192 Normal EEG This chapter encompasses the patterns of cerebral and extracerebral origin as well as patterns of uncertain significance to illustrate the range of normal electroencephalogram (EEG) encountered in clinical practice. It reviews the parameters of recording and analyses the state of the patient and background activity. The chapter explores the most salient features of the epoch. The alpha rhythm is the starting point in the orderly approach to analyze EEG. In the normal EEG, a posterior dominant rhythm is represented bilaterally over the posterior head regions and lies within the 8-Hz to 13-Hz bandwidth. Beta rhythms are frequencies that are more than 13 Hz. Theta rhythms are composed of 4- to 7-Hz frequencies of varying amplitude and morphologies. Delta rhythms are frequencies that consist of <4-Hz activity. With new waveforms still being identified, EEG continues to remain at the forefront of neurological evaluations. alpha rhythm, beta rhythms, clinical practice, delta rhythms, neurological evaluations, normal electroencephalogram, theta rhythms Alpha Rhythm, Beta Rhythm, Delta Rhythm, Electroencephalography, Neurologic Examination, Theta Rhythm The EEG is a unique and valuable measure of the brain’s electrical function. It is a graphic display of a difference in voltages from two sites of brain function recorded over time. EEG involves the study of recording these electrical signals that are generated by the brain. Extracranial EEG provides a broad survey of the electrocerebral activity throughout both hemispheres of the brain. Intracranial EEG provides focused EEG recording directly from the brain from surgically implanted electrodes that are targeted at specific regions of the brain. Information about a diffuse or focal cerebral dysfunction, the presence of interictal epileptiform discharges (IEDs), or patterns of special significance may be revealed. For the successful interpretation of an abnormal EEG, one must first understand the criteria necessary to define normal patterns. While a normal EEG does not exclude a clinical diagnosis of epilepsy, an abnormal finding on EEG may be supportive of the clinical diagnosis. Furthermore, it may be indicative of cerebral dysfunction by demonstrating focal or generalized slowing. The results of a standard EEG may have nothing to do with the reason that the study was performed (i.e., patients with headache) and the results need to be taken in context with the individual reason for referral. It is the clinical correlation applied to the findings of the EEG that imparts its utility. Electrical signals are created when electrical charges move within the central nervous system. Neural function is normally maintained by ionic gradients established by neuronal membranes. Sufficient duration and length of small amplitude potentials (measured in microvolts) of electrical currents generated by cerebral activity are required to be amplified and displayed for visual interpretation. Normally, a resting (diffusion) membrane potential exists through the efflux of positive-charged (potassium) ions maintaining electro-chemical equilibrium at −75 mV. With depolarization, an influx of positive-charged (sodium) ions exceeding the normal electrochemical resting potential occurs. Ion channels open within the lipid bilayer via a voltage-dependent mechanism, and closure is time-dependent. Conducting the change in cellular depolarization to adjacent portions of the nerve cell membranes results in an action potential when threshold is exceeded. However, it is the synaptic potentials as opposed to the action potentials that are the most important source of the extracellular current flow to produce the EEG. Excitatory postsynaptic potentials (EPP) flow inwardly (extracellular to intracellular) to other parts of the cell (sinks), via sodium or calcium ions. Inhibitory postsynaptic potentials (IPP) flow outwardly (intracellular to extracellular) in the opposite direction (source) and involve chloride or potassium ions. These summed potentials are longer in duration than action potentials and are responsible for most of the EEG waveforms. The brainstem and thalamus serve as subcortical generators to synchronize populations of neocortical neurons in both normal (i.e., sleep elements) and in abnormal situations (i.e., generalized spike-and-wave complexes). Volume conduction characterizes the process of current flow from the brain generator and recording electrode. Layers of cortical neurons are the main source of the EEG. Pyramidal cells are the major contributor of the synaptic potentials that make up EEG (Figure 2.1A). These neurons are arranged in a perpendicular orientation to the cortical surface from layers III, IV, and VI. 20Volumes large enough to permit measurement at the surface of the scalp require areas that are >6 cm2 though >10 cm2 for recording most IEDs on the scalp using the International 10–20 system of electrode placement due to the attenuating properties incurred by the scalp and skull. All generators have both a positive and negative pole and function as a dipole (see Figure 2.1B). The EEG displays the continuously changing voltage fields at different locations on the scalp. FIGURE 2.1. (A) A pyramidal cell with excitatory postsynaptic potentials and inhibitory postsynaptic potentials. (B) Dipole depicting a field of charge separation. Standard EEG recording displays the difference in electrical potentials between two different sites on the scalp overlying cerebral cortex that is closest to the recording electrode (Figure 2.1). During routine use, electrical potentials are acquired indirectly from the scalp surface, and incorporate waveform analyses of frequency, voltage, morphology, and topography. However, most of the human cortex is buried deep beneath the scalp surface. In addition, EEG represents a 2-dimensional projection of a 3-dimensional source. This presents challenges to solve the inverse problem of source localization using scalp EEG. Furthermore, the waveforms that are recorded from the scalp represent pooled synchronous activity from large populations of neurons that create cortical potentials and therefore may not reflect small interictal or ictal generators. Initial one-channel EEG recordings in the late 1920s have evolved to sophisticated digital-based computerized recording devices. From the patient scalp, electrodes conduct electrical potentials to an electrode box (jackbox). Thereafter, the montage selector permits EEG signals to pass through amplifiers before filtering and ancillary controls regulate the signal output. Data display follows acquisition and processing and has a wide variety of data presentation for EEG interpretation. Electrode placement has been standardized by an international system that uses anatomic landmarks on the skull. These sites are then subdivided by intervals of 10% and 20% to designate the site where an electrode will be placed. A minimum of 21 electrodes are commonly used for clinical studies, though digital EEG now has the capability for a greater number and 25 electrodes to accent basal temporal structures are recommended. During infant EEG recordings, fewer electrodes are often used and vary depending upon age and head size. A newer modified combinatorial electrode system uses electrode placement with more closely spaced electrodes in a 10–10 system (see Figure 2.2). The designations, Fp (frontopolar), F (frontal), T (temporal), O (occipital), C (central), and P (parietal) are utilized in the 10–20 system. Subsequently, numbers combined following the letters for location reflect either the left (odd numbers) or right (even numbers) hemisphere 21of electrode placement. The “z” designation reflects midline placement (i.e., Cz = central midline). In the 10–10 system, lower numbers in their positions reflect locations closer to the midline, and T3/T4 become T7/T8 while T5/T6 become P7/P8. Electrode impedances should be maintained between 100 and 5,000 ohms. Special electrodes may also be added, such as sphenoidal, true temporal, or fronto-temporal electrodes. Most are employed for the purpose of delineating temporal localization. True temporal electrodes (designated T1/T2 and FT9/FT10) are placed to help distinguish anterior temporal or posterior inferior frontal location to enhance recovery compared with F7 and F8 electrode positions. Combining the 10–20 system with electrodes from the 10–10 system may be most practical for routine clinical use when additional electrodes are desirable. Collodion is a compound used to secure electrodes during prolonged recording techniques, such as during video-EEG, continuous EEG in the intensive care unit, or ambulatory EEG monitoring. Paste used for routine recordings is more temporary. Subdermal electrodes are used when other recording techniques are not feasible, such as in the OR and ICU. FIGURE 2.2. Electrode placement systems use either a 10–20 system (blue) or modified combinatorial system with 10–10 electrode placement (blue + gold). Other additional electrodes may include EKG (recommended with every EEG), eye movement monitors, EMG, and extracerebral electrodes to aid in artifact differentiation (i.e., tremor monitor) and sleep staging. Respiratory monitors may also be important if respiratory problems are identified or sleep monitoring is performed.22 FIGURE 2.3. (A) Bipolar montage demonstrating phase reversal. (B) Referential montage demonstrating absolute voltage. The electrical “map” obtained from the spatial array of recording electrodes used to interpret EEG is the montage (Figure 2.3). Several montages are used throughout a 20- to 30-minute EEG recording. Every standard EEG should include at least one montage using an anterior-posterior longitudinal bipolar, reference, and traverse bipolar montage (Figure 2.4). A reference montage uses an active electrode site as the initial input, and then at least one “neutral” electrode to depict absolute voltage. The greatest amplitudes measured are commensurate with the site of maximal electro-negativity or positivity. One midline reference electrode (i.e., Pz), may be useful for a lateralizing temporal recording. However, two references (i.e., ipsilateral ear reference) may be useful for more generalized discharges. Even multiple “averaged” sites of reference (or Laplacian montages for very focal recordings) may be useful for localized discharges. Bipolar montages may be arranged in many different spatial formats, including longitudinally, transverse fashion, or in a circumferential pattern. The longitudinal bipolar (aka “double banana”) is the one that is most frequently represented in clinical practice and throughout this text. An anterior to posterior temporal and central transverse connecting chain of electrodes arranged left alternating to right-sided placement is an example of a targeted array to lateralize and cross-localize temporal abnormalities. Bipolar montages compare two active electrodes sites adjacent to each other and signify absolute electrographic sites of maximal negativity (or positivity) by phase-reversals. FIGURE 2.4. EEG demonstrating bipolar (A) and reference (B) montages to illustrate a left anterior temporal sharp wave at the F7 electrode (left) and T1 derivation (right). FIGURE 2.5. The rules governing polarity and convention are relative to deflection of the waveform. By convention, when input 1 is negative, the deflection is up. Polarity of the EEG thus has two reasons for an upward or downward deflection. By convention, when the voltage difference between electrode 1 is more negative than electrode 2, deflection of the waveform is up (Figure 2.5). Recordings are usually performed with a visual display speed of 30 mm/sec (slower with sleep studies). Amplifier sensitivities of 7 μV/mm and filter settings of 1 to 70 Hz are routine parameters during standard EEG. Reducing the low filter settings promotes slower frequency representation, while reducing high filter settings attenuates high frequencies. A narrow band reduction is possible using a “notched” filter setting to limit 60-Hz interference (or 50 Hz in some countries outside the United States). Proprietary software offers digital seizure and spike detection capabilities for digital EEG. These systems are commercially available for both standard and prolonged EEG monitoring. This section will encompass patterns of cerebral and extracerebral origin as well as patterns of uncertain significance to illustrate the range of normal EEG encountered in clinical practice. Like most interpreters for clinical EEG, the orderly approach includes (a) review of the parameters of recording, (b) identifying the state of the patient and background activity, and (c) the most salient feature(s) of the epoch. 24NORMAL EEG FIGURE 2.6. Normal 10-Hz alpha rhythm “blocked” by eye opening and returning on eye closure. Note the faster frequency immediately on eye closure (“squeak”). The alpha rhythm is the starting point in the orderly approach to analyze EEG. In the normal EEG, a posterior dominant rhythm is represented bilaterally over the posterior head regions and lies within the 8- to 13-Hz bandwidth (alpha frequency). When this rhythm is attenuated with eye opening, it is referred to as the alpha rhythm (Figure 2.6). During normal development, an 8-Hz alpha frequency appears by 3 years of age. The alpha rhythm remains stable between 8 and 12 Hz even during normal aging into the later years of life. In approximately 25% of normal adults, the alpha rhythm is poorly visualized, and in <10%, voltages of <15 μV may be seen. The alpha rhythm is distributed maximally in the occipital regions and shifts anteriorly during drowsiness. Voltage asymmetries of >50% should be regarded as abnormal especially when the left side is greater than the right. It is best observed during relaxed wakefulness and has a side-to-side difference of <1 Hz. Unilateral failure of the alpha rhythm to attenuate reflects an ipsilateral abnormality (Bancaud’s phenomenon). Normally, alpha frequencies may transiently increase immediately after eye closure (alpha squeak). Alpha variants include forms that are ½ (slow alpha) or 2 times (fast alpha) the alpha frequency with similar distribution and reactivity. Alpha variants may have a notched appearance. Paradoxical alpha occurs when alerting results in the appearance of alpha, and drowsiness does not. FIGURE 2.7. Note the prominent left central mu rhythm during eye opening.
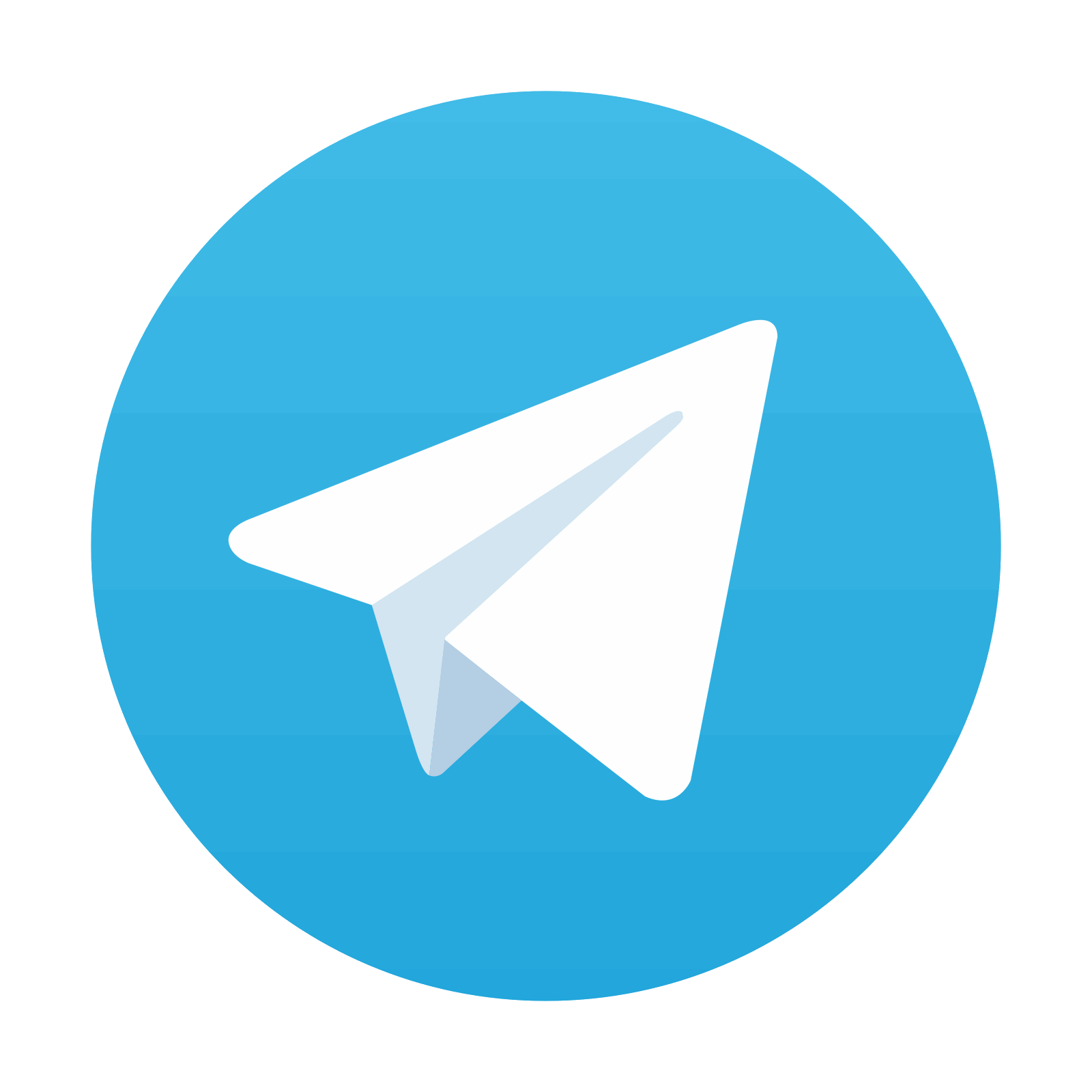
Stay updated, free articles. Join our Telegram channel

Full access? Get Clinical Tree
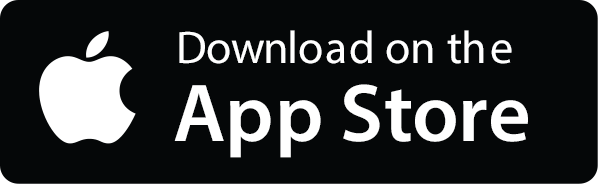
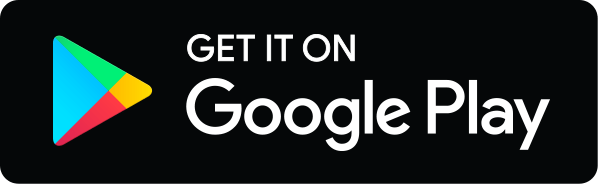