Fig. 1
T2-weighted MRI scans showing lateral ventricles (a) and periventricular white matter damage (b) at 24 h after thrombin (3 U) or saline (50 μl) injection into the right lateral ventricle in LCR and HCR rats. The bar graphs show quantification of the MRIs. Values are expressed as the means ± SD, n = 3 in LCR saline group, n = 8 in LCR/HCR thrombin group.
The number of HSP-32-positive cells in the periventricular white matter area was greater in LCR compared with HCR rats (Fig. 2a). This was confirmed by Western blot where HSP-32 protein levels in periventricular white matter were significantly higher in the LCR rats (HSP-32/β-actin ratio: 3.69 ± 0.42 vs 1.83 ± 0.63 in HCR rats, p < 0.01, Fig. 2b). Similarly, there were more activated microglial, OX-6-positive cells in the periventricular white matter area of LCR than in HCR rats (Fig. 3a). Western blot showed OX-6 protein levels in the periventricular white matter area were higher in LCR rats (OX-6/β-actin: 0.96 ± 0.09 vs 0.58 ± 0.12 in HCR rats, p < 0.05, Fig. 3b).
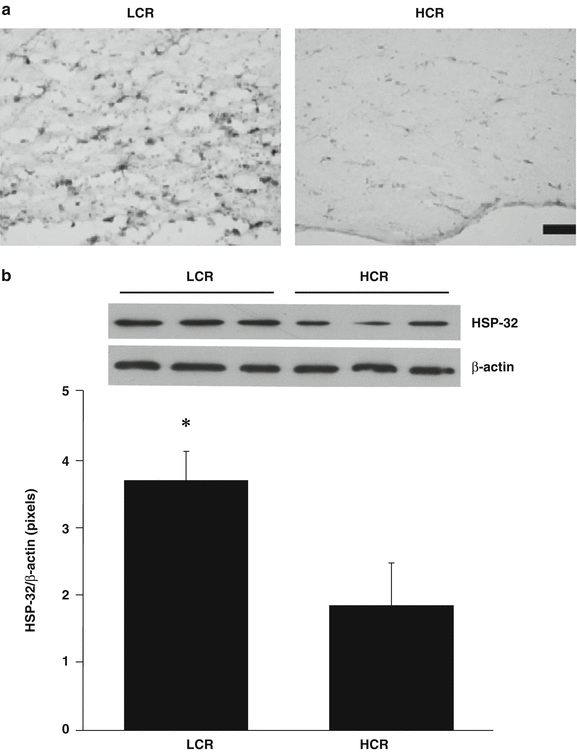
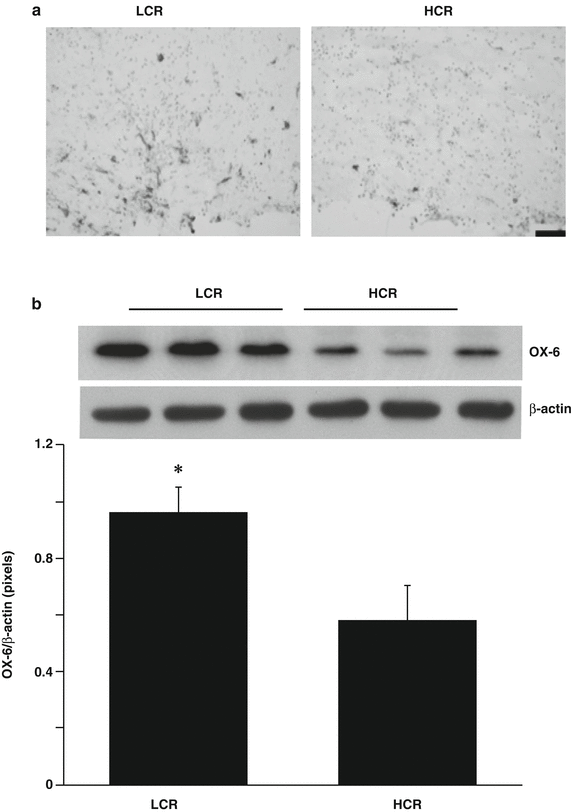
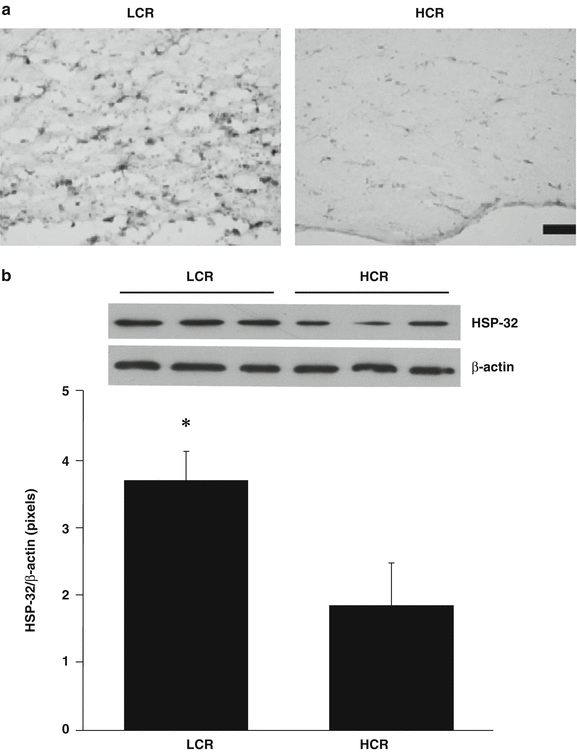
Fig. 2
(a) HSP-32 immunoreactivity in the periventricular white matter 24 h after intraventricular thrombin (3 U) injection in LCR and HCR rats. Scale bar = 50 μm. Representative example of n = 5. Note the increased expression of HSP-32 in the LCR rats. (b) HSP-32 protein levels in the periventricular white matter area at 24 h after intraventricular thrombin injection in LCR and HCR rats. Values are expressed as the means ± SD, n = 3, *p < 0.05
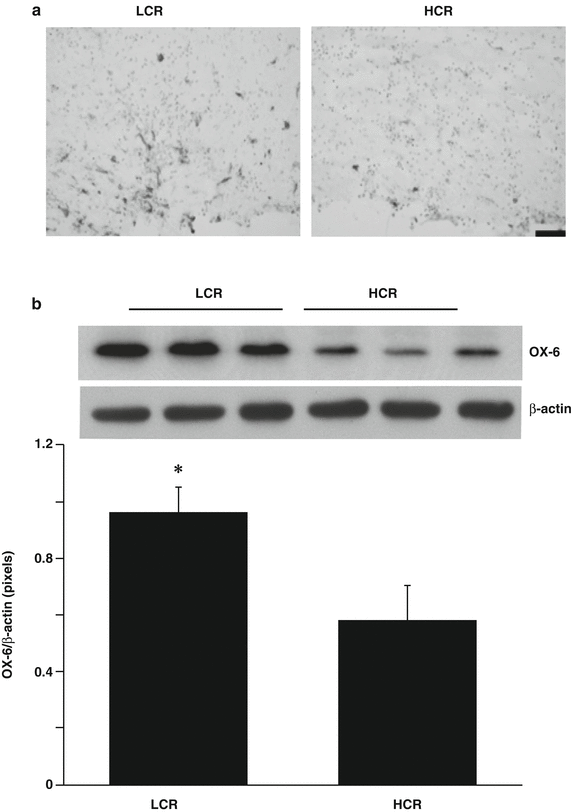
Fig. 3
(a) OX-46 immunoreactivity (marker of activated microglia) in the periventricular white matter 24 h after intraventricular thrombin (3 U) injection in LCR and HCR rats. Scale bar = 50 μm. Representative example of n = 5. Note the increased expression of HSP-32 in the LCR rats. (b) Ox-6 protein levels in the periventricular white matter area at 24 h after intraventricular thrombin injection in LCR and HCR rats. Values are expressed as the means ± SD, n = 3, *p < 0.05
Discussion
The present study demonstrates that thrombin injection causes more severe hydrocephalus and periventricular white matter damage in LCR rats compared with HCR rats. This was associated with greater HSP-32 expression and microglia activation. These results demonstrate a significant influence of exercise capacity on the initial impact of thrombin.
The LCR–HCR model system was developed based upon the aerobic hypothesis and provides polygenic-based experimental substrate for exploring both diagnostic and prescriptive translational clues that underlie disease risk factors. Low exercise capacity is a risk factor for stroke as well as other forms of cardiovascular disease [11, 13]. Thus, the LCR rats have diminished longevity [10], metabolic syndrome [21], hepatic steatosis [20], and a disordered capacity to oxidize lipids [17]. Thrombin is an essential component of the coagulation cascade and produced immediately in the brain after IVH. Our previous study has shown thrombin formation can contribute to the development of hydrocephalus after IVH [3]. In the present study, we found mid-aged LCR rats developed greater hydrocephalus than HCR rats. This result demonstrated a significant influence of exercise capacity in the initial impact of thrombin.
Hydrocephalus is often accompanied by periventricular white matter damage. Accumulating evidence implicates periventricular white matter damage as the major mechanism underlying poor behavioral outcomes [2]. In the present study, periventricular white matter damage was detected by MRI after intraventricular thrombin injection, and that damage was greater in LCR than HCR rats. The exact mechanisms of white matter damage induced by hydrocephalus are still unclear. It may be related to BBB damage, inflammation, and hypoxia-ischemia [3, 12].
That there is greater white matter damage in LCR compared with HCR rats was supported by the finding of greater HSP-32 expression, a stress marker, and more microglial activation in LCR rats. A question arises as to whether the changes in HSP-32 and microglial activation may participate in causing the greater injury. HSP-32, also called hemeoxygenase-1, is an enzyme involved in the breakdown of heme and the release of iron [4]. There is evidence in ICH that HSP-32 participates in brain injury [6, 8, 24]. Similarly, there is evidence that microglial activation may exacerbate ICH-induced brain injury [23] and that it is also associated with the development of white matter damage [9]. Thus, the changes in HSP-32 and microglia activation may participate in the greater periventricular white matter injury in LCR rats.
Conclusion
In summary, thrombin induced more severe hydrocephalus and periventricular white matter damage in the rats with low aerobic capacity. Differences in thrombin-induced injury may contribute to greater brain injury following cerebral hemorrhage in rats with low aerobic capacity.
Acknowledgments
This study was supported by grants NS-073959, NS079157, and NS-084049 from the National Institutes of Health (NIH) and 973 Program-2014CB541600. The LCR-HCR rat model system was funded by the Office of Research Infrastructure Programs/OD grant R24OD010950 and by grant R01DK099034 (to LGK and SLB) from the National Institutes of Health. SLB was also supported by National Institutes of Health grants R01DK077200 and R01GM104194. We acknowledge the expert care of the rat colony provided by Molly Kalahar and Lori Heckenkamp. Contact LGK (lgkoch@med.umich.edu) or SLB (brittons@umich.edu) for information on the LCR and HCR rats: these rat models are maintained as an international resource with support from the Department of Anesthesiology at the University of Michigan, Ann Arbor, Michigan.
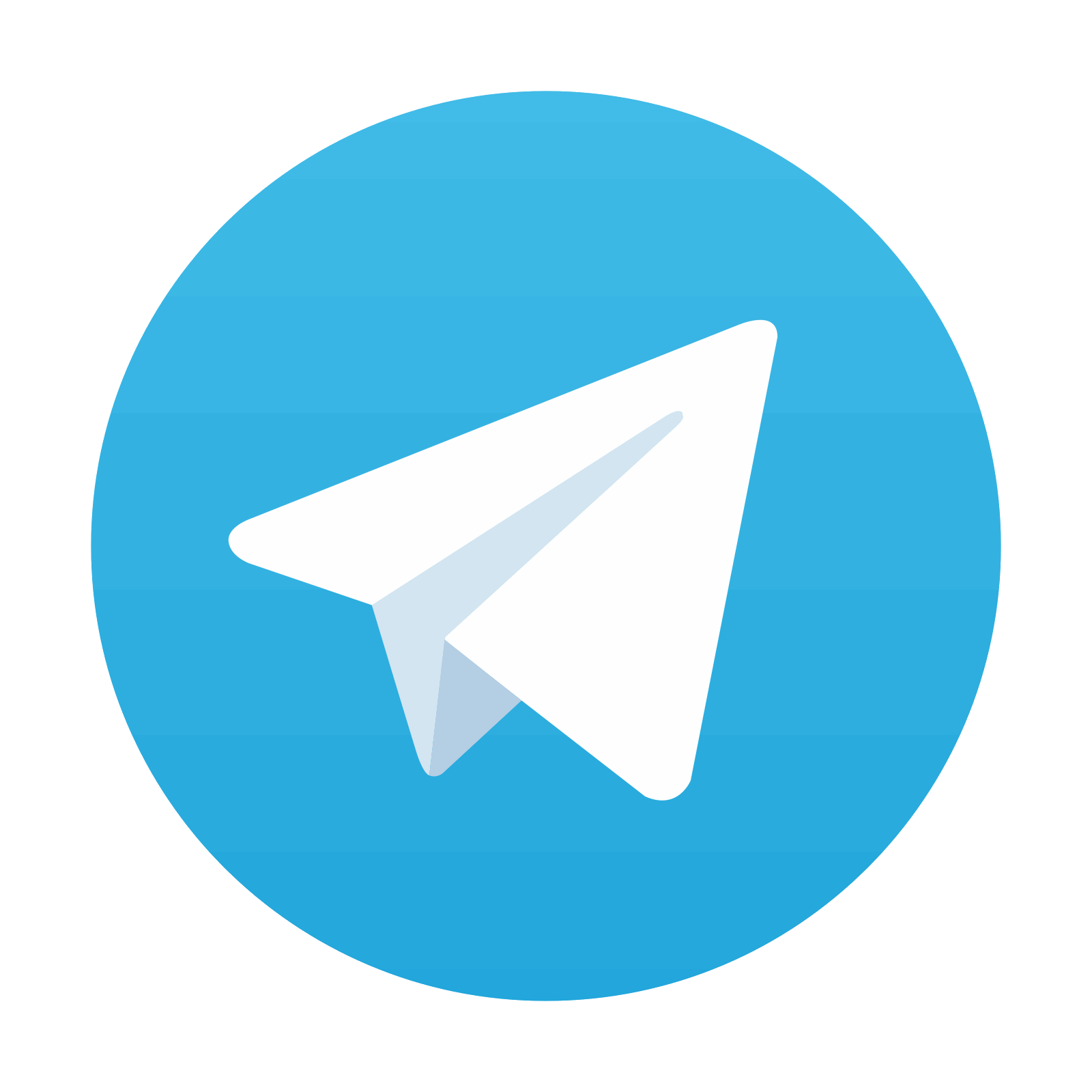
Stay updated, free articles. Join our Telegram channel

Full access? Get Clinical Tree
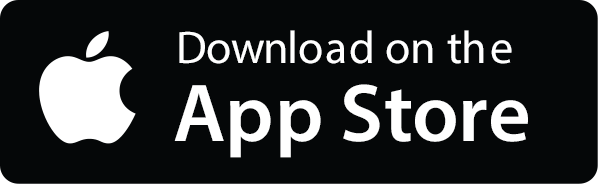
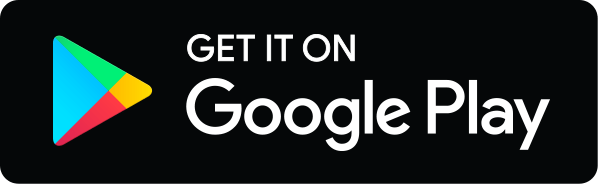