Magnetic resonance guided focused ultrasound surgery (MRgFUS) has potential noninvasive effects on targeted tissue. MRgFUS integrates MRI and focused ultrasound surgery (FUS) into a single platform. MRI enables visualization of the target tissue and monitors ultrasound-induced effects in near real-time during FUS treatment. MRgFUS may serve as an adjunct or replace invasive surgery and radiotherapy for specific conditions. Its thermal effects ablate tumors in locations involved in movement disorders and essential tremors. Its nonthermal effects increase blood-brain barrier permeability to enhance delivery of therapeutics and other molecules.
Key points
- •
Magnetic resonance–guided focused ultrasound surgery (MRgFUS) is a noninvasive, image-guided therapy method that treats neoplasms and target tissue using thermal ablation and/or nonthermal effects.
- •
Thermal ablation involves the heating of target tissues using ultrasound waves to yield cellular damage and coagulative necrosis.
- •
Nonthermal effects include modulation of cellular permeability and cavitation, which can contribute to enhanced drug delivery through the blood-brain barrier.
Introduction
Magnetic resonance–guided focused ultrasound surgery (MRgFUS) is a therapeutic modality that has gained interest in recent years for its potential use in noninvasive tumor ablation and treatment, and more recently in the treatment of essential tremors. Although surgery and radiation therapy currently provide the standard of care for many oncological conditions and have been more extensively implemented in the clinic, these are associated with differential outcomes and long-term toxicities. The benefit of MRgFUS is its potential to establish a treatment that can be maximally effective with minimal side effects.
MRgFUS is the integration of 2 widely used imaging and therapy processes, MRI and focused ultrasound surgery (FUS), respectively. MRI is a long-established imaging methodology that provides high-contrast resolution for planning treatments and quantifying effects, namely through accurate target delineation, real-time monitoring of temperature changes, and modulation of energy deposition in the tissue of interest. Whereas MRI is the monitoring component of MRgFUS, FUS is the therapeutic component; ultrasound beams can cause cellular damage in a thermal ablative or nonthermal manner. Together, MRI and FUS provide an unparalleled ability to directly visualize tumors as they are treated with ultrasound waves and monitor effects in near real-time. With further research, this has the potential to serve as an adjunct or even replace current invasive surgery and radiotherapy therapeutic courses for specific tumors and diseases. This article discusses the principles and applications of thermal ablation and nonthermal effects in cancerous and noncancerous central nervous system (CNS) disease.
Introduction
Magnetic resonance–guided focused ultrasound surgery (MRgFUS) is a therapeutic modality that has gained interest in recent years for its potential use in noninvasive tumor ablation and treatment, and more recently in the treatment of essential tremors. Although surgery and radiation therapy currently provide the standard of care for many oncological conditions and have been more extensively implemented in the clinic, these are associated with differential outcomes and long-term toxicities. The benefit of MRgFUS is its potential to establish a treatment that can be maximally effective with minimal side effects.
MRgFUS is the integration of 2 widely used imaging and therapy processes, MRI and focused ultrasound surgery (FUS), respectively. MRI is a long-established imaging methodology that provides high-contrast resolution for planning treatments and quantifying effects, namely through accurate target delineation, real-time monitoring of temperature changes, and modulation of energy deposition in the tissue of interest. Whereas MRI is the monitoring component of MRgFUS, FUS is the therapeutic component; ultrasound beams can cause cellular damage in a thermal ablative or nonthermal manner. Together, MRI and FUS provide an unparalleled ability to directly visualize tumors as they are treated with ultrasound waves and monitor effects in near real-time. With further research, this has the potential to serve as an adjunct or even replace current invasive surgery and radiotherapy therapeutic courses for specific tumors and diseases. This article discusses the principles and applications of thermal ablation and nonthermal effects in cancerous and noncancerous central nervous system (CNS) disease.
Thermal ablations
The mechanism of thermal ablation of tumors involves the conversion of mechanical energy into heat through the steering of multiple ultrasound waves to a targeted location of tissue. These waves, also called sonications, are highest and most intense at their point of convergence. When the tissue is heated to approximately 57°C to 60°C for at least 1 second at the target tissue’s point of conversion, protein denaturation, coagulative necrosis, and eventual complete ablation of the tissue will occur. This location is typically 2 mm (very precise) and thus multiple simultaneous or parallel sonication points can be done for larger ablation zones.
As mentioned, a benefit of FUS is its noninvasive nature and that it is unique in its ability to generate mechanical energy from ultrasound waves, which is converted to heat and hits the target focal area without harming adjacent tissue in its trajectory. With surgery and radiation therapy, excision of the tumor region without affecting surrounding normal tissue is not possible; MRgFUS can act as an alternative in patients for whom these other options may not be feasible due to the associated risks. In fact, this method becomes even more relevant for neoplasms that are located in regions of the body where affecting nearby tissues incidentally can yield severe, long-lasting damage, such as deep-seeded neoplasms in the brain. Given the highly invasive nature of accessing the brain in surgery, and the nonspecificity and scatter issues that plague radiation therapy, using MRgFUS for thermal ablation in the brain can provide an accurate and efficient modality to access more sensitive and hard to reach tumors. Early in the implementation of this method, the skull provided a challenge to the accurate convergence of sonications to tissues of interest given the irregular thickness of the skull that leads to phase distortions and aberrations. In addition to these irregularities, there was excessive heating of the skull caused by the bone being able to absorb up to 50 times more acoustic energy than soft tissues. These issues have led to the establishment of hemispherically configured phased-array transducers that can evenly distribute the heat across the skull while delivering the appropriate amount to the focal point. Another modification has been the addition of a cold water cap placed between the patient’s head and transducer to aid in the cooling of the scalp and skull ( Fig. 1 ).
Although using FUS alone is effective, it is often nonspecific and can lead to tissue ablation of both normal and neoplastic cells. This necessitates the use of a spatial and temporal monitoring modality, which is where MRI comes in. One of the components of MRI is its thermometry capability. MR thermal mapping can generate near real-time temperature-sensitive maps that help monitor and maintain appropriate temperature levels during focused ultrasound and focus on the region of interest while preventing any damaging heating of adjacent or trajectorial tissue. Additionally, an advantage of MRgFUS over radiotherapy is the lack of ionizing radiation and the capacity to administer repeated treatments within a short amount of time, with minimal side effects. Unlike surgery, MRgFUS is not invasive and is a bloodless surgery. Although approved in the treatment of uterine fibroids, it is also currently being tested in the brain, breast, liver, and prostate malignancies. More recently, it has been approved for treatment of essential tremor.
Thermal Ablation in Brain Tumors
The use of ultrasound in the generation of heat to cause tissue ablation has been thought of as a promising technique since the early 1940s. In an initial clinical study investigating this technology in brain malignancies, Ram and colleagues (2006) used MRgFUS for 3 subjects with recurrent glioblastoma. For this study, a craniectomy was done in advance on all subjects to provide a window for the ultrasound treatment. Over the course of the study, 1 subject suffered a secondary lesion, which was thought to be caused by a reflection of the ultrasound beam, which prompted the addition of several safety measures to the procedure. All 3 of the subjects had relatively long-term survival after treatment, at the time 33, 38, and 10 months. Histologic analysis showed that there was a defined demarcation of the treated region compared with surrounding tissue. This supports a strength of this therapy, which is its precision and specificity to targeted regions without affecting adjacent regions. Overall, although this study had a major drawback in its invasiveness and the development of a secondary lesion, the survival times helped provide initial evidence of the potential feasibility of this therapy in brain malignancies.
With the development of phased array design, active cooling, and lower frequency, the group at Brigham and Women’s Hospital in Boston became the first to use transcranial ultrasound to ablate tumors. In this seminal phase I study, the investigators were able to treat 3 subjects with glioblastoma thought to be otherwise unresectable. In 2010, McDannold and colleagues, at Brigham and Women’s Hospital published the study on use of transcranial MRgFUS, with no removal of the skull, in contrast to the Ram and colleagues (2006) study. The treatment was performed in an MRI using continuous MR thermometry sequences to assess the tissue temperature. During sonication, the images verified the location of the heat in the tumor, as well as the temperature. The lesions were treated to achieve a temperature of 55°C or 43°C at 240 equivalent minutes. Unfortunately, the peak temperature achieved was 42°C, 51°C, and 48°C for the 3 subjects, which was not high enough to cause tissue ablation. This study was innovative in its noninvasive nature. It evaluated the feasibility of MRgFUS on glioblastoma through MR temperature monitoring and focusing of the ultrasound beam through the intact skull while accounting for aberrations and attenuation of the beam. The success of the technique, however, was in the ability to accurately target brain tissue of interest, cause no significant heating or burn injury to the scalp, and to cause no injury to surrounding brain tissue. The investigators postulated that longer sonication times, which were unable to be performed due to the long length of treatment time and subject discomfort, would cause higher tissue temperature.
A subsequent case study by Coluccia and colleagues achieved the first successful thermal ablation of a brain tumor using transcranial MRgFUS. In a phase 1 clinical trial of a subject with a centrally located glioblastoma, sonications were administered into the target region with increasing energy until MR thermometry and thermal dose maps predicted ablation of significant tumor volume. The subject was evaluated with neurologic examinations and MRI after the procedure, and at 1, 5, and 21 days after the procedure, to assess any side effects. No new neurologic deficits were displayed and the subject was mobile after the procedure. MRIs were obtained at several points after the procedure, with images at 30 minutes after the sonications and at 21 days after the procedure displaying unchanged areas of ablated tumor tissue and no sign of tumor progression ( Fig. 2 ). Although these studies demonstrated the potential feasibility of this treatment in brain tumors, further research will need to be conducted to optimize safety considerations and overcome limitations in tumors located in noncentral areas in the brain.
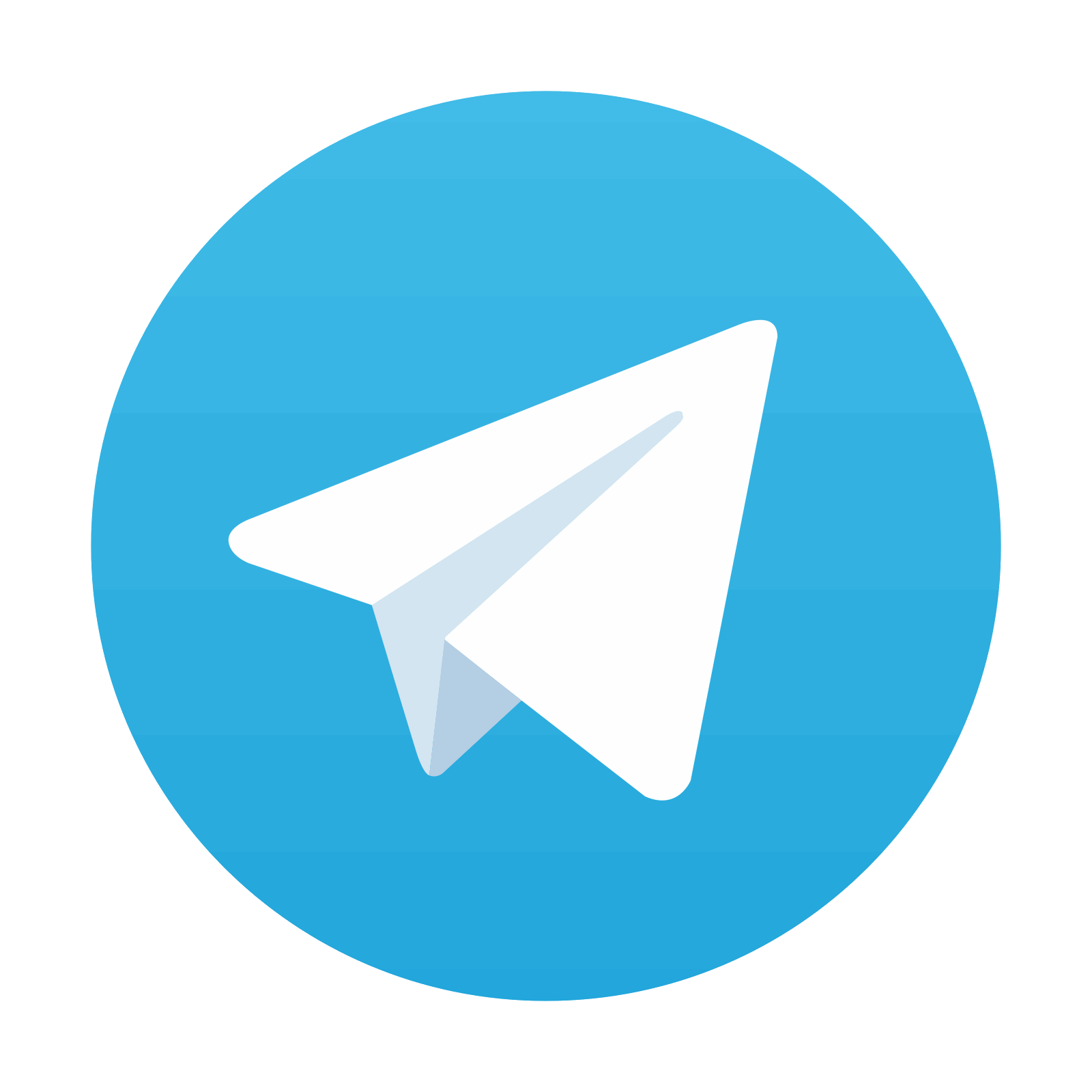
Stay updated, free articles. Join our Telegram channel

Full access? Get Clinical Tree
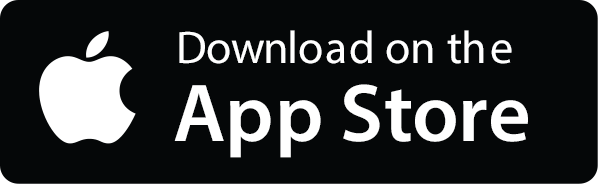
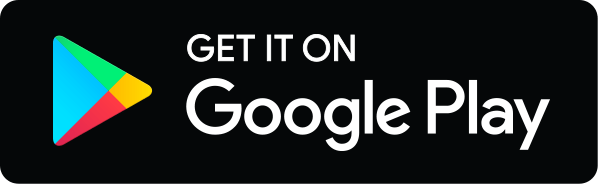
