual. Although larger brains are expected to have decreased neuron density and increased neuropil (Jerison 1973), evidence from the diversity of minicolumn structure across mammals suggests that brain size alone may not be sufficient to explain this aspect of cortical microstructure (Raghanti et al. 2010).
Reorganization in Connectivity
Major differences between humans and other primates, furthermore, may lie in patterns of connectivity among brain regions. White matter is especially important for connectivity as it contains tracts of axons (Schüz and Braitenberg 2002). However, white matter is impossible to assess using endocasts, and is most readily studied in brain tissue using magnetic resonance imaging (MRI) and diffusion tensor imaging (DTI), a variant of MRI. Both MRI and DTI can be used to study white matter in vivo, and have been applied to the study of connectivity in humans and nonhuman primates. DTI, however, has the added advantage of revealing specific white matter tracts (Rilling 2006). Evidence from MRI scans suggests that frontal lobe white matter may differ between humans and nonhuman primates. There is a general trend of whole brain neocortical white matter to increase disproportionately with gray matter in mammals (Zhang and Sejnowski 2000), and anthropoids exhibit different scaling patterns for frontal lobe and non-frontal lobe white matter (Smaers et al. 2010; Schoenemann et al. 2005a, b). This differential scaling results in a white matter to gray matter ratio in the prefrontal cortex that is greatest in humans (Sherwood and Smaers 2013). Primates also demonstrate hyperscaling of the left prefrontal cortex. Asymmetrical hyperscaling occurs in left prefrontal white matter volume compared to gray matter volume in apes and humans, but not in monkeys. Although apes and humans share this pattern of hyperscaling, the trend is taken to its extreme in humans (Smaers et al. 2011). Areas of the cerebellum that are connected with the expanded prefrontal cortex in humans have also enlarged compared to the same areas in capuchins and chimpanzees (Balsters et al. 2010), suggesting that other brain regions may have become relatively enlarged due to connectivity with the prefrontal cortex.
Zhang et al. (2012) investigated connectivity-based cortical landmarks identified in macaques, chimpanzees, and humans using DTI. These landmarks showed where white matter connectivity was similar or different across the three species. Areas with more common landmarks were considered more evolutionarily conserved, with fewer changes in patterns of white matter connectivity. Overall, there were 65 common landmarks and 175 differences across species. The most similarities were found in the frontal and occipital lobes and fewer were located in the temporal lobe. In the parietal lobe, the superior portion had more common landmarks, while the inferior portion contained more species differences. Although all regions showed some major differences, highly specialized regions in humans, such as Broca’s and Wernicke’s areas, showed a large change in white matter connectivity. These results suggest that connectivity has changed significantly across different regions during primate evolution, and may be linked with human-specific capacities such as language.
One of the major long-range white matter pathways in the primate brain is the superior longitudinal fasciculus (SLF). It is made up of four parts: three branches (SLF I, II, and III) and the arcuate fasciculus (Makris et al. 2005). The evolution of language capabilities may have influenced the connectivity between the frontal and temporal lobes in humans along the arcuate fasciculus. The arcuate fasciculus connects the frontal and temporal cortices, specifically Broca’s and Wernicke’s areas. The arcuate fasciculus is involved in higher order cognition including syntax and semantics (Rilling et al. 2012). DTI suggests that the human arcuate fasciculus has a different pattern of connectivity compared with that of chimpanzees and macaques. In humans, the portion of the arcuate fasciculus connecting the left frontal cortex to the middle and inferior temporal gyri is enlarged (Rilling et al. 2008). This portion of the arcuate fasciculus is specifically associated with speech prosody (rhythm and tone) and lexical-sematic (word meaning) processing in humans (Glasser and Rilling 2008). The human arcuate fasciculus also exhibits a higher level of modification compared with the extreme capsule. The extreme capsule is a ventral white matter pathway that is associated with auditory processing and memory retrieval in macaques. In humans, the extreme capsule is involved in retrieval of verbal information. The arcuate fasciculus experienced the most change in the human lineage, suggesting a reorganization of processing capabilities in humans due to the evolution of language (Rilling et al. 2012).
Expanded tool-use and tool-making behaviors in modern humans may have also affected white-matter connectivity in the mirror neuron system. The mirror neuron system is comprised of frontal, parietal, and temporal areas that are active during observation and execution of hand and hand-object manipulation (Rizzolatti and Craighero 2004). Mirror neurons were first identified in macaques (Gallese et al. 1996; Rizzolatti et al. 1996), and their presence has since been extended into humans (Iacoboni et al. 1999; Gazzola and Keysers 2009; Keysers and Gazzola 2010). Using DTI, Hecht et al. (2013) investigated the connectivity of the mirror neuron system in macaques, chimpanzees, and humans, specifically connectivity among frontal area F5c in macaques/area 44 in chimpanzees and humans, the inferior parietal lobule (IPL), and the superior temporal sulcus. Connections among these regions fall along ventral (extreme/external capsule) or dorsal (inferior/middle longitudinal fasciculi and SLF III) pathways that are shared with language processing. The ventral pathway is associated with goal-directed observations, while the dorsal pathway is involved in extracting detailed information from observed actions. Macaques, chimpanzees and humans differ in the pattern of connectivity among these areas; macaques and chimpanzees exhibit more connectivity via the ventral pathway, though this pattern is less pronounced in chimpanzees, while human connectivity occurs primarily through the dorsal pathway. Connections between the IPL and the inferior temporal cortex are also smallest in macaques, larger in chimpanzees, and largest in humans. These connections link the IPL with regions in the temporal lobe associated with tool recognition, with stronger connections indicating that information concerning tool type can be more easily integrated with information about tool actions. Connections between the frontal and parietal mirror neuron regions extend furthest into the superior parietal lobule (SPL) in humans. The SPL is associated with attention and spatial tasks (Husein and Nachev 2007), indicating that the human mirror neuron system relies more on these functions than in chimpanzees and macaques. These results indicate that variation in the connectivity of the mirror neuron system in macaques, chimpanzees, and humans can be linked with differences in capacity for tool-use and tool-making. Chimpanzees and humans are better able to imitate observed actions than are macaques, while humans have increased capacity for imitation of highly detailed actions compared with chimpanzees.
Von Economo neurons (VENs) are large, spindle-shaped neurons with one large axon and one primary apical dendrite on the opposite end. They were first identified in the anterior cingulate cortex (ACC) and frontoinsular cortex (FI) of humans, then subsequently great apes as well (Nimchinsky et al. 1999). In humans, VENs have also been found in the dorsolateral prefrontal cortex (DLPFC) (Nimchinsky et al. 1999; Fajardo et al. 2008). VENs are more numerous in humans than in apes (Allman et al. 2010, 2011), and also differ biochemically in humans (Stimpson et al. 2010). VENs have been found in the ACC, anterior insula, and frontopolar cortex of cetaceans (Butti et al. 2009), and the FI and ACC of elephants (Hakeem et al. 2009), though not in the same pattern as seen in great apes and humans. VENs have also recently been identified in the macaque anterior insula and ACC, though they were smaller than those in humans, chimpanzees and bonobos (Evrard et al. 2012). Neurons with VEN-like morphology have been observed in smaller numbers in other cortical areas throughout many mammals (Butti et al. 2013), but they appear to have a specialized cortical distribution in particular large-brained and social species.
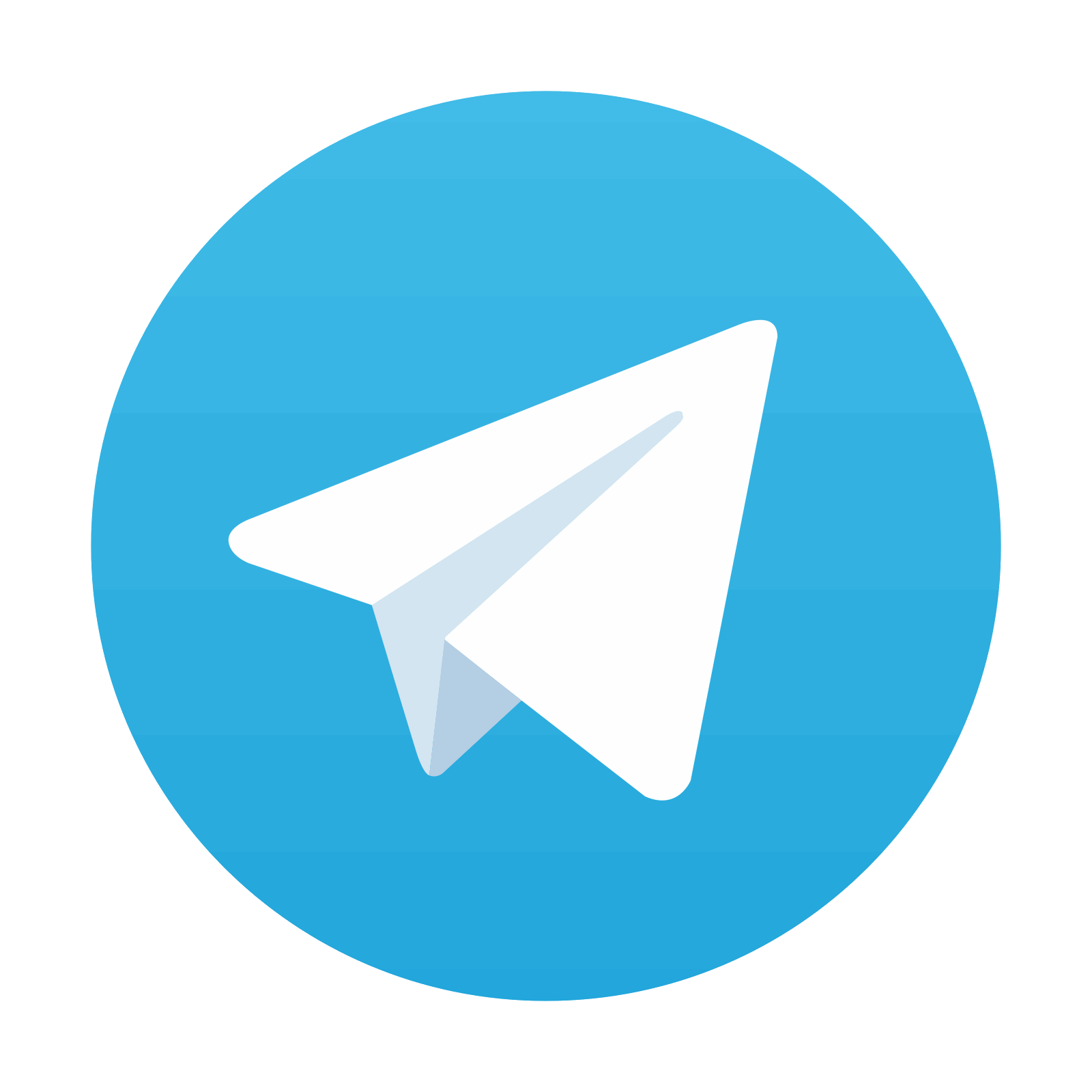
Stay updated, free articles. Join our Telegram channel

Full access? Get Clinical Tree
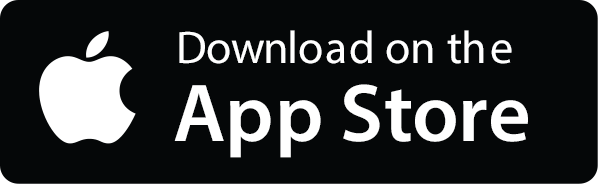
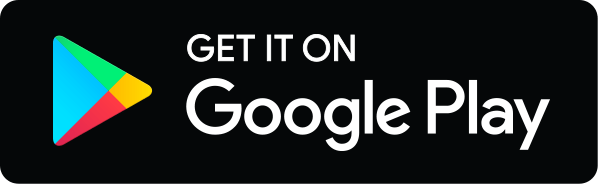