• Acetylcholine
• Adenosine
• Agouti-related peptide
• Angiotensin II
• Calbindin
• Calcitonin gene-related peptide
• Cholecystokinin
• Cocaine– and amphetamine–regulating transcript peptide
• Corticotropin-releasing hormone
• Cortisol
• Delta sleep-inducing peptide
• Dopamine
• GABA (gamma-aminobutyric acid)
• Galanin
• Gastrin-releasing peptide
• Glutamate
• Ghrelin
• Growth hormone-releasing hormone
• Incretins gastric inhibitory polypeptide
• Glucagon-like peptide-1
• Histamine
• Insulin
• Leptin
• MCH (melanin-concentrating hormone)
• α–Melanocyte–stimulating hormone
• Neuromedin B
• Neuromedin U and neuromedin S (in the SCN)
• Neuropeptide Y
• Neurotensin
• NO (Nitric oxide)
• Noradrenaline (norepinephrine)
• Opioid peptides dynorphin
• Endorphins
• Enkephalins
• Orexins (hypocretin), especially orexin A
• Peptide YY (peptide tyrosine tyrosine)
• Pituitary adenyl cyclase activating peptide
• Prostaglandins (in the gut, especially E and F)
• Serotonin (5-HT), especially 5-HT3, 5-HT4, and 5-HT1p receptors
• Somatostatin
• Substance P
• Thyrotropin-releasing hormone
• Vasoactive intestinal peptide
Cholecystokinin and many other humoral factors have a role in the CNS-ENS network. The regulation of the sleep–wake cycle is complex, and there are different theories. The important brain transmitters in sleep–wake regulation include norepinephrine, acetylcholine, 5-hydroxytryptamine (5-HT), dopamine, glutamate, histamine, adenosine, γ-aminobutyric acid (GABA), and hypocretin. Also, prostaglandins and different peptides have an effect on regulation of alertness/sleepiness. In the past, the focus was on norepinephrine, acetylcholine, and 5-HT. They all play important roles, but do not explain “why we sleep.” The role of adenosine and regulation of brain energetics is probably central. During wakefulness, extracellular adenosine increases; this increase in adenosine decreases wakefulness and causes sleepiness. Caffeine, the most common stimulant, is an adenosine receptor antagonist.
Role of Neuromediators
Peptides and Hormones
Cholecystokinin is a mixture of peptides, of which octapeptide is the most effective. It is secreted by duodenal and jejunal cells after eating food. Cholecystokinin acts on the gall bladder and the pancreas, stimulating bile production and the release of pancreatic digestive enzymes. It also acts on vagal neurons projecting to the brain stem, giving a signal of satiety that inhibits further need for eating.
Ghrelin is a peptide containing 28 amino acids. It is secreted mainly in the stomach, but its receptors (growth hormone secretagogue receptors) are present widely in the gastrointestinal (GI) tract, the ENS, and also in the CNS neurons around the arcuate nucleus. In the GI tract, ghrelin has prokinetic effects, and centrally it increases appetite. It acts on the hypothalamus to stimulate feeding, counteracting the inhibitory effects of leptin and peptide YY3-36 (PYY3-36; see later). Through its actions on the GABA system, ghrelin inhibits pro-opiomelanocortin (POMC) and cocaine and amphetamine-regulated transcript (CART) neurons and decreases production of α-melanocyte-stimulating hormone (α–MSH; see later) in the arcuate nucleus area, inhibiting the anorexigenic effects of α–MSH (i.e., increasing food intake).
Leptin is a protein of 167 amino acids and is manufactured mainly in fat cells in adipose tissue. The amount of circulating leptin correlates positively with the amount of fat in the body. Leptin has many CNS effects. It counteracts the effects of neuropeptide Y (NPY), agouti-related protein (AgRP), and GABA containing neurons and activates production of α-MSH in the arcuate nucleus. As a consequence, leptin is catabolic. It decreases appetite and inhibits food intake, contrary to ghrelin. A decrease in leptin is associated with an increase in hunger and appetite.
The inhibitory effects of leptin last long time, in contrast to the rapid inhibition of eating behavior produced by cholecystokinin and the slow suppression of hunger between meals mediated by PPY3-36. Both leptin and ghrelin have been investigated in several studies attempting to find a relationship between obesity, sleep apnea, and weight loss, but leptin does not seem to have significant effect in weight loss therapy [13–15]. Leptin is low among subjects with anorexia nervosa [16] Low levels of leptin are also associated with hyperactivity, and it has been hypothesized that leptin could have a role in the treatment of severely hyperactive patients [16].
Neuropeptide Y is a potent feeding stimulant secreted by cells in both the gut and the hypothalamic neurons. It causes increased storage of ingested food as fat. NPY inhibits transmission of pain signals to the brain.
Peptide YY 3–36 is close in structure to NPY but, contrary to NPY, is a potent inhibitor of feeding. PYY3-36 is released by cells in the intestine after meals. The secretion increases with the amount of calories eaten, especially when these derive from proteins rather than from carbohydrates or fats. This may explain partly why people who eat a lot of carbohydrates are often hungry, developing obesity.
α–Melanocyte–stimulating hormone (α–MSH) is an anorexigenic melanocortin. Melanocortins are products of POMC gene in the arcuate nucleus in the mediobasal hypothalamus. Other melanocortins include ACTH, β-MSH and γ-MSH, β-endorphin and met-enkephalin. Alpha-MSH is responsible for production of melanin (tannin) by melanocytes in skin and hair in humans. The POMC cells are glucose-sensing cells [17]. They act to suppress appetite and food intake. In addition to central effects, melanocortins act on adipocytes by stimulating lipolysis and inhibiting leptin secretion. The melanocortins, α-MSH probably as the most important, are dominant anorexigenic peptides and important regulators of food intake.
AgRP blocks the action of α-MSH in the arcuate nucleus and is also involved in the regulation of food intake. The hypothalamic POMC and AgRP neurons are major targets of leptin and insulin action in the brain. As noted above, leptin stimulates POMC/CART and inhibits AgRP secretion [18].
Hypocretins (orexins) [19, 20] are produced by about 70,000 cells in the lateral hypothalamus. They were originally considered to be important particularly in central control of food intake. Hypocretin is an “orexigenic” neuropeptide, increasing food intake (N.B. interestingly in narcolepsy, with loss of orexin, weight gain is often observed), contrary to “anorexigenic” neuropeptides that decrease food intake. It is now evident that hypocretins have a much larger spectrum of action, including energy homeostasis, sleep–wake behavior, nociception, reward-seeking behavior, and drug addiction [10, 21, 22]. Hypocretin-producing cells can be directly modified by peripheral signals. They are sensing peripheral glucose, and they have receptors for leptin and ghrelin [23]. Hypocretins are also widely present in the GI tract [10]. In the ENS, they have a role in regulation of GI motility, and also in gastric, intestinal, and pancreatic secretions. The relationships among enteric regulation, eating behavior, regulation of arousal, narcolepsy [24–26], and the orexin-hypocretin system remain to be further clarified. It is most probable that there are important interactions that explain many of the symptoms, including the effects of fasting and carbohydrate intake on the vigilance of narcoleptic subjects versus normal people.
Melanin-concentrating hormone (MCH) An important interaction exists between hypocretin cells and intermingling MCH cells in the lateral hypothalamus. Both hypocretin-producing cells and MCH cells are glucose–sensing cells—sensing blood glucose concentrations. Decrease in glucose stimulates hypocretin cells and inhibits MCH cells (increasing alertness and food intake) [23, 27–29]. Increase in blood glucose inhibits hypocretin cells and stimulates MCH cells (decreasing alertness and decreasing food intake). Both hypocretin and MCH projections are widely distributed. They are located close to each other, having synaptic interconnections, and modulating each other’s cellular activity. According to these theories, the MCH–hypocretin interactions are crucially important in the regulation of food intake and energy balance. MCH cells are involved also in regulation of REM sleep and in narcolepsy and disturbed MCH and propiomelanocortin system may be related to development of obesity in narcolepsy [30, 31].
Anandamine is an endocannabinoide that is produced in the small intestine. It is “orexigenic,” i.e., it increases appetite. Anandamine has a role especially in the hedonic regulation of eating. It is involved in removing unnecessary short-term memories. It is also responsible for slowing down movements, making people feel relaxed and calm [32].
Insulin is produced in pancreas by β cells in islets of Langerhans. The effects of insulin on glucose- and lipid metabolism are known better than those of the other mediators. Insulin increases satiety and decreases appetite. Insulin stimulates POMC/CART neurons in the arcuate nucleus, generating an anorexigenic signal [18].
Serotonin
Serotonin (5-HT) is an important neurotransmitter in the CNS with important effects on sleep–wake regulation. It also has an important role in regulation of GI function through an interaction with the ENS. The ENS can be considered the body’s second brain, with more than 100 million neurons of different types. Up to 60–90 % of the total body amount of 5-HT is in the GI tract, and 2–20 % of all enteric neurons express 5-HT. The control of 5-HT’s release from enterochromaffin cells is complex. Stimulatory receptors include β-adrenergic receptors, muscarinic and nicotinic acetylcholine receptors, and 5-HT3 receptors. Inhibitory receptors include α2-adrenergic, histamine H3, GABAB, adenosine A2, and 5-HT4 receptors. In the GI tract, 5-HT is eliminated mainly by monoamine oxidase metabolism [11, 33].
An interesting element from the point of view of sleep and movement disorders is the 5-HT1p receptor. This receptor is probably involved in the secretory and peristaltic reflexes of the GI tract. The molecular identification of this receptor is not yet clear. Lui and Gershon [34] suggested that this receptor is a heterodimer of the 5-HT1B/1D receptor and the dopamine D2 receptor. Other studies have also shown that dopamine is involved in GI tract regulation. Gershon’s group has also shown in knockout mice that intestinal motility is abnormal when D2 is absent [35]. A dysfunction of the intestinal dopamine system probably explains many of the GI symptoms, especially problems of constipation that are usually present very early among patients with Parkinson’s disease (PD). It remains to be seen whether there are some associations with symptoms of RLS, lack of iron, and dysfunction of the 5-HT–dopamine system of the GI tract.
Irritable Bowel Syndrome and Role of the Intestinal Microbiota
Irritable bowel syndrome (IBS) is one of the most common GI disorders. It is twice as frequent among women as among men. IBS is related to dysfunction of the enteral serotonin system. There are no published systematic epidemiologic studies about the occurrence of IBS among patients with RLS. In our own clinical database, GI symptoms had been tabulated from 141 RLS patients (unpublished observations). Of these patients, 17 (12 %) had GI symptoms that could be diagnosed as IBS type. Because we had not tabulated this history systematically, the true prevalence might be higher. On the other hand, about 29 % of patients with IBS have RLS [36].
Intestinal microbiota has a role as a regulator of autoimmune diabetes in animals, and probably also in humans [37]. The role of gut microbiota in other human HLA-associated autoimmune diseases, including narcolepsy, remains to be studied. Small intestine bacterial overgrowth (SIBO) is common in RLS. In a recent series, SIBO was found in almost 70 % of patients with RLS [38]. In a randomized study, all 20 patients with RLS had marked improvement in their RLS symptoms after treatment of SIBO [39]. The therapy of SIBO was based on rifaximin, which is a rifamycin-based nonsystemic antibiotic, meaning that the drug will not pass the GI wall into the circulation [39–41]. Although treatment with antibiotics may be beneficial, the spectrum of gut microbes is changed, which may be followed by various adverse effects and symptoms that may last years. Changes in the gut microbiota may be involved in many neurological and psychological symptoms [42]. The intestinal microbes are also known to be able to produce many neurotransmitters such as GABA, serotonin, melatonin, catecholamines, histamine, and acetylcholine [42–44]. Different diets have been advised for patients with IBS. Some beneficial results have been obtained by use of probiotics and by FODMAP diet. That diet is low in fermentable oligosaccharides, disaccharides, monosaccharides, and polyols (FODMAPs) that are poorly absorbed by the small intestine and fermented by bacteria in the large intestine [45].
Case Example
A 53-year-old male biochemist developed severe insomnia 3 weeks after returning from a congress in South America. He had difficulty falling asleep and he woke up repeatedly, sleeping only 2–3 h. After 3 weeks of insomnia, he became exhausted and consulted our sleep clinic. He was lean. One week before consultation, he had started zopiclone and fluoxetine because he was diagnosed to have depression, which he denied. There was no previous history of insomnia. The polysomnography at the laboratory was consistent with his history. Total sleep time was only 2.3 h, and there was no slow-wave sleep. No apnea or periodic limb movements were found.
In the clinical interview at the sleep clinic, it was found that he had diarrhea with a fever during his travel. He took ciprofloxacin for 5 days but, because he continued to have diarrhea, he consulted an internist. A variety of laboratory tests were done to exclude all potential bacterial, viral, and tropical diseases. Nothing specific was found, but he was given two more antibiotics, including metronidazole. The diarrhea became much better, and his body temperature was between 37.0° and 37.3 °C. Insomnia developed one week after the last antibiotics had been taken. The problem was discussed with the patient. The internists concluded that he had had either bacterial or viral enteritis but no serious disease had been found. He speculated that the strong antibiotics had destroyed the entire bacterial flora, including healthy saprophyte bacteria.
Because his general condition was quite good, we decided to stop further laboratory examinations and start down-titrating zopiclone and fluoxetine. He also started to take omega-3 fatty acids, and Lactobacillus casei/Enterococcus faecium to normalize his intestinal flora. We encouraged him to restart exercise. After three weeks, he began to sleep normally as he had done before. His insomnia was of a psychophysiologic type, and anxiety (fear of some severe disease) probably also played a role. It is possible that his insomnia could have been caused by a change in the intestinal flora, and hence changes in the ENS-CNS regulation. The amelioration of his insomnia by reintroducing bacteria in the diet, without any other treatment, is in favor of this theory.
Role of Neurosteroids
Neurosteroids are synthesized in astrocytes, oligodendrocytes, Schwann cells, Purkinje cells, hippocampal neurons, and retinal amacrine and ganglion cells [46, 47]. Cholesterol is transported to glial mitochondria, where it is converted to pregnenolone. In the cytosol, pregnenolone is then converted to different neurosteroids such as allopregnanolone and dehydroepiandrosterone (DHEA). DHEA is a precursor of testosterone, which is converted to estradiol by an aromatase [46, 47]. Vitamin D is also an important neurosteroid [48]. Synthesis of neurosteroids is regulated by interactions between neurons and glial cells.
Neurosteroids have many important actions. Allopregnanolone activates neuronal GABAA receptors having anxiolytic, sedative, sleep-inducing, and anticonvulsant effects. Benzodiazepines, alcohol, and γ-hydroxybutyrate increase brain levels of allopregnanolone. Thus, these drugs may potentiate GABAergic transmission directly and also by increasing allopregnanolone. Pregnenolone sulfate, DHEA, and DHEA sulfate (DHEA-S) are inhibitory, noncompetitive modulators of GABAA and positive modulators of N-methyl-d-aspartate (NMDA) receptors by facilitating calcium influx. Estradiol inhibits NMDA receptors. Neurosteroids acting on NMDA are implicated in cognition, neuroprotection, and neurotoxicity. While pregnenolone sulfate is excitotoxic to cortical and retinal cells, DHEA and DHEA-S have neuroprotective effects against glutamate toxicity. However, the exact mechanisms of such neuroprotective effects are not clear.
Neurosteroids have been implicated in many neurologic and psychiatric disorders, including epilepsy, neurodegenerative diseases, schizophrenia, and depression. For example, depression is associated with reduced levels of allopregnanolone in cerebrospinal fluid. Antidepressant treatment with fluoxetine increases allopregnanolone levels [49]. There is also some evidence that DHEA helps in the treatment of depression. The beneficial effect of DHEA correlates with a decrease in glucocorticoids, which are increased in depression.
There is increasing evidence about many important roles of neurosteroids in regulation of vigilance as well. It remains to be studied whether nutritional factors could also play some role in this. DHEA is marketed in pharmacies and health shops. Little is known about possible positive (and, perhaps, also negative) long-term neuropsychiatric effects of nutritional factors that may cause changes in brain neurosteroids.
Role of Neurohormetic Phytochemicals
Several lines of evidence have shown that diets rich in fibers, vegetables, and fruits are associated with reduced risk of cardiovascular disease and many neurologic diseases [50]. There is also evidence that a vegetarian diet helps in weight control, and in this way prevents obesity and occurrence of obstructive sleep apnea. As stated previously, rapidly absorbed low-fiber carbohydrates induce sleepiness. Therefore, a vegetarian diet with a lot of fiber and slowly absorbing “good” carbohydrates is better for staying alert during waking time. Some of the positive effects are explained by antioxidant effects of different phytochemicals, but there is also evidence that some effects may be due to subtoxic effects of some neurotoxic molecules in the gut.
Hormesis refers to a paradoxical process in which low doses of a given toxic substance, or radiation, induce beneficial effects while larger doses of the same substance are toxic to cells and organisms [51–53]. Examples of endogenous molecules with neurohormetic actions are nitric oxide, carbon monoxide, glutamate, and calcium. Neuroprotective natural substances include α-tocopherol, lycopene, resveratrol (red grapes, red wine, peanuts, and soy), sulforaphanes (broccoli), catechins (green tea), allicin and allium (garlic), curcumin (turmeric), hypericin (in St. John’s wort), and many others. In randomized clinical trials, hypericin (Hypericum extracts) has shown clinical efficacy comparable with the efficacy of some commonly used antidepressants, including citalopram, paroxetine, and fluoxetine [53–60]. This is of interest to sleep specialists because antidepressants are also used in treating insomnia, especially if it is thought to be associated with underlying depression. Much more research is needed to find out if natural substances affect sleep–wake behavior.
Hot spices may disturb sleep, perhaps by a neurohormetic action. In one study on six young men, tabasco and mustard in the evening reduced slow-wave and stage 2 sleep, reduced total time awake, and prolonged sleep onset. The spicy food in the evening elevated body temperature during the first sleep cycle. It is possible that capsaicin affects sleep by its effects on body temperature [61].
Caffeine, Adenosine, and Sleep
Caffeine, used mainly in the form of coffee, is the world’s most common psychoactive drug. Caffeine is present in coffee, tea, cola, energy drinks, and chocolate, and it induces wakefulness. Its stimulant properties depend on its ability to reduce adenosine transmission in the brain. Caffeine acts as an antagonist to adenosine A1 and especially to adenosine A2 receptors [62]. Huang and collaborators found in knockout mice that caffeine increased wakefulness in both wild-type mice and A1 receptor knockout mice, but not in A2a receptor knockout mice. Thus, caffeine-induced wakefulness may be due mainly to its effects on adenosine A2a receptors [63]. Porkka-Heiskanen and her collaborators [64–66] have also recorded human sleep electroencephalograms (EEGs). The longer the previous wakefulness period is, the longer and deeper is the following sleep. The inhibitory neuromodulator adenosine is one promising candidate for a sleep-inducing factor. Its concentration is higher during wakefulness than during sleep, it accumulates in the brain during prolonged wakefulness, and local perfusions as well as systemic administration of adenosine and its agonists induce sleep and decrease wakefulness. The hypothesis is that adenosine accumulates in the extracellular space of the basal forebrain during wakefulness, increasing the sleep propensity. The increase in extracellular adenosine concentration decreases the activity of the wakefulness-promoting cell groups, especially the cholinergic cells in the basal forebrain. When the activity of the wakefulness-active cells decreases sufficiently, sleep is initiated. During sleep, the extracellular adenosine concentrations decrease, and thus, the inhibition of the wakefulness-active cells decreases, allowing awakening and a new wakefulness period [65].
In addition to coffee, caffeine is found in tea, cola drinks, energy drinks, and chocolate. Theobromine is also present in large quantities in chocolate. Dark chocolate is stimulating; 100 g of 70 % chocolate corresponds to 1–2 cups of coffee depending on strength of the coffee and size of the cup. Caffeine is a CNS stimulant. Its actions are variable among different people. Caffeine is absorbed rapidly, and peak activity is achieved in 30–60 min. The duration of action is usually 4–6 h, but in elderly subjects with slower metabolism, the duration may be up to 16–20 h. Insomniacs are usually advised to avoid coffee after 6:00 pm, but in some sensitive persons with insomnia, coffee at noon may disturb falling asleep in the evening.
One cup of coffee contains 75–125 mg of caffeine. A large amount of caffeine, usually over 300–500 mg (depending on individual sensitivity), causes restlessness, anxiety, trembling, tinnitus, and feelings of euphoria/delirium. Everyday use of more than 500 mg of caffeine leads to caffeinism with insomnia, fatigue, and different psychosomatic symptoms. It has been estimated that perhaps 10–20 % of coffee drinkers have caffeinism. Chronic coffee drinkers have often developed tolerance to caffeine, and some people may drink more than 10 cups of coffee daily. They have withdrawal symptoms if they do not have their coffee. Coffee is a well-known factor disturbing sleep [67–69]. Two or three cups of coffee (or in sensitive persons just one cup) before bedtime is followed by difficulty falling asleep and restless sleep.
Landolt et al. [70] administered 200 mg of caffeine in the morning and analyzed the sleep stages and EEG power spectra during the subsequent night in nine healthy men. They also measured caffeine levels in saliva, which decreased from a maximum of 17 μmol/L 1 h after intake to 3 μmol/L at 23:00 in the evening. Compared to placebo, sleep efficiency and total sleep time were significantly reduced after the morning intake of caffeine [70]. Slow-wave sleep decreases and amount of stage 1 sleep usually increases after drinking coffee. Insomnia and RLS are more frequent among habitual coffee drinkers than among others. Paradoxically, in some persons, one or two cups of coffee may ameliorate quality of sleep. The reason can be behavioral conditioning, but it is also known that caffeine has a stimulating effect on both breathing and cardiac function. In patients with RLS, symptoms worsen by drinking a lot of coffee. Missak postulated that the human body—in cases of iron deficiency and chronic renal failure—may produce a substance similar to caffeine [71].
Epidemiologic studies have provided evidence that caffeine, an adenosine receptor antagonist, reduces the risk for PD [72–75]. There are indications of specific interactions between striatal adenosine A2a and dopamine D2 receptors. In one study, the dopaminergic effects of caffeine were examined with [11C]raclopride positron emission tomography in eight healthy habitual coffee drinkers after 24 h of caffeine abstinence. Compared to oral placebo, 200 mg of oral caffeine induced a 12 % decrease in midline thalamic binding potential (p < 0.001). These findings indicate that caffeine has effects on dopaminergic neurotransmission in the human brain, which may be different in the striatum and the thalamus [73]. Caffeine and other A(2A) receptor antagonists are also potentially neuroprotective, which may explain some of their favorable effects [74–76].
Glucose, Lactate, and Glial Cells
The main sources of energy for the brain are glucose and lactate. According to Magistretti’s theory, lactate may be the most important source of neuronal energy [7, 9, 77]. Most of the glucose from brain capillaries enters glial cells through the blood–brain barrier. In astrocytes, glucose is metabolized into lactate by glycolysis. The rate of lactate metabolism depends on brain activity and use of oxygen. Mitochondrial function should be intact for proper functioning.
Besides the heart, the CNS is the only part of the body that may have both functional and structural changes after hypoglycemia. However, the brain resists fasting better than other organs. The brain uses glucose at a rate of about 70 mg/min and contains about 1–2 g of glucose, which is sufficient for about 90 min if no more glucose is obtained from circulation. There are no strong associations between behavioral symptoms and plasma glucose levels, but fatigue and other symptoms usually occur when glucose levels are below 1.5–2 mmol/L. Common reasons for hypoglycemia are overdose of diabetic medications, high insulin levels, and severe malnutrition.
Glutamate, adenosine, and GABA have crucial roles in controlling brain energetics and lactate formation in the astrocytes. Monocarboxylate transporters (MCT1 and MCT4 in astrocytes and MCT2 in neuronal cells) are needed in the transfer of lactate from astrocytes to neurons [9, 78]. It is important to note that alpha- and beta-adrenoceptors exist in the surface of astrocytes [79]. Norepinephrine activates these adrenoceptors and stimulates glycogenolysis in astrocytes, which then produce lactate from glucose and release it to provide necessary energy for neurons [9, 80].
These theories also fit findings of Haydon and collaborators about the importance of glial cells in control of synaptic transmission, neuronal activity, and sleep [81–83]. Also Newman, Montana et al., and Zhang et al. have pointed out the role of astrocytes in regulation of synaptic activity [84–86], and, as Hertz and Zielke have written, astrocytes may be the “stars of the show” [87]. All functions of glutamate are regulated by astrocytes. Glutamate is the most important excitatory brain transmitter, and all is dependent on the ability of astrocytes to produce glutamate and glutamine [87]. The studies by Aubert and his collaborators of CNS lactate kinetics support these findings, indicating that neurons are lactate-consuming cells, whereas astrocytes are lactate producers [88]. Lactate production by the astrocytes increases with enhanced glutamatergic activation, and consumption of lactate by neurons also increases as a result of glutamatergic activity [8, 88]. It may well be that many neurologic and psychiatric diseases will turn out to be mainly glial diseases. This may also be true for fatigue and sleepiness. One of the main roles of sleep is to ensure sufficient energy for waking brain activity. To understand this better, the glial–neuronal network should be investigated, rather than concentrating our research efforts on neuronal synaptic relationships. If neurons do not have enough food (energy) of proper quality, they may starve and will not function normally [9].
In sum, the astrocyte-neuron lactate shuttle (ANLS) model, as Pellerin and Magistretti call it, can now be recognized as a major theory of brain energetics. The ANLS model helps to understand also neuronal plasticity, neurodegeneration, memory consolidation, and functional brain imaging [9]. The relations of sleep disturbances (sleep deprivation, fragmented sleep) and in the regulation of the ANLS need to be studied in more detail.
Meals, Dietary Nutrients, and Sleep
Effect of different nutrients on sleep has been studied in a large survey of 4500 people. Use of the following nutrients was associated with shorter sleep latency: alpha carotene, selenium, dodecanoic acid, and calcium. For example, the following nutrients were associated with better sleep and less awakenings during sleep: avoiding excessive salt, eating carbohydrates, use of vitamin D, dodecanoic acid, and lycopene [89]. Nonrestorative sleep was associated with butaneoic acid, lack of calcium, lack of vitamin C, lack of water, excess moisture, and excess cholesterol. Daytime sleepiness was associated with increased moisture, more caffeine/theobromine, less potassium, and less water [89]. These different elements will be handled in more detail below.
Rapidly Absorbing Carbohydrates, Large Meals, and Tryptophan
The hedonic regulation of food intake is powerful and it may be a key element in development of obesity. In normal weight subjects, the hedonic eating behavior is in balance with the homeostatic control of energy intake [90]. In obesity, the hedonic drive for eating may outweigh the homeostatic control of eating, leading to excess of energy intake. In a French study, rats were allowed to choose between water sweetened with saccharin and intravenous cocaine. Almost all (94 %) rats preferred saccharin water instead of cocaine. Rats preferred also natural sugar (sucrose) over cocaine. In order to abolish this finding, high doses of cocaine, causing intoxication, were needed [91]. Sugar and sweet food can induce reward and craving in humans that are at least comparable in magnitude to those induced by addictive drugs [92].
Clinicians treating narcoleptics know that rapidly absorbing carbohydrates induce sleepiness in the afternoon among their patients. What about the effect of carbohydrates in normal subjects? Most studies in which different foods have been compared with each other show that carbohydrates at lunch induce afternoon sleepiness more than proteins. In a study by Spring et al. [93], normal adults consumed either a high-protein or high-carbohydrate meal. Two hours later, their mood and performance were tested. Women, but not men, reported greater sleepiness after a carbohydrate as opposed to a protein meal. Men, but not women, reported greater calmness after a carbohydrate as opposed to a protein meal. Age of subjects had an effect on the response to meals. When meals were eaten for breakfast, persons older than 40 years felt more tense and less calm after a protein-rich than after a carbohydrate-rich meal. Older subjects did not like a morning protein meal as much as a carbohydrate meal, but objective performance was impaired more after a carbohydrate-rich lunch than after a protein-rich lunch. Sustained selective attention, as measured by dichotic shadowing, was impaired in the afternoon after consuming a high-carbohydrate lunch. In sum, these findings suggest negative effects on concentration after a high-carbohydrate, low-protein lunch [93].
In another study, fatiguing effects of lunch after carbohydrate-rich meals were compared to other types of meals [94]. Observed behavioral changes were correlated with changes in plasma glucose, insulin, and amino acids. Only the carbohydrate meal significantly increased fatigue, which could not be attributed to hypoglycemia because plasma glucose remained elevated. The afternoon fatigue began when the carbohydrate meal elevated plasma tryptophan but ended even though the ratio remained elevated. According to Christensen, fatigue after a high-carbohydrate lunch cannot be explained only by reactive hypoglycemia or sweet taste and could partially be explained by the hypothesis that fatigue parallels an elevation of tryptophan and effects endogenous opioids [95]. Associations among a carbohydrate-rich meal, an increase of tryptophan, and feelings of fatigue have also been confirmed in other studies [96].
Rapid increase in insulin and its effect on tryptophan are related to symptoms of fatigue. In particular, after rapidly absorbing carbohydrates as well as after a glucose intolerance test, insulin is rapidly increased. This rapid increase in insulin is associated with an increase in the ratio of tryptophan to large neutral amino acids (LNAAs) causing abnormal neuroglycopenic symptoms. In practice, this may be seen as “sugar drunkenness” [96]. These findings have been confirmed by Cunliffe et al., who found that subjects consuming a pure carbohydrate meal were more tired with feelings of fatigue and had had slower reaction times. A general feeling of mental or central fatigue was noticed after a pure isocaloric fat meal. The ratio of tryptophan to LNAAs was decreased after a pure fat or mixed meal and rose after a pure carbohydrate meal. The authors concluded that central and subjective fatigue after carbohydrate intake may be related to an increase in tryptophan relative to other competitive amino acids. Pure fat intake may also have a negative influence on CNS arousal [97].
Wheat, Starch, Amylopectins, and Postprandial Sleepiness
Celiac disease is well-defined autoimmune disorder. It occurs in genetically predisposed people. In celiac disease, ingestion of gluten leads to damage of the small intestine. Gluten is a protein that is found in rye, barley, wheat, and foods made with these grains. Patients with celiac disease suffer from abdominal bloating, pain, gas, diarrhea, pale stools, and weight loss. They may have skin rash due to dermatitis herpetiformis, iron deficiency anemia, and tingling sensations in the legs. They may mimic symptoms of restless legs. The prevalence of celiac disease is about one percent. Celiac disease may be a cause of a sleep disorder, but gluten may not be the most important factor in grains, that may cause sleepiness.
Wheat, other grains, potatoes, and other sources of starch may cause sleepiness also directly by increasing blood glucose and insulin levels. It is to be noted that wheat bread may cause a faster and higher increase in blood sugar than ingestion of sugar. The amylose-amylopectin ratio is one of the factors explaining glucose- and insulin-response differences. Foods with higher amylose content are accompanied by a lowered metabolic response and lower increase in glucose and insulin than foods with higher amylopectin content. Of the starchy foods, legumes with high fiber content elicit a much lower postprandial response of glucose and insulin, and hence less postprandial sleepiness than food rich in starch [98, 99]. Legumes are also good sources of high-quality proteins. The amount of amylose and amylopectins depends on many factors. For example, macaroni has a lower amylose-amylopectin ratio than spaghetti, and it increases blood glucose more than spaghetti.
The amount of food eaten is also related to feelings of sleepiness. Solid foods cause more sleepiness than liquid foods. Valuable studies have also shown that the larger the meal, the sleepier the person is afterward [100]. It may be that filling the stomach with plenty of food might have a stronger effect than different constituents of food per se [101].
Eating in the Evening and Night Sleep
Sleepiness and fatigue after lunch are usually unwanted. For this reason, people wanting to avoid postlunch sleepiness should avoid rapidly absorbing carbohydrates and large meals. Needless to say, alcohol during lunch increases sleepiness. On the contrary, in the evening, the sleep-facilitating effects of carbohydrates may be beneficial. Recently, Afaghi et al. [102] explored the effect of the glycemic index (GI) on sleep in 12 healthy men. Their subjects were administered standard, isocaloric (3212 kJ; 8 % of energy as protein, 1.6 % of energy as fat, and 90.4 % of energy as carbohydrate) meals of either Mahatma (GI = 50 [low]) or Jasmine (GI = 109 [high]) rice 4 h before their usual bedtime. On another occasion, the same high-GI meal was given 1 h before bedtime. Sleep onset latency shortened significantly after a high-GI meal compared with a low-GI meal. The high-GI meal given 4 h before bedtime had better action on sleep latency than the same meal given just 1 h before bedtime (9.0 ± 6.2 vs. 14.6 ± 9.9 min; p = 0.01) [102]. This finding confirms earlier studies showing that a (light) carbohydrate-rich diet during dinner may be beneficial for sleep.
Adding tryptophan may enhance the action of carbohydrates. A controlled double-blind study lasting five weeks evaluated whether eating of cereals enriched with nutrients facilitating sleep could help to improve infants with sleep disorders. A “sleep facilitating cereal” product containing 225 mg tryptophan, 5.3 mg adenosine-5′-P, and 6.3 mg uridine-5′-P per 100 g of product. As compared to similarly tasting cereals without added nutrients, the administration of enriched cereals led to an improvement in sleep [103]. In another study, the same group found that cereals enriched with tryptophan (60 mg tryptophan in 30 g cereals per dose) eaten at both breakfast and dinner increased actigraphically measured sleep efficiency, actual sleep time, immobile time, and decreased total nocturnal activity, sleep fragmentation index, and sleep latency [104].
According to common knowledge, heavy meals late in the evening disturb night sleep. Driver and collaborators studied effects of different types of evening meals and of a 10-h fast (no evening meal) on body temperature and night sleep. Seven healthy people participated. Meals of varying energy content and episodes of sleep influence body temperature. Lower rectal temperatures were measured during the fast than following the meals. Higher energy content of the evening meal associated with higher body temperatures at night. No differences were observed in the subjective or polysomnographic sleep measures [105]. A transient heavy evening meal, 2–3 h before going to bed, or a 10-h fast may not alter significantly sleep of previous good sleepers. However, the higher body temperature after heavy meal reflects higher autonomic activity, and there is evidence for example in insomniacs and in patients with narcolepsy that higher core body temperatures (and lower peripheral temperatures) are associated with less sleepiness and lower quality of night sleep [106, 107].
Contents of meals may also be related to the circadian type. In a Japanese study, evening-type persons, as compared to morning-type persons, had significantly lower energy-adjusted intake of protein, calcium, magnesium, zinc, vitamins (D, riboflavin, B6), and vegetables and with a higher intake of noodles [108]. In other words, evening chronotype may be associated with inadequate dietary habits such as low vitamin and mineral intake.
Postlunch Fatigue
A siesta after lunch is still common in some parts of the world, although it has disappeared from most developed countries. Also in developed countries, a feeling of tiredness in the afternoon is common. According to Bell [109], 71 % of narcoleptics and 9 % of healthy adults suffer often or always from postprandial sleepiness. The vigilance level has a bimodal pattern: Alertness is at its lowest level after midnight, and there is another dip in alertness occurring during the afternoon independent of whether one has been eating or not. The effects of different types of meals have been studied. There is evidence that heavy meals cause more fatigue than light meals. There is also agreement that alcohol during lunch causes sleepiness in the afternoon. In clinical settings, there is some evidence that rapidly absorbing carbohydrates in particular cause more sleepiness and fatigue after lunch than slowly absorbing carbohydrates and proteins. Eating carbohydrates at breakfast, on the contrary, may not increase daytime sleepiness. In another study, subjects also tended to feel more sleepy and fatigued 2–3 h after a high-fat, low-carbohydrate breakfast than after low-fat, high-carbohydrate breakfast [110].
Obesity and Sleep
Obesity is an epidemic, especially in Western countries. It is believed that the epidemic is a consequence of eating too much food with energetic value combined with a decrease in physical activity. This is probably true, but it does not explain everything. In the medical world, research on cardiovascular diseases has been one of the primary foci. High-cholesterol levels are associated with an increase in cardiovascular morbidity. Therefore, the food industry has been developing foods with no cholesterol, and people are advised to avoid butter and fats. However, the food industry has also been developing more and more foods and soft drinks with high sugar content (e.g., Coca-Cola, Pepsi-Cola). Despite eating less, fat people become more and more obese, suggesting that other factors must be involved.
From a physiologic standpoint, body fat is deposited in excess amounts when caloric intake exceeds caloric expenditure [111]. Most of our calories are expended in a resting state. We know that obese patients with sleep apnea have difficulty losing weight. It has been questioned whether obese people have a lower resting metabolism than lean people. Are obese people like diesel motors? Using whole-body direct calorimetry and other methods that enable measurement of basic metabolism, researchers have shown that obese subjects often have lower resting metabolic rates that persist after weight reduction, that people with a high genetic propensity for obesity often show this decreased resting metabolism before they become obese, and that these people are more likely to regain their weight after weight reduction. The mechanism of the low resting metabolic rate in many obese people is not known [111]. Eating less food with high energy content is needed to lose weight. Also, avoiding rapidly absorbing carbohydrates should be an element in weight reduction. Avoiding fats and increasing exercise do not help if an obese subject continues to drink sweet soft drinks and eat a lot of potatoes, white bread, or sweets. It is important to note that, with excess caloric intake, carbohydrates convert to fat. Citrate from the citric acid cycle diverts from mitochondria into cytosol for fatty acid synthesis. After a meal rich in carbohydrates, insulin release from the pancreas causes a 30-fold increase in glucose transport into adipocytes.
Several prospective epidemiological studies have shown that there is a relationship between sleep length, sleep quality, and obesity. Both short sleep (too short sleep relative to individual need of sleep) and poor sleep quality increase the risk of developing obesity, and consecutively type 2 diabetes and metabolic syndrome [112–117]. In experimental studies, sustained sleep fragmentation in mice affects brain temperature, food intake, and glucose tolerance [118]. Different neuronal networks and transmitters are involved as discussed earlier in this chapter.
Dietary Patterns and Sleep
Effect of Fasting on Sleep
Effects of fasting on sleep length differ depending on the study. Fasting was associated with shorter sleep in some human studies and with increased sleep in others. Results of studies on the effect of fasting on sleep architecture are somewhat divergent. Fasting, resulting in decreased energy intake, seems to be associated with an increase in slow-wave sleep, and a decrease in rapid eye movement (REM) sleep and sleep stages 1 and 2. In the earlier studies, this was explained by the restorative function of slow-wave sleep [119, 120]. In the 1980s, it was thought that sleep was mainly for the brain and had little restorative value [121]. It is now known that sleep not only is important for the brain, but also has a restorative function [13, 122]. The relationship is complex. In experimental studies, caloric restriction protects the brain against aging and disease, while restriction of sleep has an opposite effect. Markers of oxidative stress were lower in cultured neuronal cells treated with caloric restriction serum compared with those treated with ad libitum serum [123].
Muslims fast during the ninth month (Ramadan) of the Islamic Hijra calendar. Hijra is a lunar calendar, and Ramadan occurs at different times in the seasonal year over a 33-year cycle. Fasting during Ramadan occurs daily from sunrise to sunset. The effects of the intermittent type of fasting during Ramadan differ from those of continuous fasting. In one study, the main finding was that during Ramadan, evening sleep latency was increased and nocturnal sleep architecture was modified. Slow-wave sleep and REM sleep decreased during Ramadan. The effects of Ramadan fasting on nocturnal sleep have been explained by changes in drinking and meal schedule, rather than an altered energy intake, which may be preserved [124]. In another study, Ramadan diurnal fasting induced an increase in subjective and objective daytime sleepiness, measured by MSLT, associated with changes in diurnal rectal temperature [125]. Later bedtime during Ramadan shortens consistently night sleep by about an hour or more, which explains probably a part of the increased daytime sleepiness [126, 127].
Ketogenic Diet and Sleep
The ketogenic diet has been used for a long time in the treatment of severe epilepsy. It is efficient but it is usually only a temporary solution. Some efforts have been made to develop other diets that are based mainly on lowering amounts of carbohydrates in order to lower blood sugar levels without inducing marked metabolic ketosis. Early results have been promising. Again, these diets are based on Magistretti’s theories and on theories about the role of astrocytes [7, 9, 85, 128]. Hypocretin and MCH cells are sensing glucose levels. Lower blood glucose is associated also with higher hypocretin and lower MCH levels increasing alertness [23]. It is possible that in fact brain cells, especially glial cells, perform better when glucose levels are lower. This is followed also by better synaptic function and less paroxysmal activity.
According to theories on brain energetics, the ketogenic diet could help people to sleep better and have better alertness during the daytime. Hallbook et al. [129] have published first results supporting this idea. They examined 18 children with treatment-resistant epilepsy. All children had polysomnography studies before starting a ketogenic diet and after three months on the diet. Eleven children were evaluated after staying on the diet for one year. Seizures were controlled with the diet, and it was associated with decreased total sleep and total night sleep. There was no change in amount of slow-wave sleep, and REM sleep was increased. Stage 2 sleep was decreased (P = 0.004), and sleep stage 1 was unchanged. There was a significant correlation between increased REM sleep and improvement in quality of life.
Brown has formed an interesting hypothesis to explain the positive mental effects of a low-carbohydrate diet [130]. Better vigilance, feelings of well-being, and feelings of mild euphoria have been attributed to increased production of ketone bodies, which can replace glucose as an energy source for the brain, as Magistretti has postulated [7, 9]. Brown noted that one of these ketone bodies, β-hydroxybutyrate (BHB), is an isomer of γ-hydroxybutyrate, which is used as treatment for alcohol and opiate dependence and also for narcolepsy with cataplexy. Brown hypothesized that the positive mental effects with fasting and a low-carbohydrate diet may be due to shared actions of BHB and GHB on the brain. BHB, like GHB, is a weak partial agonist for GABAB receptors [130].
Ketogenic diet has many adverse effects, and it may not even be necessary to achieve clinical results. In a study by Johnson et al., ketogenic and nonketogenic low-carbohydrate diets were equally effective in reducing body weight and insulin resistance, but the ketogenic diet was associated with significantly more adverse effects [131].
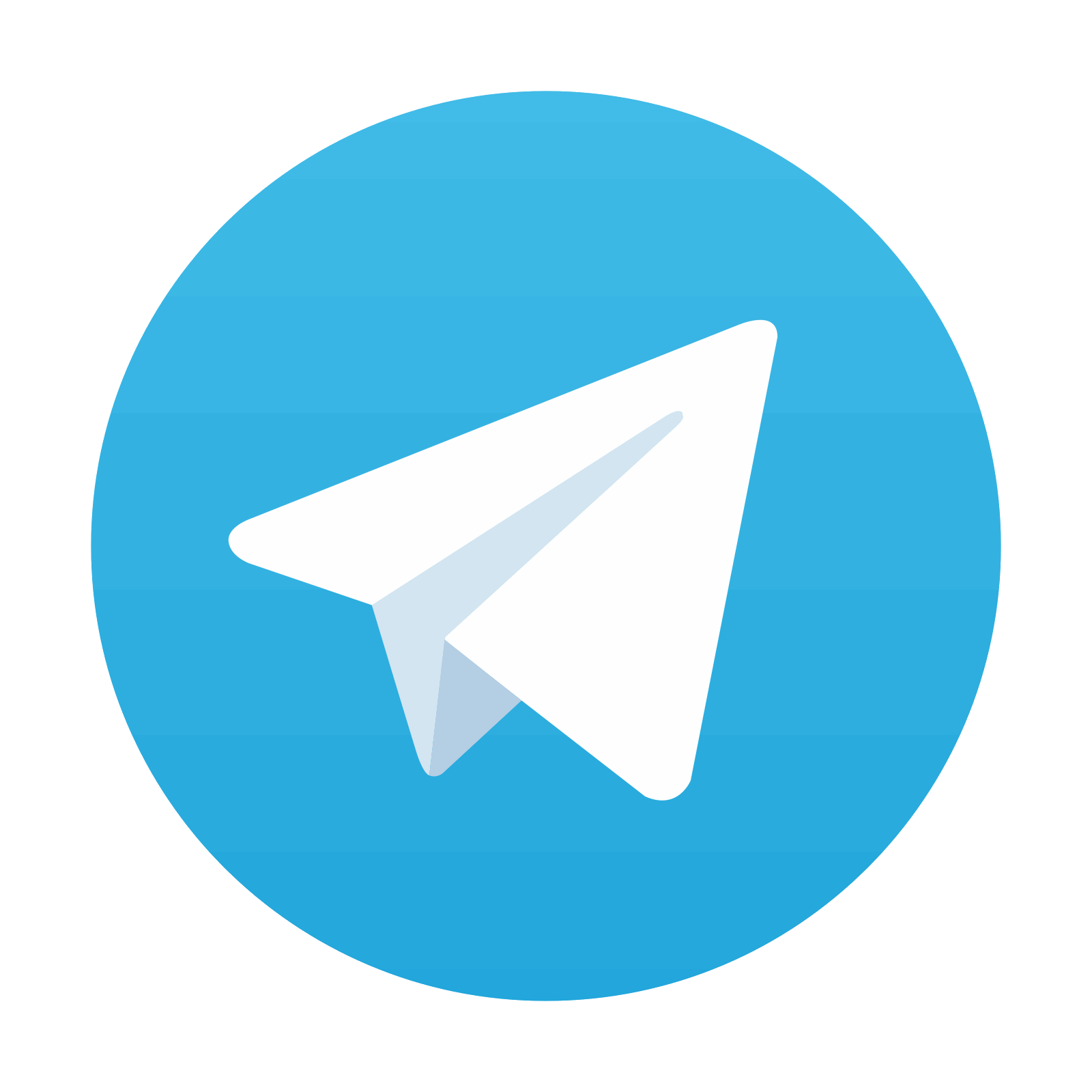
Stay updated, free articles. Join our Telegram channel

Full access? Get Clinical Tree
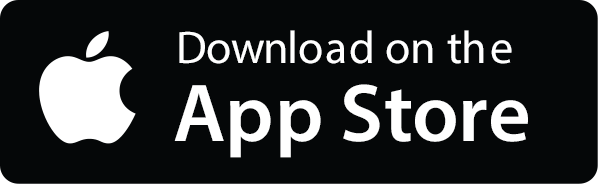
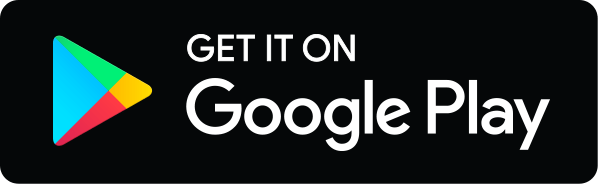