Nucleus Pulposus Replacement: Basic Science and Indications for Clinical Use
Michael A. Pahl
Joon Yung Lee
Alexander R. Vaccaro
Degenerative disc disease affects millions of people within the United States each year. When patients are not responsive to conservative management, surgery rarely may be an option. However, traditional surgical treatment such as an arthrodesis creates a nonphysiologic motion within the spinal axis that may produce greater stresses across the remaining discs as they compensate for the nonmobile motion segment. As a result, the risk for adjacent segment degeneration may increase in up to 35% to 41% of patients who undergo a discectomy and fusion (1,2,3). One solution to this problem may be to treat degenerative disc disease with a more physiologic surgery. An artificial disc prosthesis, such as Charité by DePuy-Acromed (Raynham, MA), attempts to recreate stable intervertebral spinal segment motion by replacing the excised disc with an articulating implant.
Several artificial disc prostheses have been used clinically in Europe for many years, and the Charité device has been recently approved by the Food and Drug Adminis-tration (FDA) for general use in the United States for single-level degenerative disc disease as of this writing. The designs of the current total disc replacements, however, mandate similar surgical dissection as the traditional anterior lumbar interbody arthrodesis with complete removal of the intervertebral disc anulus. As minimally invasive procedures become more sophisticated, there have been some technologic advances that may allow less invasive replacement of the diseased disc. This alternative is the nucleus pulposus replacement procedure. The theory behind nucleus pulposus replacement is that the native anulus is left intact, and the diseased nucleus is replaced via more minimally invasive approaches. The technology of delivery of these implants and mechanisms to ensure their stability and positioning are currently in the infant stages, and further testing to validate their theoretical advantages are presently ongoing.
Nucleus Pulposus Anatomy and Biomechanics
The nucleus pulposus is a jellylike structure located within the core of the interver-tebral disc and is surrounded by the lining of the disc or the anulus fibrosus. The nucleus pulposus consists of a matrix of collagen, proteoglycans, and water. The anulus fibrosus also consists of collagen, proteoglycans, and water; however, the type of collagen is different and the relative proportion of collagen is higher than in the nucleus pulposus. The nucleus pulposus, in turn, has a higher relative proportion of proteo-glycans and water.
The proteoglycans are large extracellular molecules consisting of glycosamino-glycans linked to proteins. The glycosaminoglycans found within the nucleus pulposus include chondroitin 6-sulfate, chondroitin 4-sulfate, keratin sulfate, and hyaluronate (4). Proteoglycans are negatively charged and have the ability to attract and link with water molecules, primarily due to their content of chondroitin 4-sulfate. As the relative proportion of chondroitin 4-sulfate increases, so does the ability to attract and retain water. A disc with high proteoglycan content has the ability to maintain a higher fluid capacity than a disc with lower proteoglycan content. A newborn disc contains high proteoglycan content and has a fluid content that comprises up to 88% of the disc’s weight. With aging, the proteoglycan content decreases and the net charge of the extracellular matrix of the nucleus pulposus becomes less negative (5). This leads to a reduction in disc hydration with the fluid content of the disc only accounting for approximately 65% of the disc weight in a 77-year-old (6).
Disc collagen is composed primarily of type I and type II collagen. In the outer anulus fibrosus, collagen accounts for about 70% of the dry weight of the disc, decreasing to around 6% to 25% in the center of the nucleus pulposus (7). The nucleus pulposus is comprised primarily of type II collagen, which is designed to resist compression. The anulus fibrosus is made primarily of type I collagen and is designed to resist tensile forces. However, due to the tensile and compressive forces to which the anulus fibrosus is subjected, it contains a substantial amount of type II collagen as well (8).
The proportion of type I collagen decreases from its highest concentration in the periphery of the anulus fibrosus to its lowest concentration next to the nucleus pulposus. Type II collagen concentration is inverse to this with its highest concentration in the center of the nucleus pulposus and decreasing outward toward the periphery of the anulus fibrosus. The collagen in the anulus fibrosus is arranged in sheets, called lamellae, with the fibers arranged in opposite directions to each other at an angle of 120 degrees (9). The blood supply to the disc is limited, as is its ability for regeneration (10).
A healthy nucleus pulposus maintains the joint space between adjacent vertebrae and places tension on the anular fibers of the disc, which increases the stability across the disc space. This allows for proper shear force transmission across the disc and compressive force disbursement. Adjacent vertebrae are connected together by the “triple joint complex.” This consists of the intervertebral disc anteriorly and each of the facet joints posteriorly. In the presence of a diseased nucleus pulposus, water content is lost and the disc decreases in size, primarily in height. This causes slackening of the anular fibers as they relax and allows greater shear forces to be transmitted across the disc space, in turn placing greater loads on the facet joints. This can cause changes in the facet articular cartilage biology. The fluidlike nature of the nucleus pulposus also gives it a floating center of rotation and allows for it to shift within the disc in response to forces placed on it (11). During forward flexion, the nucleus pulposus translates dorsally, and during extension, translates ventrally. This allows for great spinal motion segment flexibility while decreasing the loads across the facet joints.
Indications for Nucleus Pulposus Replacements
The trend in spinal surgery is to minimize soft tissue dissection and restore physiologic motion. Replacement of the nucleus pulposus may fulfill these two goals. Nucleus pulposus replacement is primarily indicated for symptomatic lumbar discogenic back pain. As with any surgical intervention, nucleus pulposus replacement is only considered if the patient’s complaint of back pain, preferably isolated to a single symptomatic disc
level, is not responsive to conservative treatment for at least 6 months. Consideration for primary replacement of the nucleus of a disc following disc herniation excision in the presence or absence of low back pain is presently under debate.
level, is not responsive to conservative treatment for at least 6 months. Consideration for primary replacement of the nucleus of a disc following disc herniation excision in the presence or absence of low back pain is presently under debate.
Nucleus pulposus replacement should not be technically performed if disc height loss is greater than 50% of an adjacent normal disc or if there are Schmorl nodes present within the disc’s respective endplates (12,13). Nucleus pulposus replacement is best indicated for discs with early degenerative changes on magnetic resonance imaging (MRI), with no more than grade I spondylolisthesis, in the setting of a positive discogram.
Nucleus Pulposus Replacement Design
As the disc degenerates, the nucleus pulposus loses its ability to attract and maintain water. This causes the nucleus pulposus to lose its height and allows for loss of tension within the fibers of the anulus fibrosus. Mechanically this may lead to multidirectional increased motion of the spinal interspaces. By replacing the nucleus pulposus, the surgeon hopes to restore tension to the fibers of the anulus fibrosus in tension.
Key in the development of a nucleus pulposus replacement is to design a device that can handle repetitive stresses over an extended period of time. A total disc replacement, for example, is designed with materials such as cobalt chromium and high molecular weight plastics, which have a proven track record in other motion preserving procedures such as total joint replacement devices in hips and knee surgery. Kostuik (14) determined that the average implant would experience approximately 100 million loads as a result of strides over the course of 40 years.
The implant must also have low wear characteristics and generate minimal particulate matter with use. The particulate matter formed must also have no associated toxicity or carcinogenicity.
Finally, the nucleus pulposus implant should have stiffness similar to that of the native disc. This is to prevent stress shielding, atrophy, and resorption of the underlying vertebral endplate. This can all lead to subsidence of the vertebral endplate with extrusion of the implant into the vertebral body if the implant is too stiff and the loads carried by the implant are greater then the underlying bone (15).
There are currently numerous nucleus pulposus replacement designs that are under clinical investigation. Several of these devices use a variety of three-dimensional expanding polymers including hydrogels and elastomers, and others are mechanical and built from pyrocarbons and metallic springs. Several of the polymers are implanted in a semihydrated state through minimally invasive methods and then hydrated once inside the disc to restore the disc space height, whereas others are injected in a liquid state and cure in situ. The mechanical devices are implanted much like a disc replacement, except that they are designed to be anulus fibrosus sparing as they function as a nucleus pulposus replacement.
Nucleus Pulposus Implant Devices
Polymer Based Implants
Aquarelle (Stryker Spine, Allendale, NJ)
This device is a hydrogel pellet. It is composed of a semihydrated polyvinyl alcohol hydrogel. When implanted, it contains approximately 80% water, but its final volume
depends on the water content at equilibrium (Fig. 12.1A). Its swelling pressure is similar to that of the nucleus pulposus in vivo. In biomechanical testing, it has demonstrated durability up to 40 million cycles.
depends on the water content at equilibrium (Fig. 12.1A). Its swelling pressure is similar to that of the nucleus pulposus in vivo. In biomechanical testing, it has demonstrated durability up to 40 million cycles.
It is implanted in a minimally invasive fashion by a pressurized trocar into the disc through a small annulotomy (Fig. 12.1B, C). It can be implanted using either a posterior or lateral approach. Cadaveric studies have demonstrated a high extrusion rate of up to 20% to 33% with devices implanted in a posterolateral and anterior approach, respectively (5). However, further testing has revealed that when fully hydrated, extrusion only occurred when the disc space was placed under loads well beyond what is expected in vivo (16).
The device had been involved in clinical trials in Europe, but at present the manufacturer has placed further development of this product on hold until results of these trials are analyzed.
NeuDisk (Replication Medical Inc., New Brunswick, NJ)
This device is composed of a modified hydrolyzed poly-acrylonitrile polymer called Aquacryl. The polymer is reinforced by a Dacron mesh. It is inserted in a dehydrated state using minimally invasive methods and hydrolyzed in vivo where it absorbs up to 90% of its weight in water (Fig. 12.2A). It is designed to expand primarily in a vertical direction to restore disc height and absorb compressive axial load forces (Fig. 12.2B, C). In vitro biomechanical testing in normal healthy lumbar spines has shown a mean disc space height increase of 1.8 mm (17).
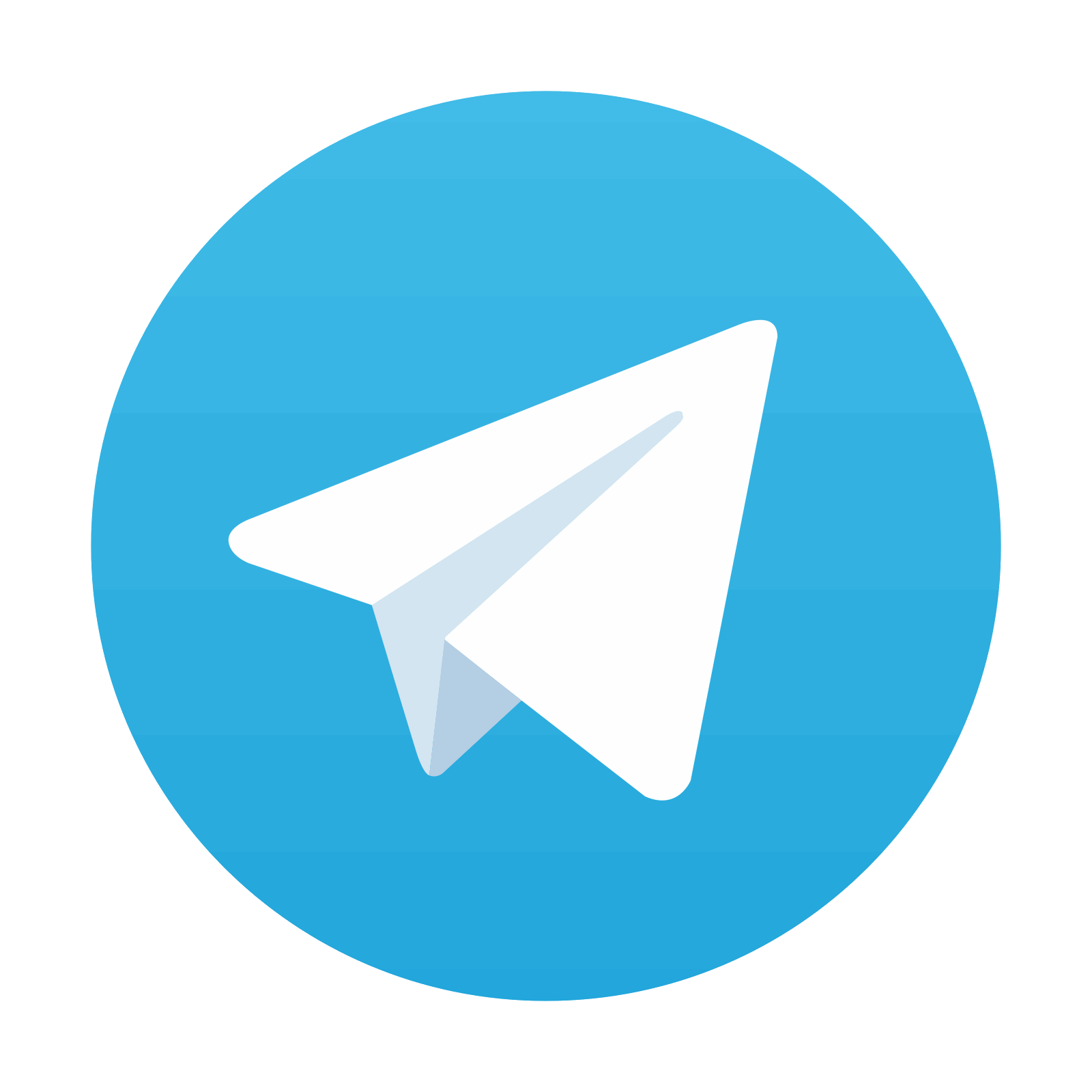
Stay updated, free articles. Join our Telegram channel

Full access? Get Clinical Tree
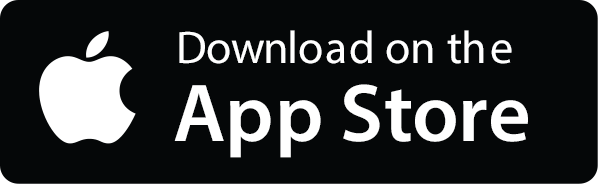
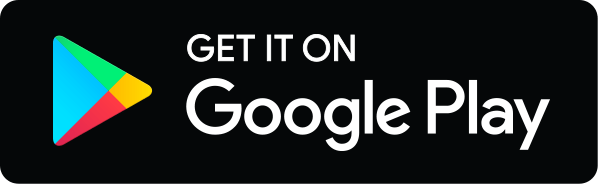
