The brain vascular system develops in such a way that it continuously adapts the supply of oxygen and other nutrients to the needs of the parenchyma. To accompany the developing brain vesicles, it evolves in several steps: superficial meningeal network first; intraventricular choroid plexuses which determine the arterial pattern; penetrating capillaries from the surface to the ventricular germinal matrix forming simple transcerebral arteriovenous loops; cortical capillaries last, mainly in the last trimester. The venous return becomes connected to both the surface and to the choroidal veins, so forming distinct meningeal and subependymal venous drainage systems, while the arteries are on the surface only. While the arterial system was determined early (week 8), the venous system is continuously remodeled by the morphological changes of the base of the skull and the expansion of the brain vesicles. Until late in gestation, the vascular system is made of simple endothelial channels in which the arterial or venous fate is determined primarily by the direction of flow.
The development of the blood-vessels of the head demonstrate[s] the embryologic principle of what may be termed integrative development. [The vascular apparatus] reacts continuously in a most sensitive way to the factors of its environment, the pattern in the adult being the result of the sum of the environmental influences that have played upon it throughout the embryonic period. […] This apparatus is continuously adequate and complete for the structures as they exist at any particular stage; as the environmental structures progressively change, the vascular apparatus also changes and thereby is always adapted to the newer condition. […] For each stage it is an efficient and complete going-mechanism, apparently uninfluenced by the nature of its subsequent morphology. George L. Streeter, 1918
Oxygen cannot diffuse beyond 150 to 200 μm in a living tissue at 37°C. As a consequence, the vascular system develops in such a way that it continuously adapts the supply of oxygen and other nutrients to the needs and the morphology of the evolving brain. Schematically, 4 overlapping consecutive steps can be described.
- 1.
Initially (weeks 2–4) the exposed neural plate and groove and the open neural tube are simply fed by diffusion from the amniotic fluid ( Fig. 1 ).
Fig. 1
Week 4. The neural tube is not closed yet, and the neural plate (pn) receives oxygen and nutrients from the surrounding amniotic fluid (amn) ( A ). Endothelial vasculogenesis is building up the cardiovascular organ system that already extends toward the cephalic extremity of the embryo ( B ).
- 2.
After closure (week 4) the neural tube is surrounded by a dense connective tissue, the meninx primitiva (weeks 5–8) (for review, see Ref. ). This meninx primitiva contains primitive vascular loops (meningeal meshwork) developed by vasculogenesis from the primitive dorsal aorta and cardinal veins, and through them connected with the primordial vascular organ initially developed over the yolk sac ( Fig. 2 ).
Fig. 2
Week 5. The neural tube is embedded into a dense connective tissue, the meninx primitiva (mp) ( A, B ) that contains vascular loops ( B ) connected to the dorsal aortae and cardinal veins. They are forming the primordial brain vascular meshwork, from which oxygen and nutrients diffuse to the neural tissue.
- 3.
As the cephalic portion of the neural tube grows and expands to form the 3 primary brain vesicles (rhombencephalic, mesencephalic, and prosencephalic vesicles), the meninx primitiva further evolves and to better supply the neural tissue invaginates into the roofs of the prosencephalic and rhombencephalic vesicles, forming the primordia of the choroid plexuses (weeks 5–7). At this stage, diffusion of nutrients to the neural tissue is both peripheral from the meninx primitiva and ventricular from the developing choroid plexuses. From the point of view of the morphogenesis of the cerebral vasculature, this plexular differentiation is crucial: it leads to the early differentiation of specific choroid feeders within the meningeal vascular meshwork from which all brain arteries eventually evolve (for review, see Ref. ): from that stage, the final arterial pattern is already recognizable. By contrast, the venous outflow that is specifically adapted to the choroid stage is only transitory and the veins will continue to adapt passively to local circulatory factors until after birth, even though the gross venous pattern can be recognized at the end of the first trimester.
- 4.
When the neural tube becomes too thick to be nourished by extrinsic diffusion alone, intrinsic capillaries develop by sprouting (angiogenesis) from the vessels of the meninx primitiva covering the brain surface. According to the same principles, they will supply the most demanding areas: first the ventricular-subventricular proliferative germinal zone already at the second month, then much later in the last trimester the developing cortex when it becomes widely connected and functional. Together with the development of the early intrinsic vasculature, the meninx primitiva undergoes progressive changes resulting in the fluid-filled leptomeninges and the dense dural coverings and reflections, as well as the opening of the fourth ventricular outlets.
The primitive perineural vascular meshwork
Shortly after its closure (week 4), the neural tube becomes embedded in a solid mesenchyme that forms the meninx primitiva (see Fig. 2 ). This meninx primitiva of the forebrain (anterior neural plate) derives from the neural crest of the more caudal posterior diencephalic and mesencephalic segments. The meninx primitiva of the developing spinal cord, hindbrain, midbrain, and posterior diencephalon derives from the somitic mesoderm.
Vasculogenesis produces the vascular organ system and the first blood cells; it extends to the meninx primitiva and forms the first arteriovenous loops .
The vascular system as an organ system derives from a differentiation of lateral and posterior mesodermal cells that migrate toward the yolk sac and form blood islands or hemangioblastic aggregates. These aggregates differentiate into peripheral endothelial cells and central hematopoietic cells. Endothelial cells form vascular cords that canalize and become interconnected in a plexular network that extends into the embryo, building the cardiovascular system, the first organ system of the embryo. This process is under the control of, among others, the vascular endothelial growth factor VEGF and its receptor VEGFR2. Vasculogenesis proceeds cranially and invades the meninx primitiva to form a vascular meshwork around the primitive cephalic central nervous system. The vascular lumen forms by vacuolization of the endothelial cords (a truly intracellular lumen). These primordial vessels connect together to form an indistinct meshwork without clear preferential channels (hence its name of primary head plexus ) and it is impossible at the beginning to differentiate between arteries and veins. This early process was not studied in human embryos but in chick embryos between the stages of 9 and 16 somites; this would correspond to day 28 in human, that is, just before and at the time of the closure of the neuropores (for developmental staging, see Ref. ).
Over the next day or so, an active proliferation of endothelial channels takes place between the cranial ectoderm and the neural surface. This proliferation starts early around the forebrain and the midbrain, and later around the hindbrain; it also proceeds from the ventral aspect of the brain vesicles to its dorsal aspect. At the same time the meninx primitiva begins to undergo the dramatic changes that will result in the formation of the calvarium, dura, and fluid-filled leptomeninges, to the development of the choroid plexuses and to the opening of the fourth ventricular outlets. The perineural vascular meshwork follows this meningeal reorganization. The deeper vascular endothelium that covers the wall of the neural tube flattens and takes the appearance of a capillary network. The superficial vascular layer takes the appearance of larger and more continuous channels that form clear connections with the paired dorsal aorta and cardinal veins, and will eventually become the major brain arteries and veins. Between these deep and superficial vascular layers, a few communications persist and become the branches of the arteries and the tributaries of the veins : arterial supply and venous drainage channels become selected from this initial meningeal meshwork as they respond to the evolving and species-specific needs and morphology of the developing brain.
The morphogenetic alterations that result in the adult pattern of brain arteries has been described in great detail by Padget, while the morphogenesis of the veins has been mostly described by both Streeter and Padget.
Morphogenesis of the brain arteries
Padget studied the development of the cerebral arteries by using a method of graphic reconstruction from 22 sectioned embryos of the Carnegie Collection ranging in age from 24 to 52 days (4–43 mm). Her outstanding contribution therefore rests on a relatively limited number of specimens. Based on the evolution of the cardiovascular system, especially the aortic and pulmonary arches, Padget identified, defined, and illustrated 7 steps or stages in the development of the brain arteries, from an early undifferentiated pattern to the essentially adult pattern.
At stage 1, the primitive carotid artery supplies the forebrain as well as the hindbrain through the transient carotid-vertebrobasilar connections (4–5 mm, 28–29-day embryo) .
The internal carotid artery (ICA) can already be recognized at this stage. The ICA supplies the 3 forebrain, midbrain, and hindbrain vesicles. Rostrally when reaching the forebrain, it divides into an anterior olfactory branch (future anterior cerebral artery, ACA) that passes dorsal to the optic vesicle, and a posterior branch that resolves into a plexus around the midbrain without reaching the hindbrain. The ICA also connects with the contralateral ICA behind the Rathke pouch, so forming the posterior segment of the future circle of Willis.
More proximally, the hindbrain instead is fed by 3 presegmental and 1 intersegmental arterial channels. Two originate from the proximal ICA: the trigeminal artery at the level of the trigeminal ganglion, and the otic artery at the level of the otic vesicle. Two originate from the paired dorsal aorta: the hypoglossal artery along the hypoglossal nerve, and the proatlantal artery (first intersegmental cervical C1 artery) along the first cervical nerve ( Fig. 3 ). These trunks supply the paired ventral bilateral longitudinal neural arteries that feed the hindbrain on either side at this stage ( Fig. 4 A). The trunks eventually regress, after the bilateral longitudinal neural arteries have fused on the midline, and connected cranially with the caudal division of the ICA and caudally with the longitudinal paravertebral anastomosis that will become the vertebral artery. The channels actually exist for a very short time of 4, at most 8 days (for the trigeminal and proatlantal arteries) before vanishing at about stage 3. Uncommonly they may persist and be functional as anatomic variants/malformations in clinical settings.
At stage 2 the posterior communicating artery forms ( 5–6 mm, 29-day embryo) .
The caudal divisions of the ICAs extend caudally and join the bilateral longitudinal neural arteries to form the true posterior communicating arteries (PCOMs). Consequently, the trigeminal arteries are dwindling at their carotid origin, as well as the hypoglossal arteries. The longitudinal neural arteries tend to unite along the midline to form the basilar artery (BA) (see Fig. 4 B). At this stage they still remain largely dependent on the proatlantal (first intersegmental) arteries for their caudal supply.
At stage 3 the forebrain arteries can be recognized; the basilar and vertebral arteries are completed ( 7–12 mm, 32 days) .
Within the primitive meshwork, the trunk of the ACA develops rostrally around the neck of the growing hemispheric vesicle, and the early stem of the future middle cerebral artery (MCA) extends laterally from it (see Fig. 5 ). Behind the neck of the growing hemisphere, the primitive anterior choroidal artery (ACHA) courses toward the diencephalon; it is now the largest branch of the ICA. The ICA also provides a primitive dorsal ophthalmic artery. Caudally, from the caudal end of the PCOM 2 dorsal branches emerge, 1 posterior choroidal artery (PCHA) toward the diencephalon and 1 mesencephalic artery toward the midbrain ( Fig. 6 A). The BA has further evolved by midline fusion of the longitudinal neural arteries; the trigeminal artery may still be found at this stage, but it is usually interrupted already. The vertebral artery (VA) is now forming as a longitudinal paravertebral anastomosis between the intersegmental cervical arteries from C7 to C1 ; (see also Fig. 1 in Ref. ). Over the rhombencephalon the anterior superior cerebellar artery (ASCA) becomes distinguishable.
At stage 4 the mature pattern becomes apparent ( 12–14 mm, 35 days) .
The anterior division of the ICA now provides clearly recognizable although still plexiform branches: the ACA with a medial branch that approaches the midline to form the anterior communicating artery (ACOM), the early MCA, dorsal and ventral primitive ophthalmic branches, the prominent ACHA, as well as the PCHA and the mesencephalic arteries. The BA and the VA show further development, and early rhombencephalic branches can be recognized.
At stage 5, which can be called the choroid stage, the adult pattern has become obvious ( 16–18 mm, 40-day embryo) .
Klosovskii stressed the role the choroid plexuses play in supporting the early brain tissue. During weeks 5 to 7, these projections of the meninx primitiva develop into the fourth, third, and lateral ventricles ( Fig. 5 A, B). Because they become extremely active from a metabolic point of view, they induce a clear selection of their arterial feeders: the ACA anteriorly, the ACHA inferiorly, and the PCHA posteriorly (see Fig. 5 C). (Although the adult ACA is separated from the tela choroidea by the callosal and hippocampal commissures, it is originally a choroidal artery. Its subfornical branch still reaches the foramen of Monro in the human fetus, the adult chimpanzee, and in injected adult human anatomic specimens [personal data]). The MCA grows as a lateral branch, primarily striatal at this stage, of the proximal ACA. The mesencephalic artery also is now prominent and forms a rich plexiform network over the tectal plate. This specific pattern at this stage—choroidal arteries including the ACA, and mesencephalic arteries—corresponds to the anatomy of the arterial feeders of the vein of Galen arteriovenous malformations.
At stages 6 (20–24 mm, 44 days) and 7 (40 mm, 52 days), the mature pattern is completed with the circle of Willis and the capture by the posterior hemispheres of the vertebrobasilar blood supply .
A completed, still plexiform ACOM sends a branch to the anterior corpus callosum. A prominent perforant (intracerebral) artery, the recurrent artery of Heubner is already apparent as well, coursing from the ACA toward the medial striatum. The MCA, striatal also at this stage, is fully developed. Dorsal branches of the mesencephalic arteries have extended across the dorsal meninges to the inferior and medial-posterior portion of the now significantly expanded cerebral hemispheres, forming the cortical territory of the posterior cerebral artery (PCA) at the expense of the ACHA ( Fig. 6 ). In addition, this new mesencephalic-PCA territory typically is not supplied by the ICA any more but via the VAs and BA instead: the now huge cerebral hemispheres have captured part of the vertebrobasilar blood supply. Besides the ASCA, the anterior inferior and posterior inferior cerebellar arteries (AICA and PICA) have become more apparent in the plexus that still covers the caudal hindbrain ( Fig. 6 ).
In summary, the arterial system of the brain evolves within and from an initially undifferentiated vascular meshwork, always in a precisely adapted response to the evolving metabolic requirements of the expanding neural tissue. Initially, the ICA feeds the fore- and the midbrain through its anterior and posterior terminal divisions, while the hindbrain is fed by presegmental/intersegmental branches from the ICA and the paired aorta: these transient branches regress with the development of the final PCOM and vertebral arteries. Over the brain surface, specific arterial channels differentiate, which at the early stages correspond to the growing zone (neck) of the cerebral hemispheric vesicles and to the differentiation of the choroid plexuses: the ACA anteriorly, the ACHA posteriorly; together with the PCHA, they later supply the prosencephalic tela choroidea and its glycogen-loaded plexular formations. The MCA emerges as a lateral branch of the ACA, together with the other nonchoroidal parenchymal branches of the ACA, ACHA, and (captured) PCA when the intrinsic intraparenchymal vasculature develops at the late embryonic and early fetal period. In a different way because of different local conditions, the vasculature of the midbrain and hindbrain evolve according to the same hemodynamic (ie, metabolic) rules. The conditions in which the initial meshwork consolidates into discrete arteries explain most of the many variants and morphologic abnormalities that can be observed in later life. As the metabolically defined territories are species-specific and essentially constant, distal arterial distribution is fairly constant and most variations are found in the proximal segments of the feeders, where different hemodynamic “solutions” may become selected in the initial meshwork (resulting in a “variations on a theme” anatomy).
Morphogenesis of the brain arteries
Padget studied the development of the cerebral arteries by using a method of graphic reconstruction from 22 sectioned embryos of the Carnegie Collection ranging in age from 24 to 52 days (4–43 mm). Her outstanding contribution therefore rests on a relatively limited number of specimens. Based on the evolution of the cardiovascular system, especially the aortic and pulmonary arches, Padget identified, defined, and illustrated 7 steps or stages in the development of the brain arteries, from an early undifferentiated pattern to the essentially adult pattern.
At stage 1, the primitive carotid artery supplies the forebrain as well as the hindbrain through the transient carotid-vertebrobasilar connections (4–5 mm, 28–29-day embryo) .
The internal carotid artery (ICA) can already be recognized at this stage. The ICA supplies the 3 forebrain, midbrain, and hindbrain vesicles. Rostrally when reaching the forebrain, it divides into an anterior olfactory branch (future anterior cerebral artery, ACA) that passes dorsal to the optic vesicle, and a posterior branch that resolves into a plexus around the midbrain without reaching the hindbrain. The ICA also connects with the contralateral ICA behind the Rathke pouch, so forming the posterior segment of the future circle of Willis.
More proximally, the hindbrain instead is fed by 3 presegmental and 1 intersegmental arterial channels. Two originate from the proximal ICA: the trigeminal artery at the level of the trigeminal ganglion, and the otic artery at the level of the otic vesicle. Two originate from the paired dorsal aorta: the hypoglossal artery along the hypoglossal nerve, and the proatlantal artery (first intersegmental cervical C1 artery) along the first cervical nerve ( Fig. 3 ). These trunks supply the paired ventral bilateral longitudinal neural arteries that feed the hindbrain on either side at this stage ( Fig. 4 A). The trunks eventually regress, after the bilateral longitudinal neural arteries have fused on the midline, and connected cranially with the caudal division of the ICA and caudally with the longitudinal paravertebral anastomosis that will become the vertebral artery. The channels actually exist for a very short time of 4, at most 8 days (for the trigeminal and proatlantal arteries) before vanishing at about stage 3. Uncommonly they may persist and be functional as anatomic variants/malformations in clinical settings.
At stage 2 the posterior communicating artery forms ( 5–6 mm, 29-day embryo) .
The caudal divisions of the ICAs extend caudally and join the bilateral longitudinal neural arteries to form the true posterior communicating arteries (PCOMs). Consequently, the trigeminal arteries are dwindling at their carotid origin, as well as the hypoglossal arteries. The longitudinal neural arteries tend to unite along the midline to form the basilar artery (BA) (see Fig. 4 B). At this stage they still remain largely dependent on the proatlantal (first intersegmental) arteries for their caudal supply.
At stage 3 the forebrain arteries can be recognized; the basilar and vertebral arteries are completed ( 7–12 mm, 32 days) .
Within the primitive meshwork, the trunk of the ACA develops rostrally around the neck of the growing hemispheric vesicle, and the early stem of the future middle cerebral artery (MCA) extends laterally from it (see Fig. 5 ). Behind the neck of the growing hemisphere, the primitive anterior choroidal artery (ACHA) courses toward the diencephalon; it is now the largest branch of the ICA. The ICA also provides a primitive dorsal ophthalmic artery. Caudally, from the caudal end of the PCOM 2 dorsal branches emerge, 1 posterior choroidal artery (PCHA) toward the diencephalon and 1 mesencephalic artery toward the midbrain ( Fig. 6 A). The BA has further evolved by midline fusion of the longitudinal neural arteries; the trigeminal artery may still be found at this stage, but it is usually interrupted already. The vertebral artery (VA) is now forming as a longitudinal paravertebral anastomosis between the intersegmental cervical arteries from C7 to C1 ; (see also Fig. 1 in Ref. ). Over the rhombencephalon the anterior superior cerebellar artery (ASCA) becomes distinguishable.
At stage 4 the mature pattern becomes apparent ( 12–14 mm, 35 days) .
The anterior division of the ICA now provides clearly recognizable although still plexiform branches: the ACA with a medial branch that approaches the midline to form the anterior communicating artery (ACOM), the early MCA, dorsal and ventral primitive ophthalmic branches, the prominent ACHA, as well as the PCHA and the mesencephalic arteries. The BA and the VA show further development, and early rhombencephalic branches can be recognized.
At stage 5, which can be called the choroid stage, the adult pattern has become obvious ( 16–18 mm, 40-day embryo) .
Klosovskii stressed the role the choroid plexuses play in supporting the early brain tissue. During weeks 5 to 7, these projections of the meninx primitiva develop into the fourth, third, and lateral ventricles ( Fig. 5 A, B). Because they become extremely active from a metabolic point of view, they induce a clear selection of their arterial feeders: the ACA anteriorly, the ACHA inferiorly, and the PCHA posteriorly (see Fig. 5 C). (Although the adult ACA is separated from the tela choroidea by the callosal and hippocampal commissures, it is originally a choroidal artery. Its subfornical branch still reaches the foramen of Monro in the human fetus, the adult chimpanzee, and in injected adult human anatomic specimens [personal data]). The MCA grows as a lateral branch, primarily striatal at this stage, of the proximal ACA. The mesencephalic artery also is now prominent and forms a rich plexiform network over the tectal plate. This specific pattern at this stage—choroidal arteries including the ACA, and mesencephalic arteries—corresponds to the anatomy of the arterial feeders of the vein of Galen arteriovenous malformations.
At stages 6 (20–24 mm, 44 days) and 7 (40 mm, 52 days), the mature pattern is completed with the circle of Willis and the capture by the posterior hemispheres of the vertebrobasilar blood supply .
A completed, still plexiform ACOM sends a branch to the anterior corpus callosum. A prominent perforant (intracerebral) artery, the recurrent artery of Heubner is already apparent as well, coursing from the ACA toward the medial striatum. The MCA, striatal also at this stage, is fully developed. Dorsal branches of the mesencephalic arteries have extended across the dorsal meninges to the inferior and medial-posterior portion of the now significantly expanded cerebral hemispheres, forming the cortical territory of the posterior cerebral artery (PCA) at the expense of the ACHA ( Fig. 6 ). In addition, this new mesencephalic-PCA territory typically is not supplied by the ICA any more but via the VAs and BA instead: the now huge cerebral hemispheres have captured part of the vertebrobasilar blood supply. Besides the ASCA, the anterior inferior and posterior inferior cerebellar arteries (AICA and PICA) have become more apparent in the plexus that still covers the caudal hindbrain ( Fig. 6 ).
In summary, the arterial system of the brain evolves within and from an initially undifferentiated vascular meshwork, always in a precisely adapted response to the evolving metabolic requirements of the expanding neural tissue. Initially, the ICA feeds the fore- and the midbrain through its anterior and posterior terminal divisions, while the hindbrain is fed by presegmental/intersegmental branches from the ICA and the paired aorta: these transient branches regress with the development of the final PCOM and vertebral arteries. Over the brain surface, specific arterial channels differentiate, which at the early stages correspond to the growing zone (neck) of the cerebral hemispheric vesicles and to the differentiation of the choroid plexuses: the ACA anteriorly, the ACHA posteriorly; together with the PCHA, they later supply the prosencephalic tela choroidea and its glycogen-loaded plexular formations. The MCA emerges as a lateral branch of the ACA, together with the other nonchoroidal parenchymal branches of the ACA, ACHA, and (captured) PCA when the intrinsic intraparenchymal vasculature develops at the late embryonic and early fetal period. In a different way because of different local conditions, the vasculature of the midbrain and hindbrain evolve according to the same hemodynamic (ie, metabolic) rules. The conditions in which the initial meshwork consolidates into discrete arteries explain most of the many variants and morphologic abnormalities that can be observed in later life. As the metabolically defined territories are species-specific and essentially constant, distal arterial distribution is fairly constant and most variations are found in the proximal segments of the feeders, where different hemodynamic “solutions” may become selected in the initial meshwork (resulting in a “variations on a theme” anatomy).
Angiogenesis: the intrinsic vascularity of the brain
The 2 main steps in the development of the forebrain (using it as a model of the whole brain) are firstly the cellular proliferation (in the germinal matrices or neuroepithelia) and migration, and then the energy-avid intracortical organization. Cellular proliferation and migration mainly occur before week 20, while cerebral connectivity becomes significant in the last trimester of gestation until well after birth. Accordingly, the brain vasculature develops in 2 separate episodes: the first, within the periventricular germinal zone, mostly before week 20, fading progressively toward the end of gestation, and the second in the cortical plate, mostly after week 27.
Two discrete kinds of germinal matrices exist in the forebrain. One overlays the central gray matter (ganglionic eminence), and is designated the striatal neuroepithelium and subventricular zone by Bayer and Altman. In addition to glial cells, it provides mostly GABAergic cells, both to the underlying basal ganglia (local migration) and distantly to the cerebral cortex (tangential migration). The other germinal matrix sits in the depth of the future white matter (cerebral pallium, or mantle), but not under the corpus callosum. Depending on its location it is designated the frontal, parietal, posterior, temporal neuroepithelium and subventricular zone by Bayer and Altman. This matrix provides both the radial glia and the glutamatergic pyramidal neurons to the cortical plate. The germinal matrices attenuate during the last trimester and disappear around term. These matrices remain prominent in the depth of the frontal lobes and over the caudate heads later than in the posterior parts of the hemisphere.
Around the time of its closure, the rostral neural tube is composed of an undifferentiated stratified epithelium surrounding a ventricular lumen. In the 2 following weeks, the development of the ventral ganglionic gray matter (subpallium) antecedes the development of the dorsal cortex (pallium). A laterobasal thickening corresponding to the development of the basal ganglia appears as early as week 6 ; its results from the migration of mostly GABAergic neurons from the ganglionic germinal zone (ganglionic neuroepithelium) to the ventral periphery according to an outside-in process (the peripheral cells are the oldest). At about that time, a preplate made of primitive neurons (it is also called the plexiform layer or the marginal zone) also appears at the periphery of the dorsal pallium ; however, a true cortical plate does not appear until the eighth week. There are 2 types of neuronal migrations to the cortex, radial and tangential. The migration of the excitatory pyramidal neurons from the germinal zone of the pallium (cortical neuroepithelium) develops radially in an inside-out fashion, the older neurons sitting in the deep layers and the younger ones in the superficial layers. Most of the migration of the cortical pyramidal neurons takes place between week 8 and week 17, and is nearly achieved at 20 weeks, but late cortical neurons migrate from the subventricular germinal zone until term time. The migration of the inhibitory cortical interneurons develops tangentially (parallel to the surface of the brain) from the ganglionic neuroepithelium toward their final destination in the cortex.
The long-range connectivity mostly develops after 25 weeks, and keeps increasing until about 2 years after birth. The synaptic activity that goes with it induces a shift from anaerobic to aerobic cortical metabolism, which is supported by a shift of the angiogenetic activity from the (fading) germinal zone to the cortical plate between 20 and 25 weeks. It is also reflected by a corresponding increase in cortical blood flow.
Angiogenesis, both arterial and venous, develops from the surface network .
Angiogenesis is the process by which vessels form by budding from preexisting vessels. In the brain, it proceeds from the surface capillary layer of the leptomeningeal vascular meshwork. These endothelial surface capillaries form buds that approach the external basal lamina and the marginal glia of the cortex, and develop numerous filopodia that penetrate into the brain tissue. A tip cell leads the progression, ahead of a strand of stalk cells that proliferate and form a vascular lumen while pushing the tip cell forward. Adjacent vessels form horizontal connections in the germinal zone so that a flow with incoming (“arterial”) and outgoing (“venous”) blood is constituted ( Fig. 7 A, B). Oxygen concentration obviously is a potent regulator of angiogenesis. From the genetic point of view, VEGF-A (vascular epithelial growth factor) is the main actor in the angiogenetic process through the receptor VEGFR-2 and the coreceptor Neuropilin-1 ; VEGF-A is highly expressed in the subventricular zone first, then later in the neurons of the cortical plate, then after vascular remodeling is completed, in the glial cells. VEGFR-2 is expressed in the endothelial cells of the perineural vascular plexus and in the capillary sprouts. The Dll4/Notch signaling pathway further regulates the number/density of vessels within the parenchyma, as well as the Slit2/Robo4 signaling pathway: both prevent endothelial stalk cells from acquiring the tip cell phenotype. Integrins are cell adhesion receptors involved in cell-to-cell and cell-to-matrix interaction; they help to regulate angiogenesis by maintaining the endothelial cell communication with the neuroepithelium. Wnt7a and Wnt7b also appear to be critical in the regulation of central nervous system (CNS) angiogenesis, and their expression is required in the neuroepithelium during the angiogenetic process. After migration into the CNS, the blood vessels need maturation, remodeling, and pruning, together with the recruitment of vascular smooth muscle cells (VSMC): this involves the Pdgfb/Pdgfrβ pathway. The TGFβ signaling pathway is important for endothelial cell proliferation and differentiation, and for recruitment of VSMC; it involves endoglins and alk1, whose mutations cause hereditary hemorrhagic telangiectasia (HHT). A last pathway crucial to CNS angiogenesis is the Angiopoietin/Tie2 signaling pathway: deficient mice present with immature vessels lacking branching, organization in large and small vessels, and VSMC. Finally, a last interesting point to mention is that the tip cells of the endothelial channels behave like the growth cones of the axons: in the processes of remodeling and vessel navigation, they respond to the influence of the same guidance molecules. For example, ephrinB2 (arterial) and EphB4 (venous) are repulsive and are required for establishing and maintaining the arterial-venous interface; netrins are mostly repulsive (mostly attractant for axons); Slit/Robo are involved also, as mentioned above, as well as the Semaphorin3E-PlexinD1 signaling pathways (see Ref. for review).
Intrinsic vascularity develops from ventral ( ganglionic ) to dorsal ( pallial ) and in the germinal zone long before the cortex .
From a morphologic point of view, endothelial cell proliferate, canalize, approach, and connect with the neighboring channels to form simple arteriovenous loops into the deep germinal matrix first, and much later in the cortex: early branching does not appear in the cortical layers until week 20. At the early stages the vessels are not yet differentiated, their arterial or venous function being determined by the direction of the blood flow only (see Fig. 7 ). However, the vascular pattern becomes more elaborate as the cerebral mantle thickness and complexity increase, with a multiplication of intermediate small-caliber arteriovenous connections that become true capillaries (<2 red cells), and enlarging feeding and draining trunks (up to 10 red cells) that behave like true arterioles and venules. Only in the germinal zone do the primitive “sinusoid” capillaries remain undifferentiated until the germinal zone disappears at term. Because the perforators originate from the surface to feed the most active layer in the deep ventricular zone first, all arterial perforators are transcerebral.
- •
At 5 weeks, intrinsic vessels are seen entering the medulla and pons to ramify in the ventricular zone; this process becomes more prominent at 6 weeks, with other vessels entering the cerebellar rudiment as well as the rhombencephalic tela choroidea. In the following weeks the vasculature of the cerebellum expands to the territory of the 3 future cerebellar arteries. The development of the cerebellar cortex is significantly different in its modalities from the development of the cerebrum; however, to the best of the author’s knowledge, no specific description of the cerebellar angiogenesis is found in the literature.
- •
The early vascularization of the midbrain develops in a similar fashion, very dense from the early stage (5 weeks), first in the ventricular area, and later more diffusely. The vasculature of the diencephalon is also dense. Both start ventrally from the PCOMs, with longer feeders extending to the dorsal diencephalon (tela choroidea), and from the midbrain further toward the posterior and medial part of the cerebral hemispheres after 9 weeks, thus establishing the distal hemispheric territory of the PCA. At the same time, these distal branches of the PCOMs become supplied by the vertebrobasilar arteries.
- •
In the telencephalon, the first striatal branches appear at 5 weeks and become more numerous at week 6. These branches develop from the olfactory artery, future ACA (forming the artery of Heubner), and from the stem of the future MCA. The ACHA also gives branches to the basal diencephalon as well as to the posteromedial part of the hemisphere: the later contribution, however, regresses as that of the PCA expands, so that the ACHA comes to supply mostly the choroid plexus of the forebrain (and the temporal uncus).
- •
The cortex itself does not demonstrate any endothelial structure at 5 weeks. At 6 weeks a few endothelial strands without apparent lumen and without apparent connection with the surface network have been noted, suggesting that endothelial channels could originate de novo also in the parenchyma. By week 7 these channels seem to establish links with the leptomeningeal plexus while becoming canalized. By 12 to 15 weeks, radial, likely arterial vessels connected to the meningeal plexus course toward the germinal zone where they form a subventricular plexus, still without giving off any ramification to the cortical plate. Only by 20 weeks do horizontal intracortical branches start to arise in the deep cortical layers, with few recurrent branches directed to the more superficial cortex. These horizontal branches and their associated recurrent collaterals become more numerous from 20 to 27 weeks while new horizontal branches develop in the superficial layers of the cortex. From 27 weeks to term, new superficial radial branches develop from the superficial network. After birth the cortical vasculature becomes much denser, especially in the intermediate cortical layers. It seems that in response to the predominantly peripheral brain growth, shorter vessels develop in between the longer ones ; in the rat brain this has been described as forming a system of hexagonally packed vascular supply units that would provide and maintain an even distribution of the perfusion.
Similar findings were reported, obtained by using microradiography (radiopaque vascular injections) in brain specimens of neonates ranging in age from 23 to 40 weeks’ gestation. An abundant arteriolar network fed by the transcerebral arterial perforators was demonstrated in the ventricular and subventricular zone in preterms from 23 to 30 weeks. After 34 weeks this deep network progressively fades away to disappear toward the time of term. Note that the absence of any centrifugal arterial pattern and of any deep arterial border zone is specifically mentioned in the anatomic literature of the last decades, especially when using stereoscopic analysis.
The vascular fate ( arteries, capillaries, veins ) depends primarily on flow .
In the early fetal period all vessels are simple endothelial channels, and it is impossible to identify morphologically what is arterial, capillary, or venous. Because of their very simple hollow structure they are designated as “sinusoid” channels or “sinusoid” capillaries (this undifferentiated appearance is reproduced in undifferentiated tumors such as the glioblastoma multiforme). The arterial versus venous function is defined by the direction of flow only, not by the vascular structure. In addition, the arterial or venous fate of the vessels may change over time depending on the local metabolic conditions. However, most horizontal connections between the perforators are likely to represent the early capillary network.
- •
By tracking the course of the perforators, Allsopp and Gamble were able to identify transcerebral perforators from the germinal zone that seemed to connect with the venous plexus on the brain surface in a 5-week embryo, suggesting that the pattern of transcerebral medullary vein could be present early in the development. In the same developmental period they also noted vessels lining the third ventricular ependyma, possibly forming early subependymal collectors.
- •
Kuban and Gilles noted that while all channels in the germinal zone are sinusoid capillaries devoid of media and adventitia, larger channels with the characteristics of veins are identified at 27 weeks at the interface between the germinal tissue and the caudate, as well as at the angles of the lateral ventricles, so forming the early subependymal veins.
- •
A muscularis appears at midgestation (20 weeks) in the striatal vessels, giving them their arterial appearance. Development of this muscularis extends from the pial vessels toward the putamen (24 weeks) and the caudate (26 weeks), but does not extend into the germinal tissue.
- •
The extrastriatal parenchymal vessels remain sinusoid channels without a muscularis until the end of the gestation. At 24 weeks, they are relatively small (10–25 μm); they may divide into 5- to 15-μm capillaries, or converge to form 20- to 40-μm vessels at the ventricular surface, presumably venous. Toward the end of gestation, transcerebral trunks measure 20 to 40 μm and the collecting vessels 50 to 120 μm : a clear arteriovenous differentiation in the pallium therefore appears in the last weeks of gestation only.
For all observers, the arterial, venous, or capillary fate of the primary vessels appears to depend mainly on the flow. Still, specific signaling molecules have been identified that label the channels as arterial or venous at very early stages (see Ref. for review): ephrin-B2 , neuropilin-1 , notch3 , Dll4 , and gridlock for arteries; EphB4 , receptor for ephrin-B2, and neuropilin-2 for veins. However, experiments in which the flow pattern was artificially modified could reverse the arterial or venous character of the channels, and even change the expression of the markers, suggesting that it is the hemodynamics that plays the major role in arteriovenous specification and patterning of the intrinsic brain vessels.
The cranial neural crest cells give the forebrain arteries their identity .
A last point that should be mentioned regarding the brain arteries concerns the origin of their smooth muscle cells and pericytes (cells that mediate vasoconstriction and form part of the blood-brain barrier). Like that of the meninx primitiva, this origin is different in the cord, hindbrain, and midbrain vessels on the one hand, and in the forebrain vessels on the other hand. In the caudal parts of the brain and in the cord the arterial media and the pericytes derive from the mesoderm like the endothelial cells. By contrast, in the forebrain they originate from the neural crest (or mesectoderm ). The forebrain itself originates from the anterior neural plate, which forms an expansion of the neural tube rostral to and beyond the anterior end of the notochord. As mentioned earlier, the skeleton of the face and anterior skull base (maxillary, nasal, orbital regions, paranasal sinuses) as well as the coverings of the brain (meninges, calvarium, scalp, dermis) cannot have a somitic origin, and instead are produced by the neural crest. As the anterior neural plate itself is also devoid of neural crest, the midfacial skeleton and the coverings of the forebrain are made by neural crest cells that migrate from the posterior diencephalic and the mesencephalic portions of the neural tube. In a similar way, the same groups of neural crest cells form the muscular media and the pericytes of the forebrain arteries as well. The limit between the neural crest-associated forebrain arteries and the classic endothelium-associated mid- and hindbrain arteries is clearly demarcated at the level of the circle of Willis. Such a difference in origin might explain why arterial pathologies may be different in the forebrain from the more posterior segments: not only Stürge-Weber disease or meningo-angiomatosis, but also Moya-Moya disease and syndromes that characteristically affect the arterial supply to the forebrain only.
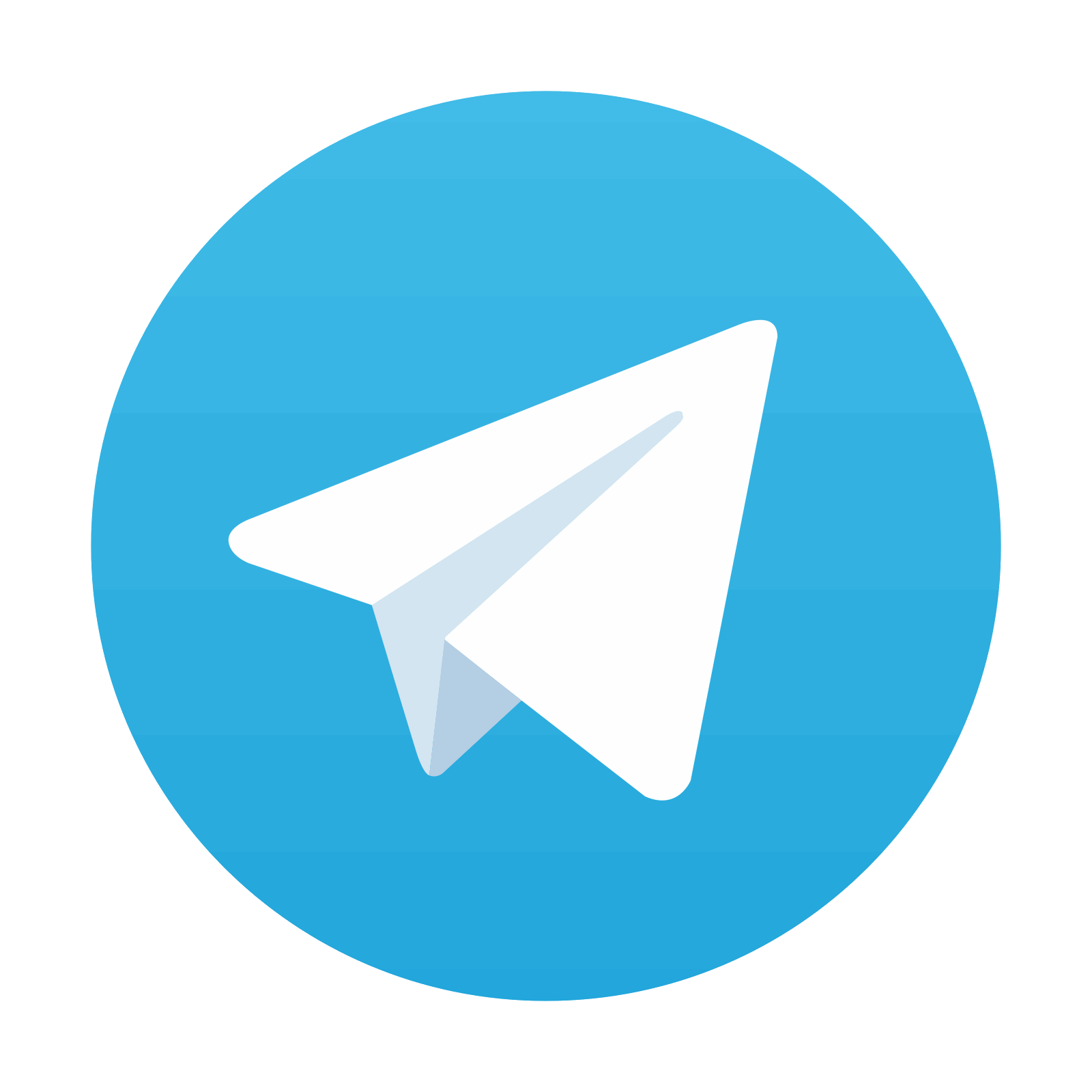
Stay updated, free articles. Join our Telegram channel

Full access? Get Clinical Tree
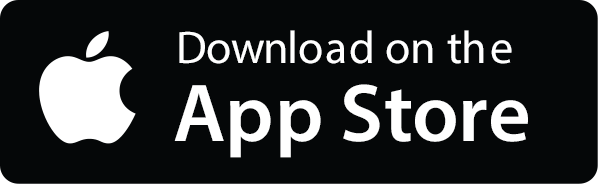
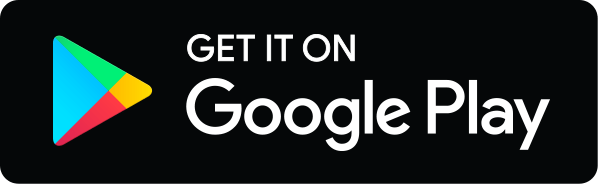