Leksell stereotactic frame being applied.
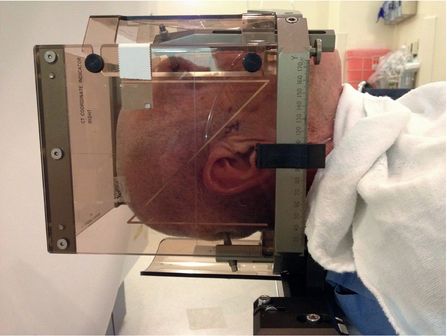
Patient on CT gantry with localizer box containing fiducial markers.
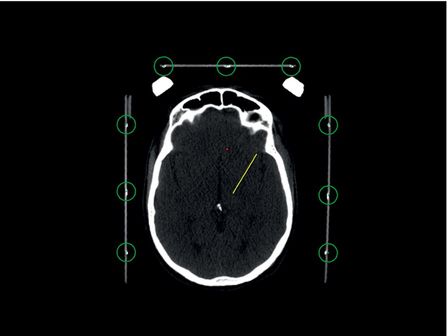
CT head showing localizer box and fiducial markers; fiducials are seen inside the green circles.
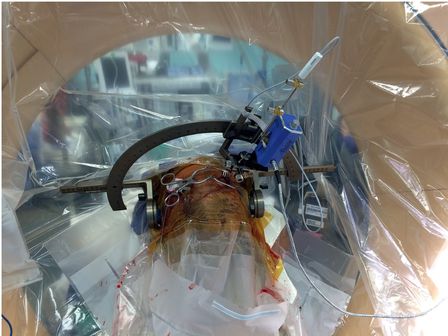
Intraoperative view of a Leksell arc and micropositioner.
These surgical platforms, although well established and highly accurate when tested under controlled laboratory conditions, have a real-world application accuracy of somewhere between 1 and 3 mm.4 As the efficacy of DBS is highly dependent on consistent, proper placement of the DBS lead, a high degree of both precision and accuracy is desirable. For this reason, and to ensure placement in the motor subterritory of the various nuclei implanted for movement disorders, the majority of centers use some form of physiological mapping to define the borders of the target and refine the preoperative targeting before placing the lead.
Microelectrode recording (MER) is perhaps the most common method used for mapping the borders of the target. MER takes advantage of the fact that white matter regions of the brain are relatively silent, while gray matter areas (nuclei) are relatively active and have their own characteristic pattern of spontaneous motor activity. Moreover, the motor subterritories of nuclei such as the subthalamic nucleus (STN), globus pallidus internus (GPi), and ventral intermediate nucleus of the thalamus (Vim) have cells with activity that is modulated by contralateral limb movement.
Although many consider MER to be the gold standard for mapping the nuclear borders, other techniques have been used, including recording of local field potentials.6 Finally, most centers use macrostimulation during final target confirmation, either through a larger electrode or the implanted DBS lead, to look for efficacy (such as reduction in tremor or rigidity) and determine thresholds for stimulation-induced adverse effects from activation of structures adjacent to the target.
Preoperative imaging
Physiologically guided stereotactic surgery is dependent on preoperative imaging for target selection and planning of the entry point and trajectory. MRI is preferred because it provides superior tissue discrimination and differentiation between gray matter nuclei and white matter. However, all MRI images have some degree of inherent distortion. CT has relatively poor tissue discrimination, but has no image distortion; it therefore provides very accurate visualization of the fiducial markers. Most centers use a combination of MRI and CT to take full advantage of each modality’s strengths. An MRI is usually obtained as an outpatient prior to the day of surgery. This typically includes a volumetric, whole-brain T1 sequence with gadolinium to visualize vascular anatomy in the region of the cortical entry point, along the trajectory, and at the target itself. In the case of STN and GPi, sequences such as T2 or inversion recovery are also obtained to provide direct visualization of the nuclei for targeting (Figure 4.2). The Vim is not discernible from the surrounding thalamic nuclei on 1.5T and 3T MRI, so for Vim the targeting is performed by taking measurements relative to the mid-commissural point.
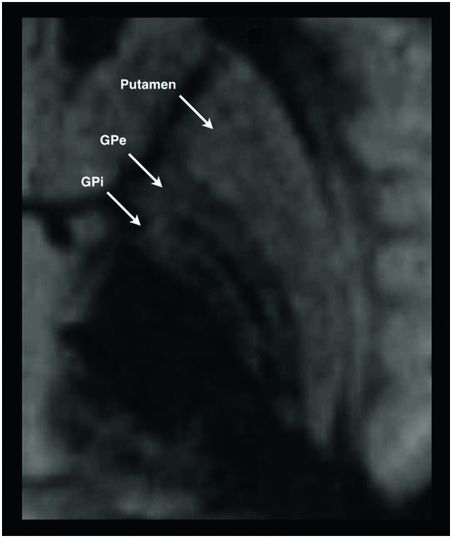
Fast gray matter acquisition T1 inversion recovery MRI sequence showing the globus pallidus internus and surrounding structures. Target selection based on direct visualization of the nucleus is performed on sequences such as this.
These MRI sequences are transferred to a surgical planning workstation running specialized stereotactic software that allows the implanting team to perform targeting and trajectory planning at their convenience. On the day of surgery, a CT scan is typically obtained after frame/fiducial placement, and the stereotactic software is used to fuse the CT and MRI images. When the image fusion paradigms work well, this is a powerful technique that provides the surgeon with both the accuracy of fiducial localization provided by CT and the superior tissue discrimination of MRI. However, image fusion between two different modalities is not always accurate, and this introduces a source of potential error to the implantation process. Fiducial registration (and the 3D stereotactic spatial map) is established by CT, but target selection is performed on MRI; in fact, the target itself is not even visible on CT. The accuracy of image fusion between the two modalities can only be assessed by comparison of high-signal contrast regions, such as the ventricles or cortical surface, between the two volumes. If the surgeon is not satisfied with the automatic fusion process performed by the software, he or she can perform a manual fusion by viewing the CT and MRI side-by-side and selecting common points on the images. However, this cannot eliminate the possibility of error that is inherent in the fusion process.
Challenges with awake surgery
The newest techniques currently being pursued for DBS lead implantation seek to move the implantation procedure from an awake, physiologically guided process to a purely image-guided process.
Although awake surgery is a successful and reliable means of lead implantation, it does have drawbacks. First, it is a technically demanding and time-consuming process. Physiological mapping, particularly MER, is reliant on a team that possesses knowledge of single-cell neuronal physiology in the various basal ganglia targets, and on delicate recording equipment that is sensitive to the multiple sources of electronic noise that is present in operating rooms. Mapping a single target can take anywhere from 30 minutes to several hours. Second, the process of mapping almost always involves multiple penetrations of the brain. In the case of MER, this may consist of as few as two penetrations: one with a microelectrode, and if the map is satisfactory, a second penetration to place the DBS lead. However, if the “Ben Gun” recording technique is used, there may be up to five simultaneous microelectrode penetrations, followed by another to place the lead. Some studies have shown that increasing numbers of brain penetrations may increase the risk of bleeding.
Third, the procedure requires that patients be able to tolerate being awake for upwards of an hour or several hours, and be able to cooperate and communicate effectively with the implanting team. For Parkinson’s disease patients, this also means being able to tolerate being off medication in order for motor signs to be evident during surgery. The procedure is difficult for some patients to tolerate, and even impractical in some cases of cervical or pediatric dystonia. In our practice, there are patients who are clearly good motoric candidates for DBS but decide not to undergo awake surgery due to fear of the operation itself. Fourth, one of the historical goals of awake surgery is to be able to detect intracranial complications such as intracranial hemorrhage or improper lead placement. However, hemorrhages are often not immediately detected by examination in the operating room, and returns to the operating room for lead repositioning are still clearly needed despite awake surgery.7–9
Another shortcoming of awake surgery is perhaps the least apparent but one of the most important. Traditional stereotactic surgery involves targeting that is performed on images obtained preoperatively. These “historical” images do not reflect the actual state of the brain in the operating room.
Creation of the burr holes and opening of the dura causes CSF loss and pneumocephalus, both of which lead to brain shift within the cranium. Although it has been known for some time that shift occurs at the cortical surface, there has been a long-standing debate whether brain shift actually occurs in the deep structures that are the targets of DBS surgery. There are now well-designed studies that show that deep structures can indeed shift well over 2 mm in some instances; moreover, the direction of shift is unpredictable and is not always in the direction of gravity, as would be expected.10,11 Any brain shift, whether superficial or deep, is relevant because it not only impacts the location of the target, but also alters the structures encountered along the intended trajectory. Brain shift, like CT–MRI fusion, is another source of potential error in traditional stereotactic surgery, and another source of error that can potentially be overcome by awake mapping. If a purely image-guided technique for lead implantation is to be successful, it needs to be able to overcome errors related to brain shift and image fusion.
Finally, a shortcoming of all stereotactic surgery is something that can be referred to as “human factor issues”; that is, inaccuracy that occurs as a result of human error. It is not unusual for surgeons to transpose numbers when writing down stereotactic coordinates, set up a stereotactic frame incorrectly, or make a mistake when measuring a distance on one of the devices or the lead itself. DBS surgery occurs in a busy operating room with multiple sources of distraction that can lead to human errors, even at the best centers. This, along with some of the other factors discussed above, is why many stereotactic devices demonstrate a high degree of accuracy when tested under controlled laboratory settings but have less accuracy when assessed in real-world clinical settings; the latter is referred to as the application accuracy of a device. While some may argue that an error of 2 mm is not relevant, in DBS there is good evidence that this degree of accuracy is important for clinical outcomes.9,12
Intraoperative imaging
Intraoperative imaging with fluoroscopy, CT, and even MRI in conjunction with awake surgery has been performed for many years, and it is used by many groups to try to detect errors. However, despite descriptions to the contrary, this is not true image-guided surgery; rather, it is awake, physiologically guided surgery that uses imaging to “check” lead placement immediately after implantation. Fluoroscopy only provides information about lead position relative to the stereotactic frame; it provides no information about the position of the lead in the brain itself. Intraoperative CT must be fused with the preoperative MRI in an attempt to determine lead position relative to the intended target; this process is prone to error occurring as a result of image fusion and brain shift. Intraoperative MRI is often done with an open (and frequently low field strength) scanner, which compromises visualization of the target. Moreover, it usually requires that the lead be completely removed from the micropositioner prior to imaging, as most micropositioners are not MRI-compatible. If the lead position is found to be unsatisfactory, it must be detached, the micropositioner apparatus re-mounted, and awake lead placement repeated. None of these techniques circumvents the other challenges of awake surgery; in fact, in some cases they increase the complexity of the procedure rather than simplifying it.
Image-guided DBS surgery
Two different methods of image-guided DBS lead placement have evolved; one focusing on MRI as the imaging modality, the other on CT. Each has their own advantages and disadvantages, but together they represent the latest and most radical technical developments in DBS lead implantation.
At their core is the philosophy that purely image-guided placement is appropriate for targets that are (1) visible on MR imaging, and (2) “mature.” A mature target is one that has an extensive history of studies that correlate anatomical lead locations with positive clinical outcomes. When a target such as the STN or GPi reaches a point where the awake, MER-guided literature repeatedly demonstrates that lead locations in a predictable anatomical region result in good clinical outcomes, that target becomes appropriate for purely anatomical lead placement; that is, placement that does not use physiological mapping of any kind. Such a technique would no longer require that the patient be awake during surgery, which is why these techniques have recently been referred to collectively as “asleep DBS.”
MRI-guided DBS implantation
Our center was the first to propose and develop a purely image-guided approach to DBS implantation based on the concept of MRI-visible, mature targets. The original intention was to demonstrate that anatomical lead placement without physiological confirmation was feasible and would result in accuracy and clinical outcomes that were comparable to awake surgery. The first-generation technique was relatively crude and relied on pre-existing skull-mounted aiming devices and the native MRI scanner software to guide the procedure. Although successful, the technique had hardware and software limitations that left much of the accuracy in the hands of the surgeon and imaging team.13 Despite this, the technique demonstrated an average application accuracy of 1.2 mm ± 0.65 mm, with 87% of DBS leads placed with a single brain penetration.14 A second-generation system (ClearPoint, MRI Interventions, Irvine, CA), comprised of a novel skull-mounted aiming system and a software environment that interactively guides the MR operator and surgeon though the procedure, was approved by the US Food and Drug Administration in 2010 and addressed many of the shortcomings of the first-generation technique.15 It also made the procedure more user-friendly for the MRI operator, making it practical for use at a wide variety of centers.
The procedure takes place in a 1.5T or 3T MRI scanner. The MRI does not have to be specialized or located in an operating room; the procedure was intended to be performed in any standard MR scanner located in the radiology department or elsewhere, provided space is adequate to accommodate a surgical team and anesthesia. The patient is placed under general anesthesia and positioned on the MRI gantry with the head placed in a combination MR coil/head holder. There is no stereotactic head frame, and no preoperative imaging, image fusion, or targeting is required. The final target is intentionally not chosen until after the burr holes are made during the procedure itself. This allows any initial pneumocephalus and brain shift to occur prior to targeting. A custom surgical drape attaches to the patient’s head and the MR scanner, creating a mobile sterile field that can accommodate movement of the patient in and out of the scanner bore (Figure 4.3). MR images are streamed to a laptop running the ClearPoint software, which guides the surgeon and the MR operator through the implantation procedure. A preliminary target and trajectory are selected, and the software indicates the proper place on the scalp and skull to make the incision and burr holes. Once the burr holes are created, the skull mounted aiming devices (SmartFrames, MRI Interventions, Irvine, CA) are screwed on to the skull (Figure 4.4). The aiming devices have four degrees of freedom: two angular movements at 90 degrees to each other (pitch and roll), and two linear side-to-side and front-to-back movements (X and Y).
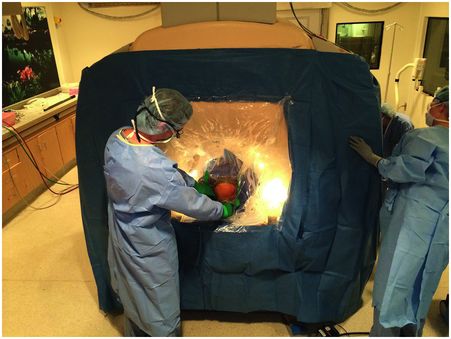
Sterile surgical drape for MRI-guided DBS. The drape attaches to the top of the patient’s head and the face of the scanner. An accordion-like center section (clear portion of the drape) allows movement of the patient in and out of the bore.
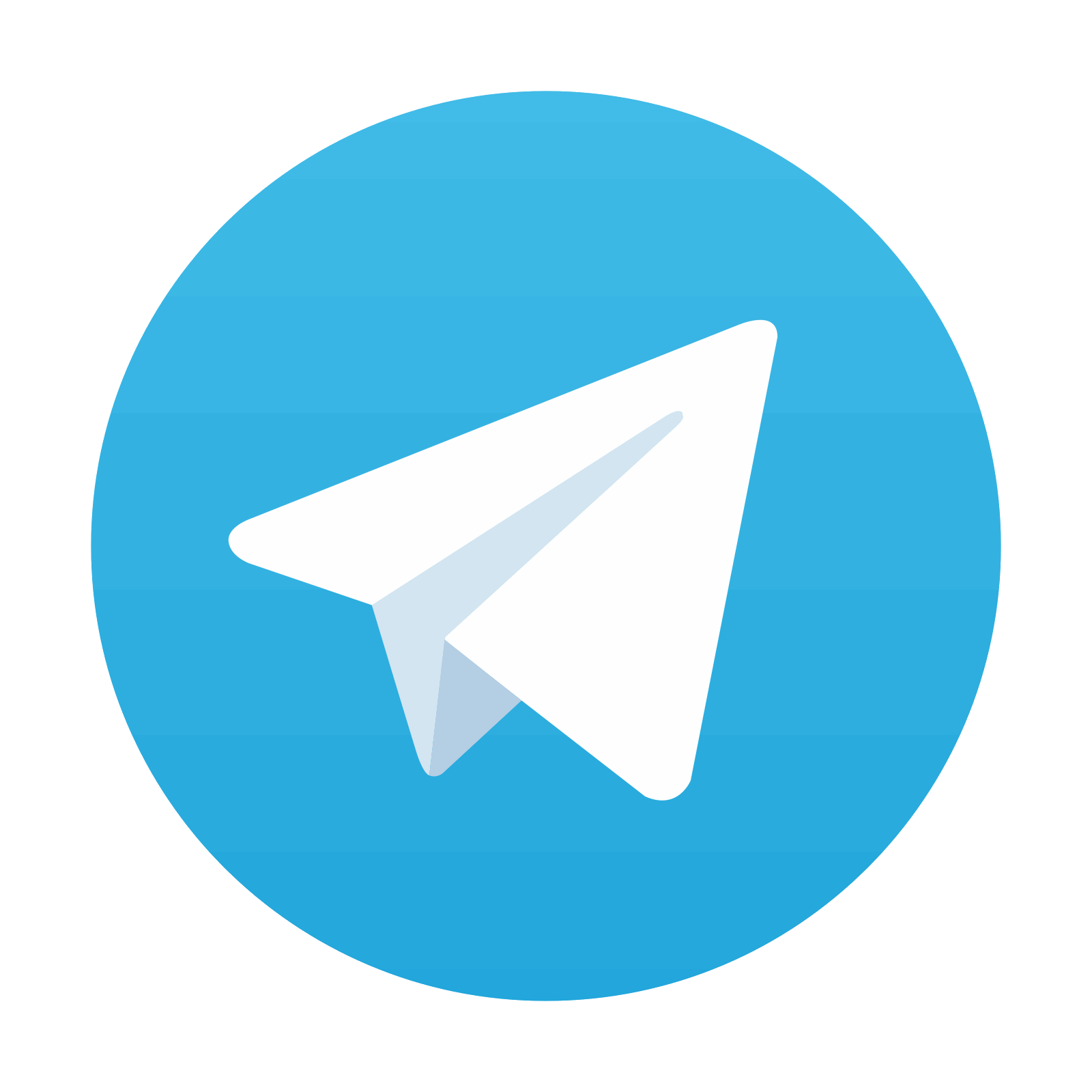
Stay updated, free articles. Join our Telegram channel

Full access? Get Clinical Tree
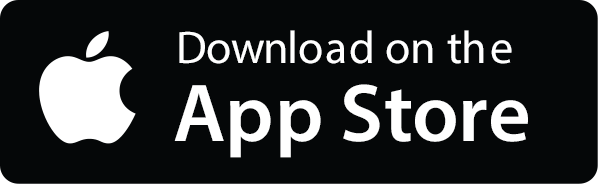
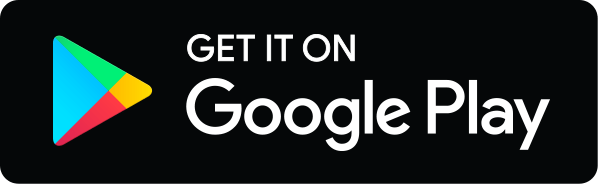