AAP guidelines
ERS guidelines
In a child who snores and has signs or symptoms of OSA, clinicians should perform a more focused evaluation
Step 1,2,3 – identification of a child at risk of OSA, morbidity and conditions co-existing with OSA, recognition of factors predicting persistence of OSA
PSG is the gold standard diagnostic test for OSA
Step 4 – objective diagnosis and assessment of disease severity PSG or RP study
If PSG is not available, alternative diagnostic tests (such as nocturnal video recording, nocturnal oximetry, daytime nap PSG, or ambulatory PSG) or referral to a specialist for more extensive evaluation should be considered
If not available, alternatives include ambulatory PSG/RP, nocturnal oximetry, sleep clinical record, or pediatric sleep questionnaire
Adenotonsillectomy is the first-line treatment in children with OSA who have associated adenotonsillar hypertrophy. Other treatments can be considered if the child does not have adenotonsillar hypertrophy
Postoperatively, high-risk patients should be monitored as inpatients
CPAP is recommended if adenotonsillectomy is not performed or if there is residual OSA
Intranasal corticosteroids should be considered in children with mild OSA in whom AT is contraindicated or for mild residual OSA post-AT
Weight loss should be recommended in patients who are overweight/obese
Step 5 – indications for treatment:
If AHI > 5/hrTST
If 1 > AHI > 5/hrTST, treatment may be warranted if any OSA-associated morbidities present
Treatment should be a priority in conditions such as craniofacial syndromes, trisomy 21, neuromuscular conditions, Prader-Willi syndrome, mucopolysaccharidoses, achondroplasia, Chiari malformation
Step 6 – treatment interventions should be implemented in a stepwise manner addressing all abnormalities that predispose to OSA:
Weight loss in children who are overweight/obese
Medical therapy (nasal steroids/montelukast)
Adenotonsillectomy
Rapid maxillary expansion/orthodontic appliances
CPAP/bilevel positive pressure ventilation
Tracheostomy
Patients should be reevaluated postoperatively to determine whether there is residual OSA and if further treatment is required
Objective testing should be performed in patients who are high risk or have symptoms/signs of residual disease after therapy
Step 7 – recognition and management of residual OSA
Reevaluation following intervention:
If OSA symptoms persist or at risk of residual OSA, PSG ≥ 6 weeks after AT or ≥12 weeks after medical therapy
PSG 12 months after rapid maxillary expansion, 6 months with an oral appliance
PSG for initial titration of CPAP/NIV, then at least yearly
Monitoring with PSG to guide tracheostomy decannulation
When residual OSA is demonstrated, airway evaluation by drug-induced sleep endoscopy, nasopharyngoscopy, or MRI may help identify site/s of obstruction
Diagnosis
As already alluded to earlier in this chapter, clinical history and examination unfortunately have poor sensitivity and specificity for the diagnosis of OSA. Objective testing is therefore recommended for children with symptoms of OSA, and the American Academy of Pediatrics suggests that the gold standard investigation is a nocturnal, in-lab polysomnography (PSG) study [195]. A typical montage would include EEG, chin and anterior tibial EMG, bilateral electrooculogram, pulse oximetry and pulse waveform, nasal pressure transducer, oronasal airflow thermistor, end-tidal capnography, chest and abdominal respiratory inductance plethysmography, body position sensor, microphone, and videomonitoring. Pediatric scoring criteria should be used, and most sleep laboratories score according to the American Academy of Sleep Medicine (AASM) guidelines [196]. PSGs provide objective quantitative data regarding respiratory parameters and sleep patterns, thus allowing patients to be stratified into disease severities, while also enabling clinicians to tailor clinical management accordingly.
In countries where PSGs are not readily available, alternative options include:
- (a)
- (b)
Nocturnal Oximetry Studies – Oximetry studies have a high specificity but low sensitivity for the diagnosis of pediatric OSA. A recent systematic review revealed that in otherwise healthy children with no symptoms of OSA, nocturnal SpO2 drops <90 %, greater than two clusters of desaturations events (≥4 %), and oxyhemoglobin desaturation index (≥4 %) >2.2/h are rare [199]. At least three clusters of desaturations and at least three SpO2 drops below 90 % in a nocturnal oximetry recording of at least 6-h duration (McGill oximetry score >1) are suggestive of moderate-to-severe OSA (Fig. 10.1). An oxyhemoglobin desaturation index (≥4 %) >2/h combined with OSA symptoms also exhibits high positive predictive value for AHI >1/h. Children with normal oximetry, aged >3 years, and with no comorbidities have very low risk of major respiratory complications following adenotonsillectomy. Overnight oximetry can help in the prioritization of treatment and determining whether children need to be admitted overnight for observation following adenotonsillectomy. It has an important role in resource-poor countries where PSGs or RPs are not available. Improvements in oximetry-based approaches using neural networks suggest that this methodological approach can improve the accuracy of the diagnosis [200].
Fig. 10.1
(a) Example of abnormal overnight oximetry study in child with OSA. (b) 2-min epoch from a respiratory polygraphy study of the same patient performed the same night
- (c)
Sleep Clinical Record – The sleep clinical record (SCR) is an instrument that has been developed to screen children for OSA and incorporates multidimensional data obtained from case history and physical examination [201]. A score of ≥ 6.5 is considered positive for OSA, with a reported sensitivity of 96.05 % and specificity of 67 %. Combining results from the SCR score and nocturnal oximetry has moderate success in predicting sleep-disordered breathing severity and is potentially useful in settings where resources are limited and PSG testing is not readily available [202].
In general and pragmatically speaking, while these investigations may not have the sensitivity and specificity of PSGs, provided clinicians recognize their limitations, the information they provide can still be highly valuable.
Home sleep apnea testing
There has been a lot of interest in home sleep apnea testing, and it is a direction that adult sleep medicine has readily adopted. Compared with in-lab PSGs, home sleep apnea testing has the potential to measure a more typical night’s sleep as the child is sleeping at home, could be substantially less expensive, and more accessible to more children. Most of the interest in home sleep apnea testing has been in the use of home RPs. Unsurprisingly, the signal most at risk of disruption is the nasal flow signal, but overall, home RPs are feasible to perform, even in young preschool children [203, 204]. The probability of obtaining a successful study appears to be higher if the studies are set up by trained staff with the attendant logistic implications. Though results from the various published studies have been discordant [197], on the whole, the sensitivity and specificity of home RPs appear to be good for the diagnosis of moderate and severe OSA in children who have a high pretest probability of having the condition [205]. More research is required into how to further optimize the sensitivity and specificity of home sleep apnea testing in children with mild OSA.
CO2 monitoring is important in children in whom there is concern regarding nocturnal hypoventilation, such as children with neuromuscular disease, underlying lung disease, or obesity hypoventilation. Not all home testing devices have a transcutaneous or end-tidal CO2 channel, and therefore, home sleep apnea testing may not be suitable in these children.
Treatment
Most clinicians consider a PSG obstructive AHI ≤1/hrTST to be normal, 1 < OAHI ≤5/hrTST as mild OSA, 5 < OAHI ≤10/hrTST as moderate OSA, and OAHI >10/hrTST as severe OSA. Most sleep specialists would institute treatment in children with moderate/severe OSA, but there is considerable variability in current practice for the management of mild OSA. It is important to remember that treatment decisions should not only be guided by PSG results in isolation, and the overall clinical picture needs to be taken into consideration. Thus, symptom severity, examination findings, presence of risk factors, and associated disease morbidity need to be carefully evaluated and incorporated prior to the formulation and implementation of an individualized treatment plan.
Adenotonsillectomy
In contrast to adults with OSA, adenotonsillectomy is the recommended first-line treatment for pediatric OSA in children with adenotonsillar hypertrophy [195]. It is now clearly established that adenotonsillectomy results in improvements in the severity of OSA in the majority of children. While adenotonsillectomy is an effective treatment, recent studies have demonstrated that although the majority of children show marked improvements in their PSG parameters following surgery, a significant proportion do not achieve complete normalization of the PSG and have residual OSA [206].
Risk factors for significant residual postoperative OSA include obesity, severity of OSA presurgery, age (risk being higher in those aged >7 years), underlying asthma or allergic rhinitis, African-American ethnicity, and children who have primary genetic conditions leading to structural abnormalities, e.g., craniofacial anomalies/chromosomal defects, and those with neuromuscular diseases. In low-risk populations, the percentage of children with residual OSA is approximately 20–25 %, while in high-risk populations, it is significantly higher [43, 46]. Clinicians should be alert to this problem, and those children at high risk for residual OSA should be reevaluated post-adenotonsillectomy. However, even in low-risk patients, an open-minded approach should be implemented, with the recurrence of OSA symptoms warranting reevaluation.
While adenotonsillectomy is generally considered a safe procedure, as with all surgical procedures, there can be complications, the most common being respiratory compromise and secondary hemorrhage [207]. It is now apparent that children with OSA have an increased risk of respiratory complications after adenotonsillectomy when compared to children who are having adenotonsillectomy for other clinical indications (odds ratio 4.9). However, they appear less likely to have postoperative bleeding compared with children without OSA (odds ratio 0.41). A possible explanation is that recurrent tonsillitis, the other main indication for adenotonsillectomy, may be a risk factor for secondary hemorrhage, not that OSA is a protective factor. Of note, in the CHAT study, none of the PSG or demographic parameters were predictive of postoperative complications [208].
There has been a gradual shift in the past few years by some ENT surgeons toward performing tonsillotomies in preference to tonsillectomies because of the benefits of lower postoperative complication rates such as hemorrhage, less postsurgical pain, and shorter recovery time. A recent meta-analysis comparing tonsillectomy versus tonsillotomy for sleep-disordered breathing in children included ten studies and demonstrated that there was no significant difference in outcomes such as resolution of OSA symptoms, quality of life, and postoperative immune function [209]. However, the risk ratio of OSA recurrence was 3.33 times higher for tonsillotomy especially in younger children who appear to have a small risk of symptom-recurrence requiring repeat surgery within 2 years after tonsillotomy due to tonsillar regrowth [210]. The 2012 American Academy of Pediatrics guidelines suggest that data are currently insufficient to definitively recommend one surgical technique over the other [195]. However, children undergoing tonsillotomy should be monitored carefully long term to ensure that OSA symptoms do not recur, and families should be counseled about the possibility of OSA recurrence secondary to tonsillar regrowth. In a population-based study, the risk of recurrent surgical intervention was significantly higher when tonsillotomy was used [211].
A recent Cochrane systematic review to examine the evidence comparing adenotonsillectomy versus nonsurgical management of pediatric OSA identified only three prospective trials that met the inclusion criteria [212]. The previously mentioned CHAT study provided the highest-quality evidence and was the main basis for the recommendation that in healthy children with mild/moderate OSA, adenotonsillectomy was of benefit. It is still the duty of the physicians to carefully weigh the benefits and risks of adenotonsillectomy against watchful waiting, as it is possible the condition may recover spontaneously over time. Certainly one of the most striking findings of the CHAT study was that in the watchful waiting group, 42 % were found to have resolution of their OSA on follow-up PSG 7 months later [48]. This was particularly true in the children with milder disease (lower initial AHI) and with waist circumference <90 % percentile [213]. It should be pointed out that despite normalization of their PSG AHI, only 15 % of the 167 patients with a baseline PSQ > 0.33 experienced symptomatic resolution, and the independent predictors for symptom resolution included lower initial pediatric sleep questionnaire and snoring scores.
Positive Airway Pressure Therapy
In children who manifest residual OSA after adenotonsillectomy or in those who present minimally enlarged upper airway lymphadenoid tissues or opt not to undergo surgery, positive airway pressure therapy is recommended (provided nasal obstruction is not present). The aim is to maintain airway patency throughout the respiratory cycle, improve functional residual capacity (FRC), and thus decrease the work of breathing. This is usually delivered in the form of continuous positive airway pressure (CPAP), but in some children who require very high positive end-expiratory pressures or who also have nocturnal hypoventilation, for example, children with neuromuscular disease or obesity hypoventilation syndrome, bilevel PAP ventilation may be required (Fig. 10.2). While PAP is an effective therapy, achieving adequate adherence can be a major challenge in children.


Fig. 10.2
(a) Children can take a little while to get used to PAP therapy, but once they get into a routine, they can feel symptomatically much better when they adhere to this therapy. (b) Example of adherence data from a child with OSA receiving CPAP therapy at home
When adherence and effectiveness were studied in a prospective multicenter study of children randomly assigned to 6 months of bilevel PAP ventilation or CPAP, a third of the subjects had already dropped out before 6 months [214]. There was no statistical difference between bilevel PAP ventilation and CPAP, both being highly efficacious, even though adherence, even in a research setting, was suboptimal with a mean nightly use of just 5.3 ± 2.5 (SD) hours. Nonetheless, achieving good adherence is not impossible, as evidenced by Ramirez et al. whose patients used PAP therapy for a mean of 8 h 17 min ± 2 h 30 min per night [215]. This impressive outcome is most likely a reflection of the available resources, with the PAP therapy being initiated in a designated pediatric inpatient unit by experienced staff, very frequent home visits, and frequent inpatient follow-up sleep studies. However, even with suboptimal adherence (mean use just 170 ± 145 min/night), a study of 52 children found that there was still significant improvement after just 3 months of PAP therapy in several neurobehavioral domains, including attention deficits, sleepiness on the Epworth sleepiness scale, internalizing and total behavior symptom scores, and quality-of-life questionnaires [119].
PAP therapy does however require regular long-term follow-up in children as pressure requirements will change, and the interface will need to be upsized and adjusted, with the growth and development of the child. Side effects also need to be monitored, and these can include nasal bridge pressure sores from the mask, discomfort from air leak especially to the eyes, abdominal distension, and oronasal dryness. In the longer term, young children may develop flattening of the midface or maxillary retrusion from the effect of pressure of the mask on growing facial structures.
Medical Therapy
Most clinicians would now consider a trial of anti-inflammatory medication, namely, leukotriene receptor antagonists such as montelukast and intranasal steroids in children with mild OSA [216]. Tonsils from children with OSA have been shown to express increased levels of leukotriene receptors 1 and 2 compared with tonsils from children with recurrent tonsillitis [217]. Consequently, the application of leukotriene antagonists to an in vitro cell culture system of cells obtained from tonsillar tissues of children with OSA elicited dose-dependent reductions in cell proliferation and reductions in the secretion of the cytokines TNF-α, IL-6, and IL-12 [218]. In an initial open-label intervention study where children with mild OSA received 16 weeks of montelukast, significant reductions in adenoidal size and respiratory-related sleep disturbances were demonstrated [219]; these findings have subsequently been reproduced in a double-blind, randomized, placebo-controlled trial [220].
Similarly, the addition of steroids to this in vitro cell culture system resulted in decreased proliferative rates of the cells, increased apoptosis, and reduction in the secretion of the pro-inflammatory cytokines IL-6, IL-8, and TNF-α [221]. A randomized crossover trial of 6 weeks of treatment with intranasal budesonide in children with mild OSA showed improvements in the severity of OSA and reduction in the size of adenoidal tissues. Importantly, discontinuation of therapy for 8 weeks, in the group of children who started with the medication and then had a 2-week washout period followed by 6 weeks of placebo, did not promote the occurrence of rebound symptoms [222]. Intranasal fluticasone and mometasone have shown similar results [223, 224]. The use of both montelukast and nasal budesonide for 12 weeks in children who had residual mild residual OSA after adenotonsillectomy led to significant improvements in AHI, nadir oxygen saturation, and respiratory arousal index, whereas no significant changes occurred over this time period in the control subjects [225]. Recently, a large retrospective review of 752 children with mild OSA who received nasal steroids and montelukast showed an overall success rate of 80.5 % [226]. A subset of 445 patients had follow-up PSG and normalization of sleep parameters was seen in 62 %. Older children (aged > 7 years) and obese children were however less likely to respond favorably. Studies to determine optimal duration of treatment, optimal combinatorial approaches, longer-term outcomes, and optimal patient selection are critically needed.
Weight Loss
Weight loss should be encouraged in obese children with OSA, both as a therapy for their OSA and also for its beneficial effects on their overall health. In the NANOS study, the group of obese children with mild OSA without adenotonsillar hypertrophy who were managed with dietary modification to encourage weight loss demonstrated improvements in their respiratory parameters with 50 % showing OSA resolution at follow-up [50], while the weight loss required for such beneficial effects to be demonstrated was encouragingly small. A study in Belgium recruited 61 obese teenagers admitted to a weight loss camp where the children underwent a multimodality treatment program consisting of moderate dietary restriction, regular physical activity, as well as group and individual psychological support [227]. Sixty-two percent had OSA when they started the program, and after a median weight loss of 24 kg, repeat sleep screening revealed a significant improvement in the severity of OSA including the AHI, ODI, mean SpO2, SpO2 nadir, and time SpO2 < 90 %, while the relative decrease in BMI z-score correlated with the change in AHI.
Rapid Maxillary Expansion
In rapid maxillary expansion (RME), a fixed appliance with an expansion screw anchored to opposing teeth is used to open the midpalatal suture, gradually increasing the transverse diameter of the hard palate over the course of several months. Data on RME are limited to small uncontrolled studies, but they suggest that it may have a role to play in carefully selected patients, such as children with OSA who have clinical signs of malocclusion (high, narrow palate associated with deep bite, retrusive bite, or crossbite) [228]. Results appear to be more promising when treatment is started at a younger age during the phase of late primary dentition or early mixed dentition [229]. In a pilot study of 31 children with OSA who had both narrow maxilla and moderately enlarged tonsils and OSA, half the children (group 1) had adenotonsillectomy performed followed by RME, and the other half (group 2) had RME, followed by adenotonsillectomy [230]. The AHI decreased after the first intervention, but it was only after the reciprocal intervention was performed that the AHI normalized. Complete resolution of OSA following just a single intervention alone (RME) occurred in only one child, and there were no significant differences as to which treatment was started first, as ultimately both treatments were needed in the vast majority of patients.
Myofunctional Reeducation
There has been recent interest in myofunctional reeducation to prevent the recurrence of OSA post-adenotonsillectomy or in the treatment of mild persistent OSA. Myofunctional reeducation involves teaching the patients oropharyngeal exercises aimed at improving labial seal and lip tone, facilitating nasal breathing rehabilitation, and improving tongue posture. These exercises need to be performed daily, with the aim of strengthening the tongue and orofacial muscles and realigning them in the correct position. In a retrospective review of 24 patients after adenotonsillectomy, 11 of whom had received myofunctional reeducation, those children who had not received myofunctional therapy developed recurrence of mild OSA symptomatically and on PSG, whereas those undergoing myofunctional reeducation had no recurrence [231]. Another study of 14 children who had residual OSA post-adenotonsillectomy demonstrated improvement in their AHI after treatment with oropharyngeal exercises for 2 months [232]. Morphofunctional evaluation also demonstrated improvements including reduction in oral breathing and increased labial seal and lip tone. One hypothesis is that residual OSA may be due to incomplete recovery of neuromuscular function after surgery, and myofunctional reeducation may help with this problem. However, studies so far have been small and uncontrolled, and therefore, larger prospective, multicenter controlled studies are required.
High-Flow Nasal Cannula Oxygen
A possible alternative to CPAP is high-flow nasal cannula oxygen (HFNC). This form of respiratory support has gained widespread use in the acute setting for the treatment of respiratory distress syndrome and bronchiolitis, and anecdotally appears to be better tolerated in infants than mask-based positive airway pressure. There has only been 1 study so far of 12 patients on HFNC for the treatment of OSA [233]. Results were promising, and the reduction in AHI was comparable to that of CPAP leading the authors to postulate that HFNC may be a gentler alternative to CPAP.
Newer Investigational Techniques
Drug-induced sleep endoscopy
Drug-induced sleep endoscopy (DISE) is a technique whereby the upper airway is evaluated via a flexible fiber-optic endoscope inserted nasally during spontaneous breathing while the patient is under light sedation. The aim is to recreate the upper airway conditions during natural sleep, thus allowing the exact level of obstruction in the child to be identified and facilitating site-specific surgical therapy [234, 235]. It can be very useful in the assessment of children who have residual OSA after adenotonsillectomy, or in complex OSA cases, such as that seen in children with cerebral palsy, craniofacial syndromes, trisomy 21, etc. Common sites of obstruction include the tongue base, primarily due to lingual tonsillar hypertrophy, adenoid regrowth, inferior turbinate hypertrophy, and laryngomalacia.
Cine MRI
Another technology potentially useful for the evaluation of children with complex upper airway obstruction is cine MRI. This approach provides high-resolution imaging of the dynamic airway without the need for ionizing radiation making it ideal for use in children. Cine MRI proponents advocate that this imaging modality allows for a better view of the airway in its entirety and enables observation of both the primary and secondary effects of obstruction. When Shott et al. used cine MRI to evaluate the airways of 15 children with trisomy 21 who had persistent OSA following adenotonsillectomy, the cine MRI identified different areas and levels of obstruction including regrowth of adenoidal tissue, glossoptosis, soft palate collapse, hypopharyngeal collapse, and enlarged lingual tonsils, and treatment could thus be tailored to the individual patient [236, 237].
Guidelines
The most recent American and European guidelines for the diagnosis and management of pediatric OSA were published in 2012 and 2015, respectively. The American Academy of Pediatrics (AAP) focused on uncomplicated OSA, i.e., OSA associated with adenotonsillar hypertrophy or obesity in otherwise healthy children [6]. Upon reviewing the data from publications between 1999 and 2010, their main recommendations are summarized in Table 10.2.
Table 10.2
Pertinent symptoms and signs when evaluating a child for possible OSA
Clinical history |
Are there any of the following symptoms? |
During sleep |
Snoring (is it loud? How frequently) |
Heavy/loud/noisy breathing |
Struggling to breath |
Gasping |
Mouth breathing |
Witnessed apneas |
Restless sleep |
Frequent awakenings |
Hyperextension of neck when asleep |
Sleeps in sitting position |
Excessive sweating when asleep |
Cyanosis |
What is the typical bedtime, wake time? |
Is the child easy to wake in the mornings? |
Does the child wake unrefreshed? |
Nocturnal enuresis (especially if secondary) |
Wake with morning headaches |
Any other sleep symptoms, e.g., parasomnias or night terrors? |
During wake |
Nasal congestion |
Mouth breathing when awake |
Chronic rhinorrhea |
Difficulty swallowing |
Neurocognitive morbidity, e.g., academic impairment, excessive daytime sleepiness (consider questionnaire such as modified Epworth sleepiness scale), inattention, hyperactivity, behavioral problems |
Are there any risk factors for OSA such as prematurity or family history of OSA? |
Are there any other medical conditions, e.g., oromotor dysfunction, asthma, metabolic syndrome, recurrent otitis media, allergic rhinitis, recurrent chest infections, depression/ low self esteem, sickle cell disease, or previous cleft palate repair? |
Is the child on any medications which can impact on sleep? |
Physical examination |
Tonsillar hypertrophy |
Mandibular hypoplasia/micrognathia/retrognathia/midface deficiency/high-arched palate |
Macroglossia |
Pectus excavatum |
Adenoidal facies |
Examination of nose – nasal patency, any obvious causes of obstruction |
Plot on growth chart |
Calculate BMI percentile or z-score |
Is there any evidence of other conditions commonly associated with OSA? |
Neuromuscular conditions |
Cerebral palsy |
Achondroplasia |
Mucopolysaccharidoses |
Syndromes such as trisomy 21, Prader-Willi syndrome, craniofacial syndromes, etc. |
Cardiovascular morbidity measure blood pressure, is there any evidence of pulmonary hypertension |
Objective findings related to OSA |
Parents now frequently take videos on their phone of events they are worried about which can provide useful information |
Other investigations that can be considered or may already have been performed: |
Lateral neck radiograph (adenoid: nasopharyngeal ratio) |
Flexible nasoendoscopy |
Cephalometry |
Upper airway MRI or CT |
In addition to the management of uncomplicated OSA, the European Respiratory Society guidelines also included recommendations regarding the management of obstructive sleep-disordered breathing in children with conditions such as craniofacial anomalies and neuromuscular disorders [1]. The variability in diagnostic facilities available in different European countries was also taken into consideration. Reviewing publications until 2014, a stepwise approach to the diagnosis and management of obstructive sleep-disordered breathing was suggested, and these are also summarized in Table 10.2.
Future Developments
PSGs are poorly predictive of OSA-associated morbidities: not every child fulfilling PSG criteria for OSA manifests end-organ morbidity, and conversely, some children with primary snoring already display sequelae despite a normal sleep study. As with many diseases, factors such as individual genetic susceptibility and environmental exposures/lifestyle will contribute to this phenotypic variance. Therefore, improving overall therapy requires individualization of evaluation and treatment and is dependent on patient-specific requirements. The development of potential biomarkers of susceptibility to and severity of OSA morbidity is therefore of critical importance. Proteomic interrogations coupled with the requisite bioinformatic analyses have revealed that OSA is associated with specific and consistent alterations in certain urinary proteins [238]. Increased levels of urinary catecholamines epinephrine and norepinephrine have been identified, and overnight increases in GABA and decreases in taurine and β-phenylethylamine (PEA) appear to differentiate children with OSA who have neurocognitive deficits from those without [239]. There is also emergent data that exosomal miRNAs may be a potential biomarker of cardiovascular risk in children with OSA [240].
The era of personalized medicine will require the development of coordinated combinatorial or multiplexed approaches, so that diagnosis and treatment can be tailored to the individual patient. In the future, algorithms that incorporate measures derived from the sleep study, from blood or urine tests, and from clinical elements obtained during history and physical examination may provide improved approaches to define those at risk of OSA, together with the timing and the nature of their required intervention. This unified approach is endorsed by an official American Thoracic Society statement on the importance of healthy sleep released in 2015 [241]. The research priorities are highlighted to include determining the molecular basis for OSA, using knowledge of these pathways to develop effective therapies, identifying the etiological role of OSA in the development of comorbidities, and determining the impact of OSA treatment on these comorbidities.
Financial Disclosures
DG is supported by the Herbert T. Abelson Chair in Pediatrics.
Conflicts of Interest Statement
The authors declare no conflicts of interest in relation to this work.
References
1.
Kaditis AG, Alonso Alvarez ML, Boudewyns A, et al. Obstructive sleep disordered breathing in 2- to 18-year-old children: diagnosis and management. Eur Respir J. 2016;47:69–94.PubMed
2.
Guilleminault C, Eldridge FL, Simmons FB, Dement WC. Sleep apnea in eight children. Pediatrics. 1976;58:23–30.PubMed
3.
Kheirandish-Gozal L, Gozal D. Sleep disordered breathing in children. 1st ed. Berlin: Springer Science; 2012.
4.
Bixler EO, Vgontzas AN, Lin HM, et al. Sleep disordered breathing in children in a general population sample: prevalence and risk factors. Sleep. 2009;32:731–6.PubMedPubMedCentral
5.
Li AM, So HK, Au CT, et al. Epidemiology of obstructive sleep apnoea syndrome in Chinese children: a two-phase community study. Thorax. 2010;65:991–7.PubMed
6.
Marcus CL, Brooks LJ, Draper KA, et al. Diagnosis and management of childhood obstructive sleep apnea syndrome. Pediatrics. 2012;130:e714–55.PubMed
7.
Rosen CL, Larkin EK, Kirchner HL, et al. Prevalence and risk factors for sleep-disordered breathing in 8- to 11-year-old children: association with race and prematurity. J Pediatr. 2003;142:383–9.PubMed
8.
Tauman R, Gozal D. Obstructive sleep apnea syndrome in children. Expert Rev Respir Med. 2011;5:425–40.PubMed
9.
Arens R, McDonough JM, Costarino AT, et al. Magnetic resonance imaging of the upper airway structure of children with obstructive sleep apnea syndrome. Am J Respir Crit Care Med. 2001;164:698–703.PubMed
10.
Schwab RJ, Kim C, Bagchi S, et al. Understanding the anatomic basis for obstructive sleep apnea syndrome in adolescents. Am J Respir Crit Care Med. 2015;191:1295–309.PubMedPubMedCentral
11.
Slaats MA, Van HK, Van EA, et al. Upper airway imaging in pediatric obstructive sleep apnea syndrome. Sleep Med Rev. 2015;21:59–71.PubMed
12.
Luo H, Sin S, McDonough JM, Isasi CR, Arens R, Wootton DM. Computational fluid dynamics endpoints for assessment of adenotonsillectomy outcome in obese children with obstructive sleep apnea syndrome. J Biomech. 2014;47:2498–503.PubMedPubMedCentral
13.
Wootton DM, Luo H, Persak SC, et al. Computational fluid dynamics endpoints to characterize obstructive sleep apnea syndrome in children. J Appl Physiol (1985). 2014;116:104–12.
14.
Van HC, Vos W, Van HK, et al. Functional respiratory imaging as a tool to assess upper airway patency in children with obstructive sleep apnea. Sleep Med. 2013;14:433–9.
15.
Persak SC, Sin S, McDonough JM, Arens R, Wootton DM. Noninvasive estimation of pharyngeal airway resistance and compliance in children based on volume-gated dynamic MRI and computational fluid dynamics. J Appl Physiol (1985). 2011;111:1819–27.
16.
Mihaescu M, Murugappan S, Gutmark E, Donnelly LF, Kalra M. Computational modeling of upper airway before and after adenotonsillectomy for obstructive sleep apnea. Laryngoscope. 2008;118:360–2.PubMed
17.
Deacon NL, Jen R, Li Y, Malhotra A. Treatment of obstructive sleep apnea. Prospects for personalized combined modality therapy. Ann Am Thorac Soc. 2016;13:101–8.PubMed
18.
Owens RL, Edwards BA, Eckert DJ, et al. An integrative model of physiological traits can be used to predict obstructive sleep apnea and response to non positive airway pressure therapy. Sleep. 2015;38:961–70.PubMedPubMedCentral
19.
Parikh SR, Sadoughi B, Sin S, Willen S, Nandalike K, Arens R. Deep cervical lymph node hypertrophy: a new paradigm in the understanding of pediatric obstructive sleep apnea. Laryngoscope. 2013;123:2043–9.PubMedPubMedCentral
20.
Chan DK, Jan TA, Koltai PJ. Effect of obesity and medical comorbidities on outcomes after adjunct surgery for obstructive sleep apnea in cases of adenotonsillectomy failure. Arch Otolaryngol Head Neck Surg. 2012;138:891–6.PubMed
21.
Friedman NR, Prager JD, Ruiz AG, Kezirian EJ. A pediatric grading scale for lingual tonsil hypertrophy. Otolaryngol Head Neck Surg. 2016;154:171–4.PubMed
22.
Abdel-Aziz M, Ibrahim N, Ahmed A, El-Hamamsy M, Abdel-Khalik MI, El-Hoshy H. Lingual tonsils hypertrophy; a cause of obstructive sleep apnea in children after adenotonsillectomy: operative problems and management. Int J Pediatr Otorhinolaryngol. 2011;75:1127–31.PubMed
23.
Fricke BL, Donnelly LF, Shott SR, et al. Comparison of lingual tonsil size as depicted on MR imaging between children with obstructive sleep apnea despite previous tonsillectomy and adenoidectomy and normal controls. Pediatr Radiol. 2006;36:518–23.PubMed
24.
Kim J, Bhattacharjee R, Dayyat E, et al. Increased cellular proliferation and inflammatory cytokines in tonsils derived from children with obstructive sleep apnea. Pediatr Res. 2009;66:423–8.PubMedPubMedCentral
25.
Serpero LD, Kheirandish-Gozal L, Dayyat E, Goldman JL, Kim J, Gozal D. A mixed cell culture model for assessment of proliferation in tonsillar tissues from children with obstructive sleep apnea or recurrent tonsillitis. Laryngoscope. 2009;119:1005–10.PubMedPubMedCentral
26.
Goldbart AD, Mager E, Veling MC, et al. Neurotrophins and tonsillar hypertrophy in children with obstructive sleep apnea. Pediatr Res. 2007;62:489–94.PubMedPubMedCentral
27.
Snow A, Dayyat E, Montgomery-Downs HE, Kheirandish-Gozal L, Gozal D. Pediatric obstructive sleep apnea: a potential late consequence of respiratory syncytial virus bronchiolitis. Pediatr Pulmonol. 2009;44:1186–91.PubMed
28.
Yeshuroon-Koffler K, Shemer-Avni Y, Keren-Naus A, Goldbart AD. Detection of common respiratory viruses in tonsillar tissue of children with obstructive sleep apnea. Pediatr Pulmonol. 2015;50:187–95.PubMed
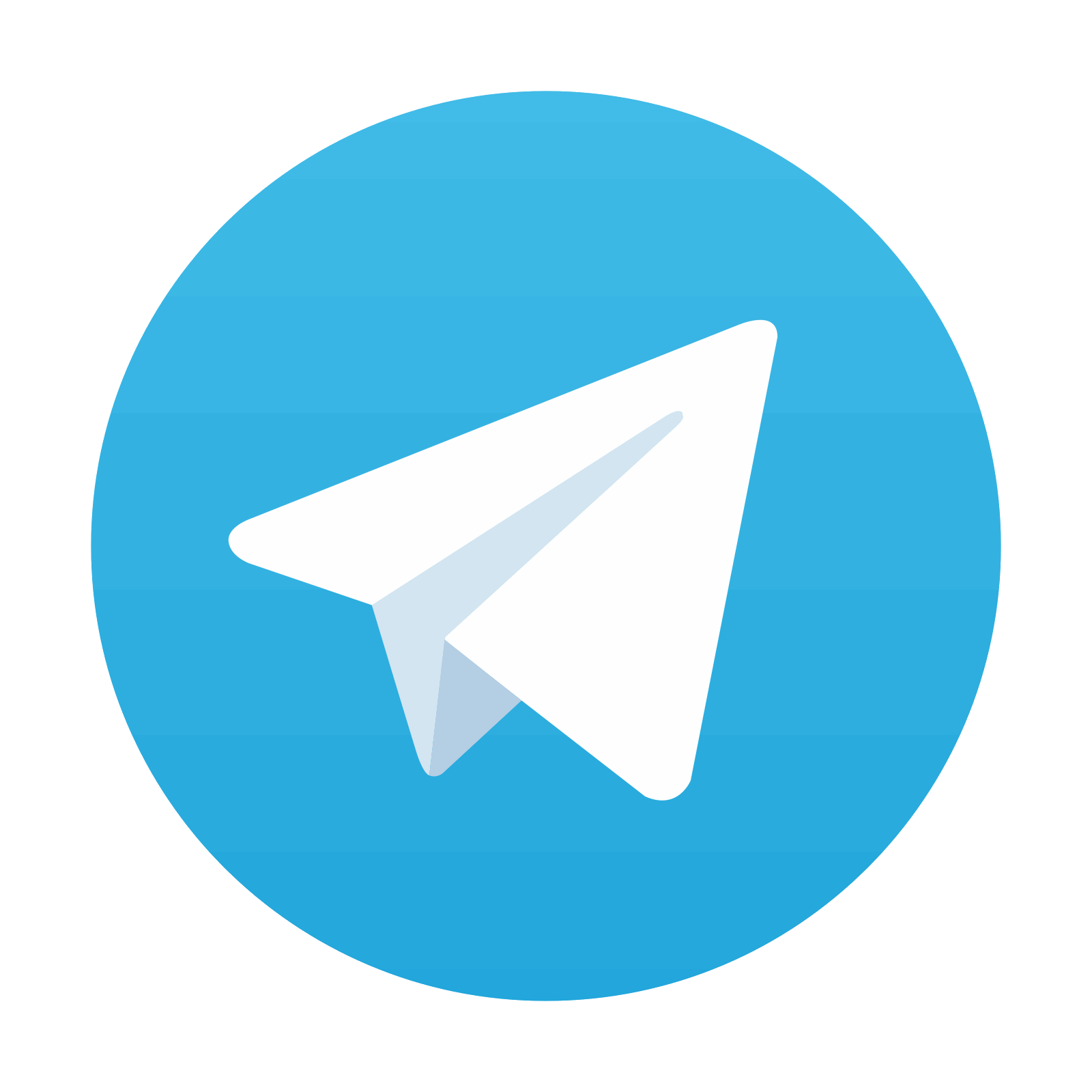
Stay updated, free articles. Join our Telegram channel

Full access? Get Clinical Tree
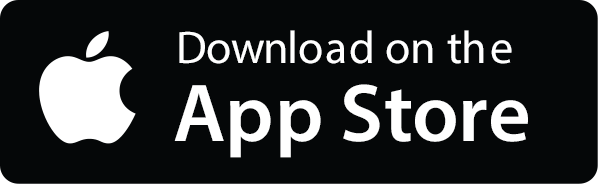
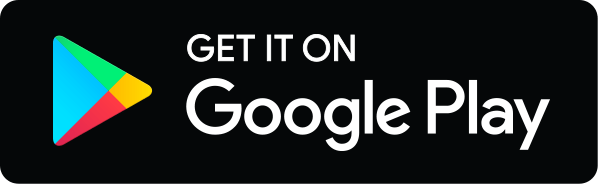