First author
Year
Imaging method
Subjects
Primary outcome measures
Dopaminergic system
Wang et al.
(2001)
[11C]raclopride PET + [18F]FDG PET
10 morbidly OB, 10 NW/OW
DRD2/3 availability
Volkow et al.a
(2008)
[11C]raclopride PET + [18F]FDG PET
10 morbidly OB, 12 NW/OW
DRD2/3 availability and metabolism
De Weijer et al.
(2011)
[123I]IBZM SPECT
15 morbidly OB women, 15 NW/OW women
DRD2/3 availability
Haltia et al.
(2008)
[11C]raclopride PET
12 OW/OB, 12 NW
Dopamine release after glucose injection
Steele et al.
(2010)
[11C]raclopride PET
5 morbidly OB women
DRD2/3 availability after bariatric surgery
Haltia et al.
(2007)
[11C]raclopride PET
12 OW/OB, 12 NW
Dopamine release after glucose expectancy
Wang et al.
(2011)
[11C]raclopride PET
8 OB, 10 OB with BED
Dopamine release after food stimulation
Dunn et al.
(2010)
[11C]raclopride PET
5 morbidly OB women
DRD2/3 availability after bariatric surgery
Chen et al.
(2008)
[99mTc]TRODAT-1 SPECT
50 subjects, BMI 18.7–30.6
DAT availability
Koskela et al.
(2008)
[123I]nor-β-CIT SPECT
16 monozygotic twin pairs, BMI 19.1–31.9
DAT and SERT availability
Van de Giessen
(2012a)
[123I]FP-CIT SPECT
123 subjects, BMI 18.2–41.1
DAT availability
Thomsen et al.
(2013)
[123I]PE21 SPECT
33 subjects, BMI 21.0–49.5
DAT availability
Wilcox et al.
6-[18F]FMT PET
3 OB, 3 OW, 9 NW
Dopamine synthesis capacity
Serotonergic system
Adams et al.
(2004)
[18F]altanserin PET
52 subjects, BMI 24.8 ± 3.7
5-HT2A receptor availability
Erritzoe et al.
(2009)
[18F]altanserin PET
136 subjects, BMI 18.4–42.8
5-HT2A receptor availability
Haahr et al.
(2012)
[11C]SB207145 PET
28 subjects, BMI 20.5–40.0
5-HT4 receptor availability
Erritzoe et al.
(2010)
[11C]DASB PET.
7 OB, 36 OW, 17 NW
SERT availability
Koskela et al.
(2008)
[123I]nor-β-CIT SPECT
16 monozygotic twin pairs, BMI 19.1–31.9
DAT and SERT availability
Kuikka et al.
(2001)
[123I]nor-β-CIT SPECT
7 OB women, 11 OB women with BED
SERT availability
Tammela et al.b
(2003)
[123I]nor-β-CIT SPECT
6 OB women, 6 OB women with BED
SERT availability
It has long been recognized that the brain plays a central role in the regulation of food intake (Mayer and Thomas 1967). Therefore, brain function dysregulations may be an important factor in the etiology of obesity and a possible target for prevention and treatment. Many brain structures participate in food intake regulation (Berthoud 2004, 2007): The caudal brainstem is directly involved in ingestion, digestion, and absorption of food and largely controls autonomic signaling related to the ingestive and digestive processes; the hypothalamus is a key structure in the homeostatic regulation of food intake, which integrates internal state signals and drives pituitary-endocrine and autonomic outputs; corticolimbic regions are important in learning and processing food-related reward and exerting control over food intake with integration of non-metabolic signals. The striatum is an important structure within the corticolimbic system and is primarily involved in motivation for and reward processing of food.
Within the brain, many neurotransmitters play a role in the regulation of food intake, including dopamine, serotonin, noradrenalin, glutamate, gamma-aminobutyric acid (GABA), opioids, and endocannabinoids (Berthoud 2004). The use of anti-obesity drugs, e.g., the serotonergic/noradrenergic blocker sibutramine and the inverse agonist for the cannabinoid-1 receptor rimonabant, has shown that manipulation of the serotonergic/noradrenergic and/or the cannabinoid system affects eating behavior and can induce weight loss. Drugs that increase dopamine and noradrenalin levels, such as methylphenidate and amphetamines, have an anorexigenic effect, whereas dopamine D2 receptor blockers (antipsychotics/neuroleptics) can lead to weight gain. In addition, it has been shown that food can induce dopamine release in the striatum (Bassareo and Di Chiara 1999; Small et al. 2003), therewith modulating the reward value of food (Volkow et al. 2011). Finally, in the key regulatory center for food intake, the hypothalamus, dopamine and serotonin also play an important role (Meguid et al. 2000). In short, it is plausible that (dysregulations of) neurotransmitter systems are associated with obesity either as a factor in the causal pathway or as a consequence of long-term overeating.
Using positron emission tomography (PET) and single-photon emission computed tomography (SPECT) imaging techniques, it is possible to visualize and measure some of these molecular brain processes in vivo in humans. In this chapter, we review studies that focus on neurotransmitter systems in the brain of obese people. Furthermore, we will compare the findings in obesity with findings in substance abuse disorders, because behavioral and neuropharmacological similarities have been hypothesized (Volkow and Wise 2005). A summary of the publications on molecular imaging in obesity reviewed in this section can be found in Table 1.
27.2 Imaging Findings on Neurotransmitter Systems in Obesity
27.2.1 The Dopaminergic System
The majority of the neuroimaging studies on the neurotransmitter systems in obese subjects focus on the dopaminergic system. The earliest imaging study demonstrating abnormalities in the dopaminergic system focused on dopamine D2/3 receptor (DRD2/3) binding in obese humans (Wang et al. 2001). Wang et al. (2001) conducted a [11C]raclopride PET imaging study in 10 morbidly obese subjects (BMI >40 kg/m2) and 10 age-matched controls (BMI <30 kg/m2). They showed that DRD2/3 binding was lower in the striatum of obese participants and also that there was a negative correlation between BMI and DRD2/3 availability in the obese subjects. The finding of decreased striatal DRD2/3 availability in obese subjects was confirmed by the same research group in a sample (BMI mean ± SD: 51 ± 5 kg/m2) that partly overlapped with the previous one (Volkow et al. 2008). Recently, this finding was also replicated in an independent sample of 15 morbidly obese women (BMI mean ± SD: 46.8 ± 6.5 kg/m2) and 15 control women (de Weijer et al. 2011). In this sample, striatal DRD2/3 availability was measured with [123I]iodobenzamide ([123I]IBZM) SPECT, and, in line with previous PET studies, striatal IBZM binding was lower in the obese compared to the control women. Another research team (Haltia et al. 2007) conducted [11C]raclopride PET scans in a sample of normal-weight and overweight/obese subjects (BMI mean ± SD: 33.1 ± 4.4 kg/m2). In a voxel-based analysis, they showed that the overweight/obese participants had significantly lower DRD2/3 binding in left and right striatal and thalamic subregions, although this difference was not significant in the region of interest (ROI) analysis (Haltia et al. 2007). However in this study, the overweight and obese subjects had substantially lower BMIs than the subjects of the three previously described studies, which could be an explanation for the fact that it was not found in the ROI, but only in the voxel-based analysis. Finally, Steele et al. (2010) reported a comparison of [11C]raclopride PET scans in five morbidly obese subjects (BMI >40 kg/m2) to an historical control sample of five females and found no significant difference in DRD2/3 availability between the two groups. However, this study is limited by several factors, such as its small sample size, the 10-year difference in average age between groups, and the use of an external control group. Overall, four out of the five studies reviewed here indicated that the striatal level of free synaptic DRD2/3 is decreased in obesity. Therewith it seems a well-established finding.
Further work investigating the dopaminergic system focused on dopamine release in the striatum. Dopamine release can be induced by a stimulus or pharmacological challenge (e.g., dexamphetamine). Quantifying the displacement of a dopamine receptor binding radiotracer through dopamine is thought to reflect the size of the dopamine release (although it also reflects processes such as dopamine receptor internalization). Based on the hypothesis that the dopaminergic system in obese people is hyporeactive, as suggested by the reward deficiency syndrome theory (Blum et al. 2000), Haltia et al. (2007) tried to demonstrate a blunted dopamine release in overweight and obese people after intravenous glucose administration that serves to mimic recent food intake. To test this hypothesis, they conducted a post-placebo injection and a post-glucose injection [11C]raclopride PET scan in overweight/obese and normal-weight subjects after an overnight fasting period. Any difference measured in DRD2/3 levels between the baseline and post-glucose injection scan could reflect a change in the intrasynaptic dopamine level and thus indicate dopamine release. However, Haltia et al. (2007) were not able to show a significant difference in dopamine release between the normal-weight and overweight/obese (BMI mean ± SD: BMI 33.1 ± 4.4 kg/m2) group. What they did find, however, was a significant gender effect in the response to the intravenous glucose injection: While men showed a decrease in binding of [11C]raclopride to DRD2/3 after intravenous glucose injection, reflecting a dopamine release, women showed an increase in binding. This suggests that increasing glucose levels can have a different effect on the brain depending on gender. Haltia et al. also demonstrated the importance of expectancy for the effect, at least in male participants (Haltia et al. 2008). Using the same sample as before, the team also compared two [11C]raclopride PET scans after placebo injection, one in which the subject was expecting glucose or placebo injection and one with an open placebo expectation. Men showed higher dopamine levels after the placebo injection with glucose expectancy compared to the open placebo injection, revealing an effect of glucose expectancy that seems to facilitate dopamine release. Again, there was no difference between the normal-weight and overweight/obese participants. However, another study showed that within a group of obese subjects, those with binge eating disorder had increased striatal dopamine release after food stimulation (Wang et al. 2011). Furthermore, the dopamine release in the caudate nucleus in response to a food cue in this study correlated with binge eating severity scores in the obese binge eaters. Also in this study, there was no association though between BMI and dopamine release. Overall, studies failed to show a significant relationship between striatal dopamine release and BMI, but increased dopamine release might be associated with binge eating.
It has further been questioned whether weight loss in obese people will lead to a normalization (i.e., increase) of striatal DRD2/3 availability. Bariatric surgery can lead to serious weight loss and influences eating behavior and, thus, may affect dopaminergic neurotransmission in the brain. Recently, two small studies have tried to answer the question whether dopaminergic neurotransmission and DRD2/3 availability might change after bariatric surgery in morbidly obese subjects (Steele et al. 2010; Dunn et al. 2010). Steele et al. (2010) performed [11C]raclopride PET imaging in five female subjects (preoperative BMI >40 kg/m2) before and 6 weeks after laparoscopic Roux-en-Y gastric bypass. They found that DRD2/3 availability increased in four of the five subjects after bariatric surgery, although no statistical test was performed to test significance and the subject with the highest BMI actually showed a strong postoperative decrease in DRD2/3 availability. In a similar study, Dunn et al. (2010) compared DRD2/3 availability before and 6 weeks after bypass surgery in 5 female patients (BMI >40 kg/m2) and found a significant decrease of DRD2/3 availability in several areas of interest (caudate nucleus, hypothalamus, medial thalamus, and amygdala). The results of Steele et al. (2010) and Dunn et al. (2010) clearly contradict each other. It is possible that this is partly due to the use of different tracers, i.e., [18F]fallypride in Dunn’s study, compared to [11C]raclopride in the study by Steele. The small sample sizes of both studies preclude firm conclusions about the effect of weight loss on DRD2/3 availability.
Most imaging studies on the dopaminergic system in obesity have concentrated their attention on the DRD2/3, of which the majority is located postsynaptically (Levey et al. 1993). However, the presynaptic dopamine transporter (DAT) could be equally important, because it regulates the synaptic dopamine levels, in particular for the tonic dopamine levels (Zhang et al. 2009). Therefore, the DAT may also play a role in reward processing of food. Three studies have investigated DAT availability in relation to BMI, so far. Chen et al. reported a negative correlation between BMI and striatal DAT availability, as measured with [99mTc]TRODAT-1 SPECT in healthy subjects (BMI range: 18.7–30.6 kg/m2) (Chen et al. 2008). However, in a monozygotic twin study that applied the more specific ligand [123I]nor-β-CIT SPECT, Koskela et al. (2008) were not able to show a difference in striatal DAT availability between the heavier twin (BMI mean ± SD: 26.8 ± 3.6 kg/m2) and its leaner twin sibling (BMI mean ± SD: 24.5 ± 3.1 kg/m2). Both these studies included only a limited range of BMIs and neither included severely obese subjects. However, a recent study using [123I]FP-CIT SPECT included a large sample with a wider BMI range. In 123 healthy European subjects (BMI range: 18.2–41.4 kg/m2), Van de Giessen et al. (2012a) found that there was no association between BMI and striatal DAT availability. Furthermore, Thomsen et al. (2013) studied a sample that also included severely obese subjects (BMI range: 21.0–49.5 kg/m2) and used the specific DAT radioligand [123I]PE21 and SPECT. They found no correlation between BMI and DAT availability nor a difference in DAT availability between obese and normal-weight subjects. Overall, three out of four studies found no association between BMI and striatal DAT availability, which suggests that striatal DAT levels are not different at high BMI.
Looking at the dopamine system from a slightly different angle, Wilcox et al. (2010) conducted a PET study in 15 healthy subjects (BMI mean: 25.3 kg/m2, 3 subjects with BMI >30 kg/m2) using the ligand 6-[18F]fluoro-l–m-tyrosine (FMT) as a tracer to analyze the capacity of striatal neurons to convert levodopa (l-dopa) to dopamine. The results of this study showed that in obese and overweight subjects, the capacity to synthesize dopamine tended to be lower than in normal-weight controls (although not significant after correction for age and gender). The study also found that the frequency of unsuccessful attempts of dieting and weight loss was negatively associated with the synthesis capacity of dopamine in the dorsal putamen. These findings point towards a downregulation mechanism that limits the available striatal dopamine production in response to overeating.
27.2.2 The Serotonergic System
Apart from the dopaminergic system, only the serotonergic system has been studied in obese humans using molecular imaging methods. In a study among 52 healthy subjects (BMI mean ± SD: 24.8 ± 3.7 kg/m2) using [18F]altanserin PET, Adams et al. (2004) found a positive correlation between BMI and (postsynaptic) 5-HT2A receptor binding in all cortical regions except the occipital cortex. They replicated this finding in a larger sample (n = 136) of healthy subjects with a broader BMI range (18.2–42.8 kg/m2), including 14 obese subjects (Erritzoe et al. 2009). This time the correlation was found for the complete neocortex, including the occipital cortex. The authors interpreted this finding as a compensatory upregulation of the cortical 5-HT2A receptor availability due to lower brain serotonin levels in the overweight and obese subjects (Lam et al. 2010; Bjorntorp 1995).
In a sample of 28 healthy subjects (BMI range: 20.5–40.0 kg/m2) using [11C]SB207145 PET, a positive correlation between BMI and (postsynaptic) 5-HT4 receptor binding in the nucleus accumbens, globus pallidus, orbitofrontal cortex, and left temporal pole was found (Haahr et al. 2012). It is of particular interest that the positive correlations were mainly found in brain regions involved in the brain reward and salience circuitry, which plays an important role in overeating behavior.
There are two studies investigating the association between BMI and the serotonin transporter (SERT), which regulates synaptic serotonin levels. The largest study was performed by Erritzoe et al. (2010), who showed in a [11C]DASB PET study with 60 healthy volunteers ranging in BMI from 20.6 to 32.4 kg/m2 (including 7 obese subjects) that BMI was negatively correlated with SERT binding in the global neocortex and in some subcortical regions (caudate nucleus, putamen, thalamus, and midbrain). This finding contrasts with results reported by Koskela et al. (2008). In this previously mentioned study among monozygotic twins, both DAT and SERT levels were investigated. Although SERT binding was not correlated with BMI on an individual level, heavier twins overall had higher SERT binding in the hypothalamus/thalamus region than their leaner twin siblings. The significance of this finding appeared to be based on differences within the female twin pairs, while there was no significant difference in SERT binding within the male twin pairs between the heavier and the leaner twin. Another study showed that obese women with binge eating disorder have decreased SERT availability in the midbrain compared to obese non-binge eating women (Kuikka et al. 2001). In a subsample of these women, successful treatment of the binge eating disorder went together with an increase of SERT availability in the midbrain (Tammela et al. 2003). This suggests that obese women with binge eating disorder have aberrant midbrain SERT availability which could normalize after treatment. Together, the reviewed studies on SERT binding indicate that a dysregulation of subcortical and cortical SERT levels is associated with a high BMI, but more studies are needed to elucidate the exact direction of the associations and to discover how it is regulated in obesity.
27.3 Discussion
Several independent research groups have shown that the dopaminergic and serotonergic systems are involved in obesity, highlighting the importance of dysregulated molecular processes in the brain in obesity. The main findings that were replicated at least once are that the striatal DRD2/3 availability is lower in obese subjects compared to normal-weight controls, that striatal DAT availability is not different, and that 5-HT2A receptor binding in cortical regions correlates positively with BMI.
27.3.1 Discussion of Findings on the Dopaminergic System
The lower striatal DRD2/3 availability in obesity is supported by animal research: Decreased DRD2/3 levels were found in the nucleus accumbens (NAc) and dorsal striatum in genetically obese rodent models and in diet-induced obese rodent models (van de Giessen et al. 2012b; 2013; Hamdi et al. 1992; Hajnal et al. 2008; Davis et al. 2009; Thanos et al. 2008; Johnson and Kenny 2010), although one study reported an increase in DRD2/3 levels in the dorsal striatum of diet-induced obese mice (South and Huang 2008). The lower striatal DRD2/3 availability has been linked to reward deficiency. It has been postulated that a decrease in DRD2/3 availability results in a decreased sensitivity of the reward circuit to food, which subsequently leads to increased food intake to temporarily reach the desired reward level (Volkow et al. 2011). The role of this system has been compared to its role in substance use disorders. In this respect, it is important to notice that the studies with morbidly obese subjects found striatal DRD2/3 binding reduction of a magnitude (17.4 % (Wang et al. 2001) and 13.4 % (Volkow et al. 2008)) very similar to those observed in alcohol (Volkow et al. 1996a), methamphetamine (Volkow et al. 2001a), and opiate abusers (Wang et al. 1997). However, in obese subjects, lower DRD2/3 availability has not yet been linked to eating behavior or other behavioral parameters. Apart from that, there is increasing evidence that glucose homeostasis can directly affect the striatal dopaminergic system (Morris et al. 2011). The obese state might therefore affect striatal DRD2/3 availability via imbalances in metabolic systems, and a focus on eating behavior alone would be too narrow.
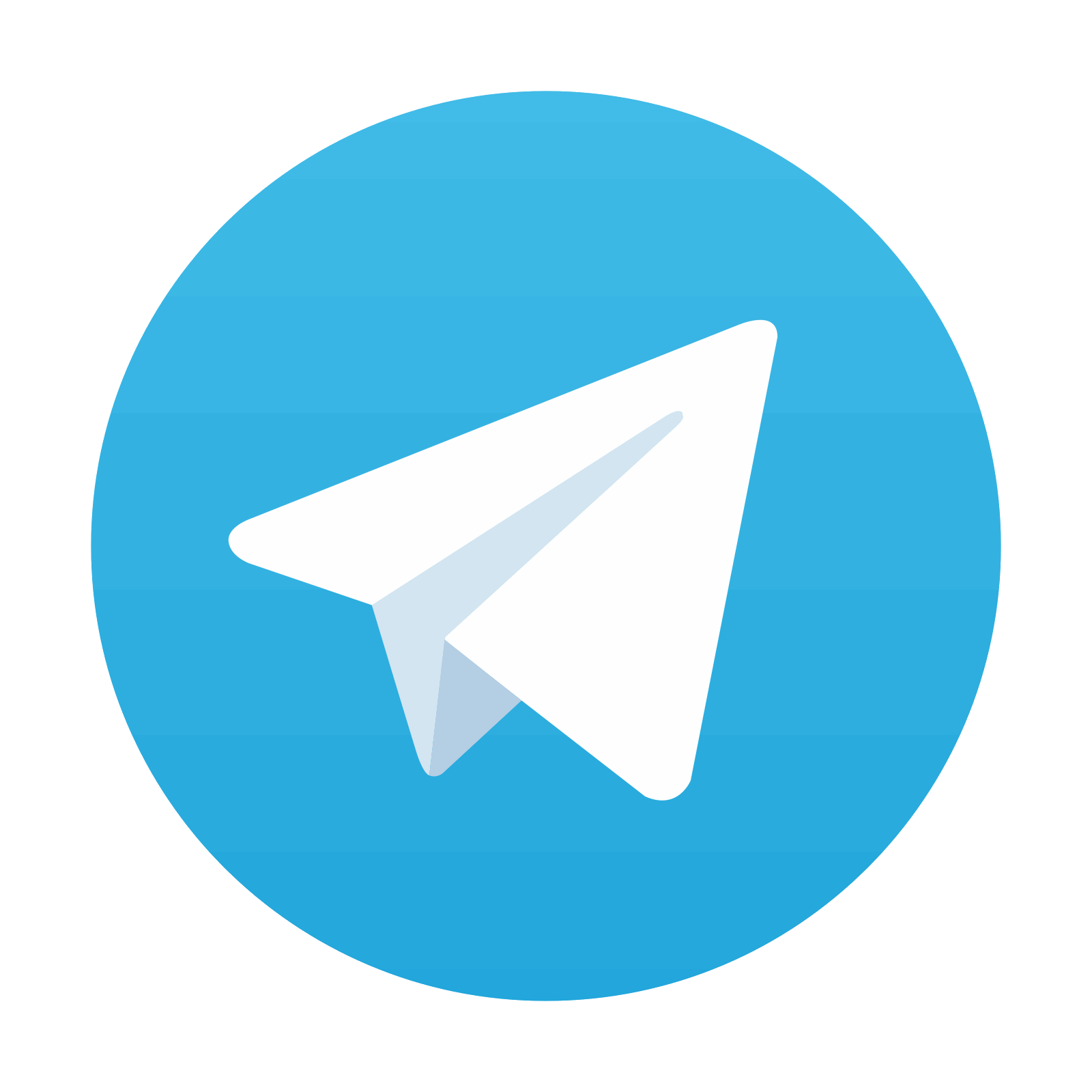
Stay updated, free articles. Join our Telegram channel

Full access? Get Clinical Tree
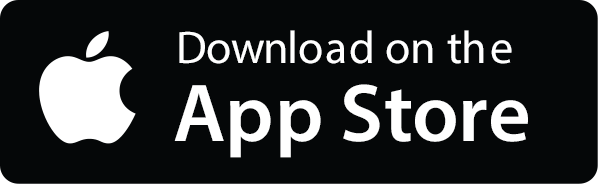
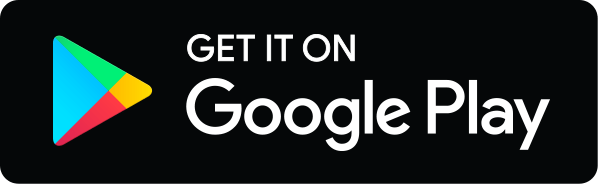