Obesity
Johannes Hebebrand
Definition and Diagnosis
Overweight is present if body weight adjusted for height exceeds a specified cutoff; obesity implies an excessive fraction of total body weight comprised of fat mass. In theory at least, obesity can only be diagnosed if fat and fat-free mass are determined. Various models and indirect methods for estimation of body composition have been developed, all of which are imperfect and require a number of assumptions, including age-specific considerations. For research purposes, body composition in children has been determined using techniques such as total body water, dual energy X-ray absorptiometry (DEXA), total body electrical conductivity, and total body potassium. By contrast, in clinical studies bioelectrical resistance and skinfold measurements have been employed instead (1).
In clinical practice, however, the BMI, defined as weight in kilograms divided by height in meters squared (kg/m2), has become widely accepted as the optimal weight-height index throughout childhood and adolescence and as a surrogate measure of adiposity (2). Indeed, the correlations between BMI and percent body fat as determined by DEXA are high (3,4). Nevertheless, a high fat free mass can, as in the case of some athletic individuals, also entail a high BMI.
The developmental pattern of BMI is similar to that of fat mass. Typically BMI increases steeply during the first 6 months of life primarily due to an increase of fat mass, to then decline until age 6 to 8 years as a result of a strong relative increase in height (Figure 5.7.3.1 a,b). The time point during childhood at which BMI again increases has been termed adiposity rebound; the earlier it occurs, the higher the risk of subsequent development of obesity and vice versa (5). BMI rises throughout the
rest of childhood and adolescence; this rise continues— albeit more slowly— during adulthood. In males the rise during adolescence is on average largely due to an increase in fat-free mass; percent body fat remains stable at approximately 15 to 18%, whereas in females percent body fat increases from a prepubertal value similar to that of boys to a value of 20 to 25% after puberty (6).
rest of childhood and adolescence; this rise continues— albeit more slowly— during adulthood. In males the rise during adolescence is on average largely due to an increase in fat-free mass; percent body fat remains stable at approximately 15 to 18%, whereas in females percent body fat increases from a prepubertal value similar to that of boys to a value of 20 to 25% after puberty (6).
![]() FIGURE 5.7.3.1. Body mass index-for-age percentiles for boys (A) and girls (B) for the U.S. population (http://www.cdc.gov/growthcharts). |
Birth weight is minimally correlated with BMI at age 35 (r = 0.1). Accordingly, an overweight infant is only at a minimally elevated risk for obesity in adulthood provided that parental obesity is not present (Figure 5.7.3.2). The greatest variation in rates of weight gain is seen in the first 1 to 2 years of life, when infants may show significant “catch-up” or “catch-down” growth as a consequence of intrauterine restraint or enhancement of fetal growth. By age two, growth usually follows the genetic trajectory (7). Small-for-date babies who rapidly gain weight after birth (catch-up growth) are at an elevated risk for adult obesity and cardiovascular disorders (8). At age 10 BMI is correlated with BMI at age 35 in the magnitude of 0.35; during late adolescence BMI tracks well into adulthood (r = 0.7) (9). If a child is obese the risk of persistence into adulthood increases with the degree of adiposity (10), age of the child, and parental obesity (Figure 5.7.3.2).
The strong age dependency of BMI implies that an adequate interpretation of the index of an individual child requires knowledge of the BMI distribution of same aged and sexed children. Frequently, centile curves are based on BMI values of a large and ideally representative sample; older children and parents are readily able to grasp this epidemiological concept as having good face value for educatory purposes. BMI standard deviation scores (BMI-SDS) or Z-scores are commonly employed to further differentiate extreme overweight or underweight (> 99th and < 1st centile).
In adults, overweight, obesity and extreme obesity are diagnosed if BMI is ≥ 25, ≥ 30 and ≥ 40 kg/m2, respectively; (11) these cutoffs were chosen because they mark increments in risks for complications of elevated BMI. For children and adolescents, however, there is no clear-cut consensus as to what cutoff should be used to define overweight and obesity; all existing definitions are statistically rather than risk-based. Himes and Dietz (12) have suggested use of the 85th and 95th centiles; in other countries the 90th and 97th centiles serve as the respective cutoffs. The 85th and 95th centiles, based on nationally representative data from the 2000 growth curves of the Centers for Disease Control and Prevention (CDC) (13) have been recommended for classification of persons as being overweight or at risk of overweight in the United States (Figure 5.7.3.1 a,b; CDC; http://www.cdc.gov/growthcharts). However, these cutoffs are not consistent with those used in adulthood; for example, the centile of an 18-year-old female with a BMI of 30.1 kg/m2— thus fulfilling the adult criterion for obesity— is below the 95th centile.
Use of specific centiles as cutoffs for the definition of obesity additionally requires a consensus as to the reference population. International comparison of the absolute BMI values constituting centiles in the lower to middle range typically reveals only a slight degree of divergence among different Western countries. However, large differences exist in the upper centile range; BMI values corresponding to the 90th or 97th centile are for example substantially higher in the United States of America in comparison to most European countries. Because of secular trends for both height and weight, centiles based on a formerly representative population-based survey usually do not reflect the true BMI distribution of the current population. For instance, the measurement data used to construct the nationally representative CDC Growth Charts were obtained from a series of national health examination surveys conducted by the National Center for Health Statistics from 1963 to 1994 and from supplemental data sources; more recent data were excluded to avoid an upward shift in the BMI-for-age curves (http://www.cdc.gov.growthcharts; see link interactive training modules).
In an attempt to define internationally acceptable centile cutoffs for overweight and obesity, the International Obesity Task Force (IOTF) averaged the national curves from six different countries passing through BMIs of 25 and 30 kg/m2 at age 18 years (14). It remains to be seen if the cutoffs are adopted sufficiently to indeed reach an international consensus of the definition of childhood obesity.
Epidemiology
Physical growth and development are sensitive indicators of the quality of the psychosocial, economic, and political environment. Child growth in terms of height, weight and body composition are widely used indicators of nutritional and health status for both the individual child and the community (15). Three major secular trends have consistently emerged at the population level in industrialized countries: Mean height and BMI have increased, and mean age at puberty has decreased. Increments in height and decrements in age at menarche can be dated back several decades; the increment in BMI roughly began three decades ago. Both body height and age at menarche have recently reached plateaus in several industrialized countries; within a society the achievement of these plateaus typically occurred first among individuals with a higher socioeconomic status (16). These plateau effects indicate that the full genetic potential has been realized under an advantageous environment, or that social conditions have ceased to improve.
Obesity has only recently reached epidemic proportions in several countries worldwide. Currently, approximately 66%, 31%, and 5% of adults over 20 in the United States are overweight, obese, and extremely obese, respectively; (17) it has been estimated that if the recent secular trend continues unabated, obesity prevalence will reach 50% by 2025. Similar trends have been observed in other countries in Asia, Europe, North and South America (18). However, it should be pointed out that slight increments in mean BMI of a population can
translate into substantial elevations of prevalence rates for overweight and obesity.
translate into substantial elevations of prevalence rates for overweight and obesity.
Children and adolescents have also been affected strongly by this secular trend. The National Health Examination Surveys have revealed a four-fold increase in the prevalence of overweight among children aged 6 to 11 and a three-fold increase for adolescents aged 12 to 19 between the 1960s and the most recent survey, conducted between 1999 and 2002 (17). In the 1999–2002 survey 16% of the children aged 6 through 19 years were overweight as defined via a BMI ≤ 95th centile of the surveys in the 1960s. An additional 16% of children were classified as at risk of overweight. In the United States of America, African Americans and Mexican Americans are bearing the brunt of the obesity epidemic (19). Worldwide mean BMI and, as a consequence, prevalence rates for overweight and obesity in children and adolescents have been on the rise in both industrialized and developing countries (20). The most pronounced secular increments in absolute BMI have been detected in the overweight and obese range (21).
A detailed review of the medical consequences of childhood and adolescent obesity is beyond the scope of this chapter (22,23,24). In brief, cardiovascular disorders including hypertension, type 2 diabetes mellitus, dyslipidemia and metabolic syndrome are becoming increasingly prevalent during adolescence as a consequence of the obesity epidemic [e.g., 25]. Childhood and adolescent obesity has been associated with increased mortality even after adjustment for adult BMI (24). Child and adolescent psychiatrists need to be aware of medical complications associated with obesity, including nocturnal enuresis and sleep apnea, both of which can occur in up to 16% of obese children and adolescents (23). Although far less common, pseudotumor cerebri can occur in young obese subjects.
Etiology
Obesity is a truly complex disorder that is caused by several genetic and nongenetic risk factors. In historic terms, the etiology of childhood obesity has been perceived differently over the past 100 years; the pendulum has swung from biological to psychological explanations and back. In the early part of the 20th century, pituitary/hypothalamic dysfunction was assumed to underlie obesity (26). From the 1940s through to the 1970s psychological and psychodynamic aspects were thought to play the most prominent role (27,28). Prader, Labhart and Willi delineated the first syndromal form of obesity in 1956. As of the 1970s the relevance of (psycho)social factors has been discussed (29,30,31,32). At the end of the 1980s and 1990s milestone twin and adoption studies substantiated that genetic factors play a prominent role in body weight regulation (33,34,35). The cloning of the leptin gene in 1994 (36) led to a virtual explosion of biomedical research and marked the introduction of large-scaled molecular genetic studies. The detection of children with leptin deficiency (37) and their successful treatment with recombinant leptin (38) for the first time proved that mutations in a single gene can lead to hyperphagia and obesity in individuals of normal intelligence. The most recent years have viewed a strengthening of the hypothesis that obesity is a neuroendocrine disorder, which results if “obesogenic” environmental factors and a polygenic predisposition act in concert. The genetic predisposition is thought to affect both metabolic and behavioral features. Both public and scientific interest in obesity has witnessed an unparalleled boom over the past 20 years, reflecting the obesity epidemic, the discovery of novel pathways involved in body weight regulation, greater health concerns, the perception of social inequality, and a stronger awareness of the societal norms for body weight.
Genetic Factors
There is a general consensus that parental obesity is by far the strongest risk factor for childhood and adolescent obesity. The risk is influenced by the degree of parental obesity (39) and is further elevated if both parents are obese (Table 5.7.3.1) (40). Several studies have found a stronger effect of maternal than paternal obesity (41). Formal genetic studies have led to the conclusion that the strong predictive value of parental BMI mainly stems from genetic rather than environmental factors (42). Accordingly, older studies that failed to include parental weight as a variable are seriously flawed. For example, the well known finding that parental neglect during childhood predicts obesity in young adulthood (30) was not controlled for parental obesity.
Twin studies (42,43) have produced the most consistent and highest heritability estimates, in the range of 0.6 to 0.9 for BMI. These high estimates apply to twins reared both together and apart. However, only single and comparatively small studies exist for twins reared apart, in contrast to the vast amount of studies pertaining to twins reared together, some of which included thousands of twin pairs; in addition, a substantial number of reared apart twin pairs were not separated immediately after birth.
Except for the newborn period, for which a lower heritability of 0.4 has been calculated (44), age does not affect heritability estimates of body weight to a substantial degree. The influence of the intrauterine environment on birth weight is strong; it is well known that particularly in monozygotic (MZ) twins other anthropometric measurements, such as body height, also correlate less well in infancy than in childhood. The feto-fetal transfusion syndrome observed only in MZ twins contributes to this phenomenon, as it substantially reduces the effect of genetic factors at birth and during infancy. Genetic factors are subsequently able to exert their influence; in school-age children high heritability of BMI already applies. Possibly, the heritability of BMI is maximal (≈ 0.9) during late childhood and adolescence (45).
Adoption and family studies have mostly derived considerably lower heritability estimates of 0.25 to 0.7 (42,46). Twin studies have the advantage of a better control for age effects on BMI and are more valid if nonadditive genetic factors play a larger role in body weight regulation. For an adequate interpretation of the heritability estimates it is noteworthy to point out that both direct and indirect genetic effects are subsumed under the genetic component. If for example both infant twins of a MZ pair are frequently irritable due to a biologically driven hunger (direct genetic effect), frequent feedings by the caretaker ensue (indirect genetic effect); even if the twins are separated at birth, the respective caretakers can be expected to respond similarly.
Another interesting and important aspect of formal genetic studies has been the observation that nonshared environment explains considerably more variance of the quantitative phenotype (BMI) than shared environment. In the large twin study of Stunkard and coworkers (35), which encompassed adult twin pairs reared together or apart, shared environment did not explain variance at all; instead nonshared environment totally explained the environmental component, estimated at 30%. Accordingly, only genetic factors would account for a familial loading with obesity. However, more recent studies indicate that the shared environment might play a more substantial role after all (46); past research may have underestimated common environmental effects on BMI because the designs lacked the power or ability to detect them. Finally, the environment of modern-day societies (easy access to a large variety of cheap and tasty foods, a lifestyle promoting physical inactivity) is quite similar for basically all children, irrespective of the family in which they grow up.
TABLE 5.7.3.1 ASSOCIATION OF RISK FACTORS AND OBESITY AT AGE 7 YEARS. THE AVON LONGITUDINAL STUDY OF PARENTS AND CHILDREN, USING MULTIVARIABLE BINARY LOGISTIC REGRESSION MODELS. OBESITY WAS DEFINED AS A BMI ≥ 95TH CENTILE RELATIVE TO THE 1990 BRITISH REFERENCE POPULATION | ||||||||||||||||||||||||||||||||||||||||||||||||||||||||||||||||||
---|---|---|---|---|---|---|---|---|---|---|---|---|---|---|---|---|---|---|---|---|---|---|---|---|---|---|---|---|---|---|---|---|---|---|---|---|---|---|---|---|---|---|---|---|---|---|---|---|---|---|---|---|---|---|---|---|---|---|---|---|---|---|---|---|---|---|
|
The complexity of the genetic basis of obesity applies both from a metabolic and behavioral perspective (46): Behavioral genetic research has convincingly demonstrated that approximately 50% of the variance of diverse complex quantitative behaviors is genetically determined (47). Both macronutrient intake (48) and activity levels (49) have been shown to be genetically codetermined. Restrained eating, drive for thinness, and other eating behaviors show heritability estimates in the range of 20 to 55% (46). It appears that television viewing may have a heritable component, albeit a small one (50).
Because the gene pool of a population cannot have changed within the past generation, environmental changes affecting both energy intake and expenditure are assumed to underlie the obesity epidemic (18). These changes are presumed to have a major impact because according to the thrifty genotype hypothesis (51) many common genotypes render humans obesity prone: Gene variants facilitating energy deposition as fat have accumulated over time in different species to enhance survival during periods of famine. Put simplistically, “the genetic background loads the gun, but the environment pulls the trigger” (52).
A small genetic contribution to the obesity epidemic cannot be dismissed. For one, overweight females have more children (53). Secondly, the recent rise of social stigmatization of obese individuals (see following) might actually have led to an increase of assortative mating (43). This mechanism could conceivably contribute to epidemic obesity, particularly by affecting the uppermost tail of the BMI distribution via both genetic and environmental factors.
Epigenetic phenomena have also been invoked to contribute to the obesity epidemic. Indeed, it is conceivable that modern-day living might affect methylation patterns of specific genes, which in turn increase the risk of obesity. In line with these considerations young monozygous twins are epigenetically indistinguishable from each other during the early years of life, whereas remarkable differences in their overall content and genomic distribution of 5-methylcytosine DNA and histone acetylation with an effect on gene-expression become evident with increasing age (54). Such environmentally induced changes could have an influence on BMI.
Over the past 10 years mutations in the genes for leptin, leptin receptor, prohormone convertase 1 (PC1), and pro-opiomelanocortin (POMC) (46,55) have been shown to lead to autosomal recessive forms of obesity in humans. All of the mutations are rare and lead to early onset extreme obesity induced by an increased energy intake. Each of these mutations leads to additional phenotypical manifestations, including adrenal insufficiency (POMC), red hair (POMC), reduced or impaired fertility (PC1, leptin and leptin receptor) and impaired immunity (leptin gene). Whereas they seemingly do not affect intelligence, the pleiotropic effects warrant the consideration that these recessive disorders are classified as syndromal forms of obesity— similar to the Bardet-Biedl, Prader-Willi and other genetic syndromes associated with reduced intelligence. The pleiotropic Bardet-Biedel syndrome, of which obesity is a feature, has been shown to have an oligogenic basis (55).
As of 1998, over 60 functionally relevant mutations have been detected in the melanocortin-4 receptor gene (MC4R) (46), which result in a codominantly inherited form of obesity; 2–4% of extremely obese children and adolescents harbor such mutations. Relevant mutations lead to a reduced or total loss of function; the endogenous ligand alpha-melanocyte–stimulating hormone is not able to induce its satiating effect. Adult male and female mutation carriers are 15 and 30 kg heavier
than their sex matched wildtype relatives (56); estimates for children are not available. The effect sizes of these mutations are lower than those of the leptin or leptin receptor gene mutations. Phenotypical effects of MC4R mutations other than obesity have been shown to encompass hyperinsulinemia, elevated growth rate and higher bone density (57). Whether or not these mutations induce binge eating is viewed controversially (58,59,60). Children with MC4R mutations have been shown to eat more at a test meal than obese controls (57).
than their sex matched wildtype relatives (56); estimates for children are not available. The effect sizes of these mutations are lower than those of the leptin or leptin receptor gene mutations. Phenotypical effects of MC4R mutations other than obesity have been shown to encompass hyperinsulinemia, elevated growth rate and higher bone density (57). Whether or not these mutations induce binge eating is viewed controversially (58,59,60). Children with MC4R mutations have been shown to eat more at a test meal than obese controls (57).
A large number of association and linkage studies have been performed in “normal” obesity (46). Whereas many associations have been reported, it is largely unclear which of these represent truly positive findings. Presumably, the power of many of these studies is too low to detect minor genes; false positive findings can result from not correcting for multiple testing. Over 30 genome linkage scans pertaining to obesity and related phenotypes have been performed; as yet unequivocal evidence for the contribution of a specific gene to one of these peaks has not been provided.
Presumably, the effect sizes of most gene variants predisposing to obesity are quite small (polygenic inheritance). Interestingly, the V103I polymorphism in the MC4R, which occurs in 2–7% of individuals of different populations, is associated with a reduced body weight (61,62). Adult carriers are on average 0.5 kg/m2 less heavy than wildtype carriers; the variant also exerts an as yet unquantified small effect in children. To identify and confirm gene variants with minor effect sizes, several thousands of probands need to be assessed.
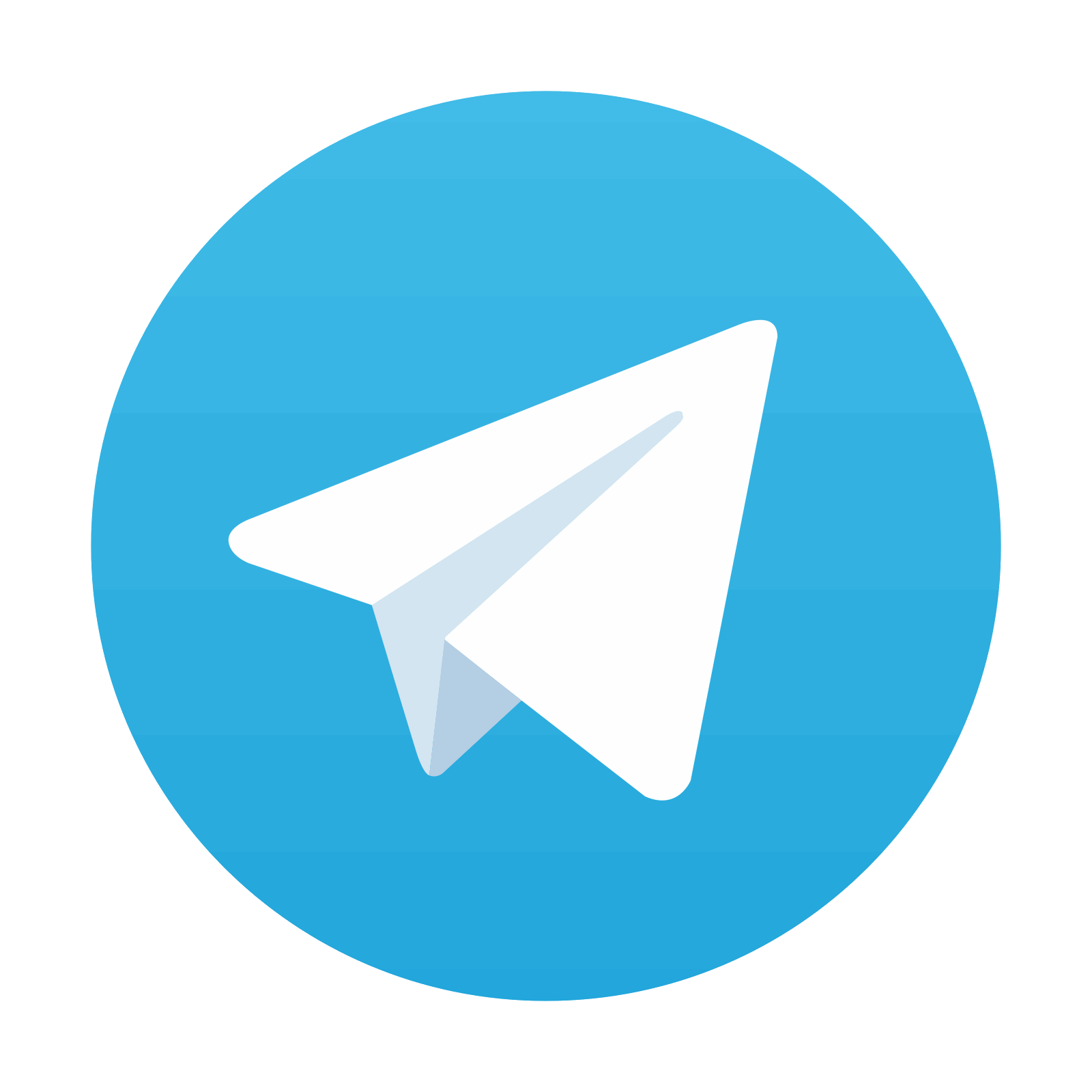
Stay updated, free articles. Join our Telegram channel

Full access? Get Clinical Tree
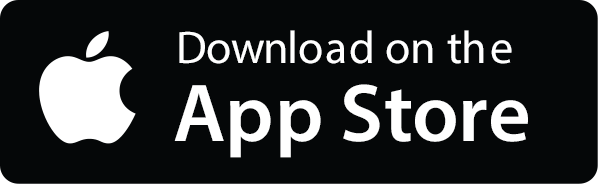
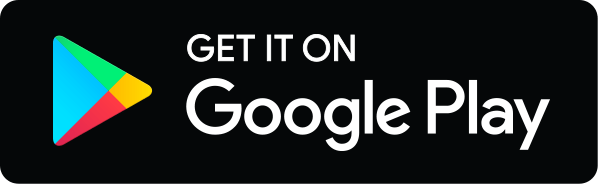